Arid zones are regions of low rainfall, high evaporation rate, and extreme temperatures (Mazuela 2013). In irrigated agricultural lands, water is an increasingly scarce resource due to drought, aquifers overexploitation, soil salinity, and contamination of water with heavy metals (Azpilcueta-Pérez et al. 2018). Furthermore, climate change is exacerbating these situations, with low water availability for agriculture production (Zárate & Miranda 2016).
In the last decades, the problem of soil salinization in agricultural areas by irrigation using water with a high salt content has become more acute (Kopittke et al. 2019). In arid and semi-arid zones, the salinization of agricultural soils due to intensive use of chemically contaminated water is caused by the accumulation of soluble salts that are deposited on the soil surface by capillary action and high evaporation rates. The problem of soil salinity in these regions is exacerbated by inappropriate water management practices, such as the inefficient application of irrigation water and inadequate soil drainage systems (İbrahimova et al. 2021).
Degradation of resources due to extreme natural and anthropic processes, and the need to maintain agri-food production make relevant the application of more sustainable production strategies (Balvanera et al. 2017). An alternative to this production approach is productive reconversion through the performance of profitable alternative crops (Mattas et al. 2015), which mitigate the environmental problems in marginal lands of arid zones.
Aloe vera is a crop of high acclimatization capacity to different environments and tolerance to extreme biotic and abiotic conditions. So, it is a productive alternative for marginal areas where traditional crops are no longer economically competitive (Davis et al. 2019). In addition, aloe leaves products, such as gel, juice as well as powdery are in high demand in the international market due to multiple benefits in the food, medical, and cosmetic industries (Sifuentes-Rodríguez et al. 2020).
More than 200 active chemical compounds have been reported in Aloe vera gel, such as vitamins, minerals, enzymes, simple and complex polysaccharides, phenolic compounds, and organic acids (Boudreau et al. 2013). Most of these chemical compounds are produced by secondary metabolism, which is activated when the plant is stressed (Isah 2019) .
Aloe vera responses to water deficit (Delatorre-Herrera et al. 2010, Silva et al. 2010, Espinoza-Garrido 2011, Hazrati et al. 2017, Minjares-Fuentes et al. 2017) and salinity (Moghbeli et al. 2012, Murillo-Amador et al. 2015, Xu et al. 2015) have been studied at length. Plant responses to water deficit and salinity are diverse and complex. Some of them are, for instance, cell membrane and cell wall adjustments, changes in the metabolic cycle, and cell division. In addition, the latter involves the synthesis of specific endogenous compounds of low molecular mass such as salicylic acid, jasmonic acid, ethylene, and abscisic acid (Fujita et al. 2006). Also, salt and water stresses cause an increase in reactive oxygen species (ROS) levels which may produce oxidative stress (Ahanger et al. 2017).
Some studies have shown that A. vera can tolerate moderate salt and water stresses. Murillo-Amador et al. (2015) found that A. vera maintains dry mass production under moderate concentrations of NaCl. Sifuentes-Rodríguez et al. (2020) found that A. vera is tolerant to certain levels of salinity by keeping biomass and gel production. Habibi (2018) reported that leaf thickness, biomass, and gel production of A. vera are not affected by moderate water stress, which may even improve plant growth. However, most studies have examined individual stress factors. The joint action of these stressors could trigger more complex response effects that need to be analyzed and, where appropriate, used for the knowledge and technology generated (Mittler 2006).
The joint occurrence of water deficit and salinity is frequent in agricultural regions where intensive water use, soil, and inputs are made, which presents a high risk in agri-food production (Mittler et al. 2001). Simultaneous study of both abiotic factors is imperative to deepen knowledge of the response to growth, development, and production of A. vera plants. This will make it feasible to design technological mitigation strategies in marginal agricultural lands. The objective of this study was to evaluate the effect of water deficit and soil salinity on some indicators of plant and leaf growth, physiology, and productivity of A. vera.
Materials and methods
Geographic location of the experimental area. The study was conducted at the University Regional Unit for Arid Zones of the Autonomous University of Chapingo in Bermejillo, Durango, Mexico. The region is located at lat. 23º 54'N and long. 103º 37'W and elevation 1,130 m. It has a dry climate with summer rains, an average annual rainfall of 239 mm, a winter rainfall percentage < 5 %, and a thermal oscillation from 7 to 17 ºC (Cano-Villegas et al. 2021).
Experimental design and treatments. The experiment was carried out under controlled shade-mesh conditions, during the spring and summer to 2020. A split-block experimental design with four replications was used. The large plots were the soil moisture contents at field capacity (FC) and permanent wilting point (PWP), corresponding to 20.7 and 12.3 % of soil water content, respectively. Soil moisture constants (FC and PWP) were determined using the membrane pot (Richards 1948) (Table 1). To maintain moisture content, irrigation was applied in ranges of 16 to 21 % and 10 to 15 %, respectively. The upper level of the first range corresponded to FC, while the lower level of the second range corresponded to a moisture content around PWP, to induce a water stress condition, due to the high drought tolerance of A. vera (Pedroza & Gómez 2006). Only two treatments of water content in the soil were carried out to study the response to extreme ranges (optimal and suboptimal) of water availability for the plant combined with five NaCl concentrations.
Table 1 Initial physical and chemical characteristics of the soil used in the study.
Physical characteristics* | Chemical characteristics* | ||
---|---|---|---|
Texture | sandy loam | Cation exchange capacity (ECC) | 18.2 |
Field capacity (%) | 20.7 | pH (paste) | 7.92 |
Permanent wilting point (%) |
12.3 |
Electrical conductivity (Paste, dS m-1) |
3.48 |
% Saturation | 30.57 | Extractable sodium (mg kg-1) | 297.3 |
Apparent density (g cm-3) | 1.56 | Sodium adsorption ratio (SAR) | 1.47 |
Exchangeable sodium percentage (ESP) | 6.1 | ||
Percentage of total carbonates (CaCO3) | 21.6 |
* Determinations carried out by the Centro Nacional de Investigación Disciplinaria en Relaciones Agua Suelo Planta Atmósfera (CENID-RASPA) of the Instituto Nacional de Investigaciones Forestales Agrícolas y Pecuarias (INIFAP), Gomez Palacio, Durango, Mexico.
The subplots varied in NaCl concentration: 0, 20, 40, 60, and 80 mM dissolved in the irrigation water to induce soil salinity. After four months of standardized irrigation at FC, irrigation was cut off until the soil moisture reached the lower limit of 16 or 10 %. Recovery irrigation brought each plot up to the corresponding upper limits of 21 and 15 %, respectively. Irrigation was made with a manual sprinkler and the water application to each treatment was based on daily monitoring of soil moisture using a digital sensor with a real-time readout (Soil Moisture Meter Mod. MO750, manufacturer Extech, Taiwan, China).
Six-month-old A. vera plants of sizes from 25 to 30 cm were used, which were obtained in the form of suckers from the mother plant. The experimental unit was one plant per pot containing 10 kg of soil. The soil was collected in the study region and according to the physical and chemical analysis is a soil with a slightly alkaline pH and an electrical conductivity (EC) of 3.48 dS m -1 equivalent to a non-saline soil (Mahdy 2011) , among other features (Table 1).
Variables of plant growth and leaves. Plant height and leaf length were measured with a flexometer, the first variable was measured from ground level to the end of the largest leaf, and the second variable was measured at the outermost leaf of the bud; while the width and thickness of the leaf were measured with a vernier (Truper, model 14388, China). All variables were measured at intervals of 22 days during 110 days after starting the treatments (DAST).
Physiological attributes. Plant water status was determined monthly, in terms of relative water content (RWC) (Yamasaki & Dillenburg 1999). At the end of the experiment, total solids (TS), which are defined as the organic and inorganic matter that remains as a residue after evaporation and dried at 103 - 105 °C to constant mass, were determined (Calzada & Pedroza 2005). This variable was determined by weighting five g gel with an analytical balance (model SHIMADZU AY220) and then each sample was placed in a porcelain crucible to achieve the constant mass. These variables were determined at 14, 42, and 80 DAST. The dates of sampling were based on finding possible differences in response due to a change in the sunshine and seasonal temperature during the experimental period.
Productivity and performance attributes. The fresh biomass (FB) production, including the aerial part and roots, was determined using a gravimetric balance, while the percentage of gel was estimated using the following equation:
For this determination, the leaves of the plant were cut from their base and filleted with a knife, cutting the tip and the lateral parts to subsequently detach the cuticle from the beam and the underside with the use of a spatula and obtain the crystalline gel separated from the cuticle.
The harvest index (HI) was defined as the ratio of the weight of gel and the weight of fresh biomass, and was calculated following the equation:
Data Analysis. The information was analyzed with a split-block experimental model with a factorial arrangement in treatments with the GLM procedure of the statistical analysis system (SAS Institute v. 9.0, Cary, NC, USA). In the separation of means, the minimum significant difference of Tukey's test was used (P ≤ 0.05). The regression models for gel production and harvest index versus salinity levels were fitted in Microsoft Excel.
Results
Plant growth and leaves. Growth of the aloe plant and leaves was slightly affected by the soil moisture content, mainly in the width and thickness of the leaf and, less consistently, in the height of the plant, without any measurable effect on leaf length. The effect of soil moisture content and levels of salinity on plant height was not consistent, with a trend to decrease at 60 mM NaCl and above at either soil moisture content. Salt stress affected leaf width only at the highest NaCl concentrations (80 mM) in plants under FC. However, there were significant effects at 60 mM NaCl in plants under PWP. Control plants had leaf width values of 3.1 cm and 3.0 cm for FC and PWP, respectively. Leaf thickness was more sensitive to salinity, a decrease in this trait was observed since 40 mM NaCl in plants under FC and PWP. The higher leaf thickness was shown by plants under 0 mM NaCl with values of 1.26 cm and 1.02 cm under FC and PWP, respectively (Table 2).
Table 2 Effect of soil moisture content and salt concentrations on different growth variables of the aloe (Aloe vera L.) plant and leaf.
Salinity (mM) | FC | PWP | FC | PWP | FC | PWP | FC | PWP |
---|---|---|---|---|---|---|---|---|
plant height (cm) |
leaf length (cm) |
leaf width (cm) |
leaf thickness (cm) |
|||||
0 (Control) | 46.2 ± 3.10 abc* | 44.2 ± 3.99 b | 31.3 ± 8.80 a | 30.1 ± 7.55 a | 3.1 ± 0.70 a | 3.0 ± 0.41 a | 1.26 ± 0.08 a | 1.02 ± 0.12 a |
20 | 46.8 ±2.99 ab | 46.5 ± 2.90 a | 29.0 ± 8.36 a | 29.8 ± 7.00 a | 2.8 ± 0.55 ab | 2.9 ± 0.48 ab | 1.15 ± 0.16 ab | 0.91 ± 0.11 a |
40 | 45.3 ±2.60 bc | 44.6 ± 1.82 ab | 29.8 ± 7.64 a | 27.8 ± 7.45 a | 2.9 ± 0.62 ab | 2.5 ± 0.39 b | 1.13 ± 0.14 bc | 0.87 ± 0.17 b |
60 | 48.4 ± 2.94 a | 44.0 ± 2.25 b | 30.4 ± 7.85 a | 28.5 ± 7.14 a | 2.8 ± 0.54 ab | 2.9 ± 0.66 a | 1.01 ± 0.21 c | 0.91 ± 0.19 a |
80 | 44.2 ± 1.93 c | 44.1 ± 0.19 b | 25.8 ± 6.94 a | 29.0 ± 6.59 a | 2.4 ± 0.40 b | 2.7 ± 0.41ab | 0.85 ± 0.11 c | 0.85 ± 0.17 b |
*Mean values (± standard deviation) with the same letters within columns, are statistically equal according to the minimum significant difference of Tukey's test (P ≤ 0.05). FC: field capacity (20.7 %); and PWP: permanent wilting point (12.3 %).
Relative water content and total solids. The relative water content (RWC), an indicator of the water status of the leaf tissue, was not affected by either soil moisture or salinity (P > 0.05) (Table 3). Total solids (TS) showed a significant effect (P ≤ 0.05), which depended on soil moisture content, salinity level, and monitoring sampling. There was a non-consistent response at the first two monitoring dates, corresponding to 14 and 42 DAST at both soil moisture contents. There was a trend toward a significantly lower concentration of TS in the control, compared to salinity treatments from 20 to 80 mM, mainly under PWP conditions, where the greatest concentrations of TS were obtained at 60 and 80 mM. The TS values for 80 mM NaCl were 5.2 and 5.3 % compared to 3.1 and 3.8 % for the control, respectively. There was a consistent treatment effect of significantly higher values (P ≤ 0 .05) at 80 DAST at both soil moisture contents with a TS concentration of 8.9 % for plants under PWP and 40 mM NaCl and 8.8 % plants under FC plus 80 mM NaCl, while control plants showed 3.8 % at both soil moisture contents (Table 4).
Table 3 Effect of salinity and two soil moisture contents on different monitoring dates on the relative water content (RWC) in Aloe vera leaves.
Salinity (mM) | Relative water content (%) | |||||
---|---|---|---|---|---|---|
14 DAST | 42 DAST | 80 DAST | ||||
FC | PWP | FC | PWP | FC | PWP | |
0 | 94.3 ± 0.46 a* | 94.6 ± 0.38 a | 96.4 ± 2.17 a | 95.8 ± 0.15 a | 92.7 ± 0.90 a | 92.8 ± 0.25 a |
20 | 95.6 ± 0.10 a | 94.8 ± 0.53 a | 94.1 ± 0.27 a | 93.3 ± 1.45 a | 90.3 ± 0.75 a | 92.3 ± 2.61 a |
40 | 97.0 ± 0.14 a | 96.7 ± 2.02 a | 93.5 ± 0.79 a | 93.5 ± 0.79 a | 89.4 ± 0.001 a | 91.3 ± 1.49 a |
60 | 93.6 ± 0.22 a | 94.8 ± 1.26 a | 94.2 ± 0.003 a | 91.8 ± 0.11 a | 88.5 ± 1.28 a | 86.6 ± 1.81 a |
80 | 95.6 ± 0.55 a | 92.0 ± 0.71 a | 92.9 ± 1.64 a | 91.9 ± 0.10 a | 89.1 ± 1.10 a | 89.1 ± 0.71 a |
*Mean values (± standard deviation) with the same letters within columns, are statistically equal according to the minimum significant difference of Tukey's test (P ≤ 0.05). DAST: days after starting treatments; FC: field capacity (20.7%); and PWP: permanent wilting point (12.3%).
Table 4 Effect of salinity at two soil moisture contents on different monitoring dates on the concentration of total solids (TS) in Aloe vera leaf.
Salinity (mM) | Total solids (%) | |||||
---|---|---|---|---|---|---|
14 DAST | 42 DAST | 80 DAST | ||||
FC | PWP | FC | PWP | FC | PWP | |
0 | 2.7 ±0.33 c* | 3.1 ± 0.27 ab | 4.9 ± 1.53 a | 3.8 ± 0.11 c | 3.8 ± 0.63 b | 3.8 ± 0.18 b |
20 | 3.8 ± 0.07 b | 2.8 ± 0.37 ab | 4.4 ± 0.19 a | 5.9 ± 1.02 a | 7.3 ± 0.53 a | 6.8 ± 1.85 ab |
40 | 4.6 ± 0.1 a | 2.6 ± 1.42 b | 4.1 ± 0.55 a | 4.5 ± 0.56 bc | 8.5 ± 0.0008 a | 8.9 ± 1.05 a |
60 | 4.6 ± 0.15 a | 4.8 ± 0.89 ab | 5.5 ± 0.002 a | 5.7 ± 0.08 ab | 8.1 ± 0.90 a | 7.5 ± 1.28 a |
80 | 3.7 ± 0.39b | 5.2 ± 0.50 a | 5.5 ± 1.16 a | 5.3 ± 0.07 ab | 8.8 ± 0.78 a | 7.8 ± 0.50 a |
*Mean values (± standard deviation) with the same letters within columns are statistically equal according to the minimum significant difference of Tukey's test (P ≤ 0.05). DAST: days after starting treatments; FC: field capacity (20.7 %); and PWP: permanent wilting point (12.3 %).
Fresh biomass (FB), gel percentage (GP), and harvest index (HI). FB was affected by both water deficit and salinity, but to a lesser extent in FC than in PWP. In both irrigation treatments, the negative impact on productivity was above 20 mM salinity (40, 60, and 80 mM). The latter effect was more severe in PWP at any salinity level (Figure 1).
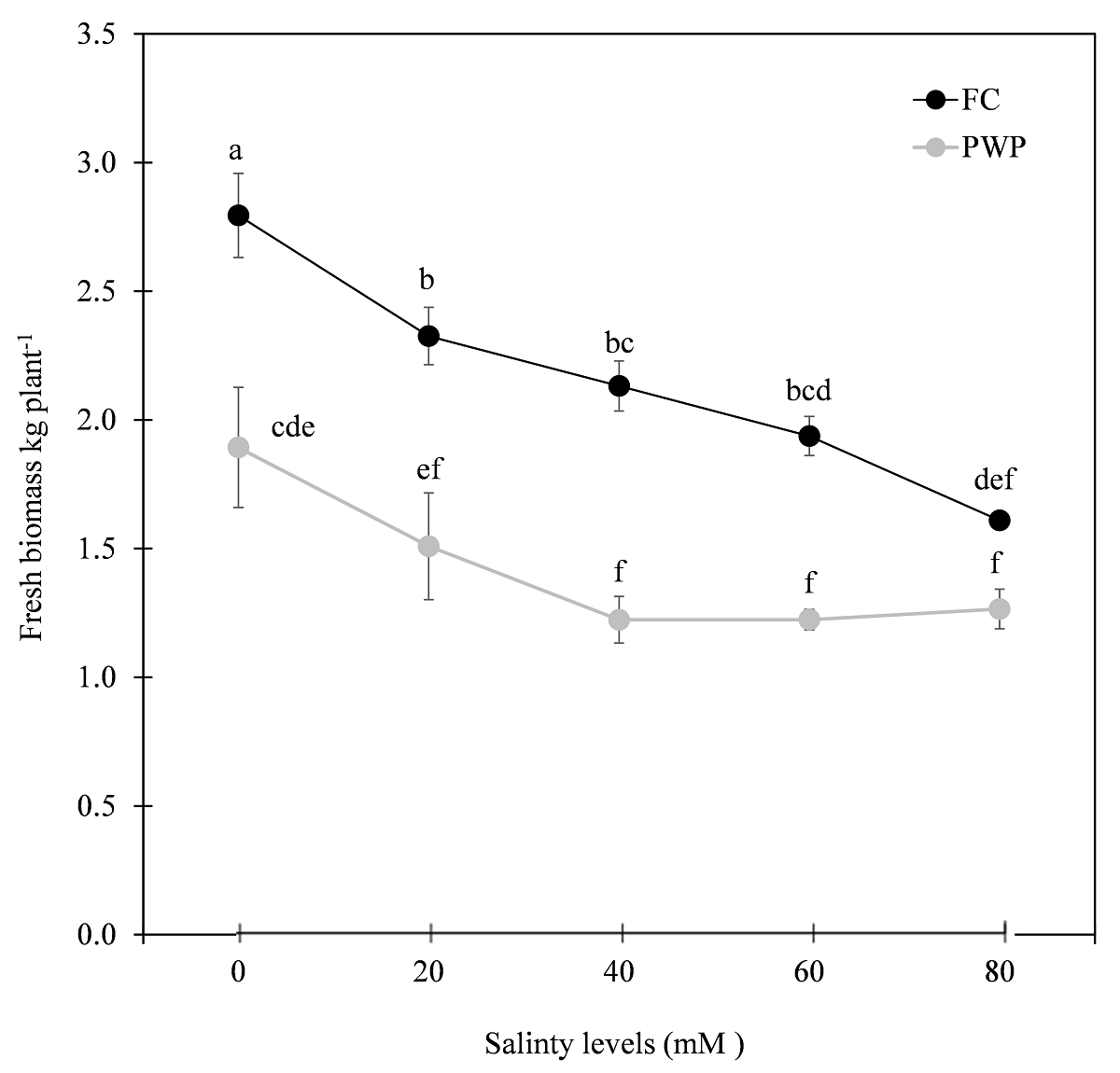
Figure 1 Effect of treatment on fresh biomass production in Aloe vera grown with two soil moisture contents and different salinity levels. Values with the same letters on each line are statistically equal according to the minimum significant difference of Tukey's test (P ≤ 0.05). FC: field capacity (20.7 %); and PWP: permanent wilting point (12.3 %).
Percentage of gel showed a negative relationship with salinity, the effect was slightly greater in PWP (β1 = -4.41; R2 = 0.98) than in FC (β1 = -4.63; R2 = 0.95), respectively (Figure 2). The maximum gel production (73.1 %) was found in the salinity control at FC. The plants under PWP and 0 NaCl produced 63 % gel . The harvest index (HI) behaved similar to gel production. The values for FC and PWP were β1 = -1.40 and R2 = 0.75 and β1 = -1.50 and R2 = 0.71, respectively (Figure 3).

Figure 2 Aloe leaf gel production in Aloe vera grown with two moisture contents and different salinity levels. FC: field capacity (20.7 %); and PWP: permanent wilting point (12.3 %).
Discussion
Plant growth and leaves. The minimum soil moisture content in this study was 10 %, corresponding to moisture below the PWP (12.3 %), but this was not enough to induce a stress condition within the A. vera plant tissues and reduce growth. Tolerance to water stress in A. vera is widely recognized and is associated with tissue succulence and the photosynthetic pathway of crassulacean acid metabolism (CAM) (Pedroza & Gómez 2006).
Salinity affected the width and thickness of the leaf, with a tendency to reduce both at 40 mM salinity on average. This suggests a moderate tolerance to this stress factor at both soil moisture contents, without drastically reducing leaf width and thickness, which are directly related to fresh biomass production. This confirms the high tolerance of A. vera to water stress conditions in the growth of the plant and leaves, but there was a negative impact of salinity, in the thickness and width of the leaves and, inconsistently, in plant height. This is supported by Asghari & Ahmadvand (2018), who found that salinity did not affect A. vera plant height consistently; however, other authors reported that salinity does inhibit plant growth (Aziz et al. 2008, Said-Al & Omer 2011).
This negative effect of salinity is recognized as a product of stress, which triggers physiological and biochemical changes in plant metabolism that determine their survival and productivity (Samadi et al. 2019). Salinity damage is associated with a toxic effect of ions such as sodium (Na+), chlorides (Cl-), and sulfates (SO4-), although sodium is an essential micronutrient in plants with C4 and CAM photosynthetic pathways; hence, its intermediate-range of tolerance to salinity (Lamz & Gonzalez 2013). Another effect of salinity in irrigated soils with saline water is a nutritional imbalance in the plant from reduced assimilation of other mineral nutrients, such as K, Ca, and Mn (Karimi et al. 2009). To soil salinity must be added poor management, which reduces water permeability and soil aeration and compacts the soil structure (Singh et al. 2012).
The width and thickness of the leaf suggest that salinity has a negative effect, somewhat independent of the soil moisture content, due to the parallel but differentiated response, with an inhibition effect on both variables from 20 mM, but a greater impact on leaf thickness in FC and 40 mM salinity. This is important since these variables are directly related to gel production, the main commercial product in the international market (Sanchez 2016). The moderating effect of salinity on these morphometric variables may be related to an acclimatization process due to the gradual accumulation of NaCl in the soil. Therefore, a moderate initial exposition to a stress factor can induce protection mechanisms that allow plants to tolerate a subsequent drastic stress factor (Janda et al. 2016).
Plants previously treated with a low concentration of NaCl, turned out to be effective in inducing greater tolerance to salinity, throughout an acquired tolerance process (Pandolfi et al. 2016). Plant height and leaf length were not affected by either soil moisture content or salinity, suggesting that the total plant water potential remained high enough to allow cell division and growth of aloe plants (Rodríguez et al. 2019).
Relative water content (RWC) and total solids (TS). The RWC is the most commonly measured indicator of plant water status and is directly related to the water potential since its components, turgor potential and osmotic potential, are a function of the volume of water in the protoplasm (Argentel et al. 2006). The null effect of water deficit and salinity in the RWC shows high aloe plants' tolerance to these stress factors, as levels applied in this study were insufficient to modify tissue water status, which is determinant to the survival, the growth, and productivity of plants (Bacarrillo-López et al. 2021). These results are supported by Moghbeli et al. (2012) and Murillo-Amador et al. (2015), who found no effect on RWC under saline stress conditions. Although there were no significant differences in this variable under the soil moisture and NaCl conditions evaluated in this study, some authors reported that average RWC values > 90 % are acceptable to maintain the growth and productivity of aloe plants (Minjares-Fuentes et al. 2017).
TS increased in a more defined way after 80 DAST, somewhat independent of the soil moisture content, but strongly, starting at 20 mM salinity. This is of great relevance for gel quality, which requires a higher concentration of Total solids that leads to increased high and low-molecular-mass polysaccharides, and other products, which are greatly valued in the international market according to the International Aloe Science Council (IASC 2008). These results matched with other studies where an increase of TS concentration in A. vera gel was obtained by an increase in soil salinity. According to Sifuentes-Rodríguez et al. (2020), the concentration of sugars and percentage of total soluble solids was the highest as so did the soil salinity, even when aloe leaf production was reduced.
Fresh biomass (FB), gel percentage (GP), and harvest index (HI). The variable most affected by both water deficit and salinity was the FB, mainly in PWP at high salinity levels; while at FC, the plants maintained adequate productivity of FB. This suggests that, although there was a moderate effect on leaf width and thickness at low or no salinity, regardless of the moisture ranges evaluated in this study, the overall FB is reduced. This could be probably due to less water availability for metabolic activity and its final products and great concentration of solutes (osmotic pressure), where water in the plant’s tissues plays a role more for survival than physiological availability for productivity (Sifuentes-Rodríguez et al. 2020). This could also be associated with the effect of osmotic adjustment in extreme environments and reduction in the cytosolic and vacuolar volumes of the cells (Flower et al. 2015). These results disagree with those of the Delatorre-Herrera et al. (2010) report, who indicates that, at a moderate water deficit, the production of FB increases. However, the results of our study were consistent with those reported by Choudhury et al. (2017), who found that a combination of two or more abiotic stresses produces greater yield loss than single stress.
The responses of gel reduction were directly related to FB production, most notably when going from FC to PWP, but with similar rates of decrease in both soil water treatments as salinity increased. The stress-induced by the combination of salinity and water deficit increased the severity of productivity reduction in Aloe vera. Rodríguez-Dorantes et al. (2003) mentioned that the first and most sensitive response to water deficit is a decrease in turgor and, consequently, the FB and gel production. Silva et al. (2013) found that plants usually react to extreme water stress by favoring the photosynthesis maintenance and reducing gel yield. Plants in general trend to reduce growth and productivity under conditions of biotic and/or abiotic stress (Cramer et al. 2011).
The HI of a crop determines yield under drought conditions (Deguchi et al. 2010). The decreased HI in this study was lesser than that of gel production and FB, which confirms the tolerance to stress that Aloe vera has compared to other crops that are more drastically affected by water deficit (Schafleitner et al. 2007, Ruttanaprasert et al. 2016).
Finally, water deficit and salinity, mainly the latter, moderately affected the width and thickness of the Aloe vera leaf, which reduced FB, gel content, and HI, but increased gel quality through increased TS, an integral quality attribute demanded in the international market since reach high values of organic and inorganic matters as minerals as well as diverse types of polysaccharides of high and low molecular weight, among other types of residues. Aloe vera showed a high tolerance to water stress and moderate tolerance to salinity in the width and thickness of leaves, FB, and gel production. This allows focusing on managing low soil moisture content close to PWP, without negatively affecting FB, while using irrigation water with moderate salinity to maintain a balance between quantity and quality in the gel production of A. vera.