In recent years, the global temperature has been rising and studies have shown that high temperature stress has adverse effects on plant growth and development. Wheat (Triticum aestivum L.), usually grown in temperate regions, is the main food crop in the northern regions of China. Its production can be reduced by 20-30 % during elevated temperature in developing countries, which account for about 66 % of worldwide wheat production (Khan et al. 2013).
Photosystem II (PSII) can be easily damaged during heat stress, resulting in reduced activity under these conditions (Lin et al. 2015, Das et al. 2016, Doğru 2021). When subjected to heat stress, D1 protein is often damaged, resulting in a significant reduction in PSII activity (Morales et al. 2003). One study by Havaux (1996) showed that under heat stress, electron transfer on the donor side of PSII was inhibited, but not on the receptor side. However, another study reported that heat stress causes damage to the PSII donor side and an over-reduced receptor side (Zhang & Liu 2016). These conflicting results indicate that the specific response mechanism of PSII to heat stress is still not completely clear.
When subjected to environmental stresses, plants can accumulate a large amount of endogenous trehalose, which acts a significant role in improving stress tolerance (Elbein et al. 2003). Rice naturally accumulates 3-10 times higher trehalose levels during stress, and exogenously-supplied trehalose enhances its stress tolerance, resulting in relatively normal growth of plants under environmental conditions that are not conducive to plant growth, for instance, drought, high temperature and high salt (Ge et al. 2008, Ali et al. 2010, Luo et al. 2010, Yang et al. 2014). Our previous research demonstrated that trehalose pretreatment could stabilize the ultrastructure of chloroplast, protect some polypeptides on the thylakoid membrane, and improve the photosynthetic capacity of wheat seedings under high temperature (Luo et al. 2010). Doehlemann et al. (2006) have hypothesized that trehalose protects plants during heat stress, allowing them to maintain a relatively normal growth state.
Up to now, there are few studies on the impact of trehalose on PSII secondary structure and function in plants under heat stress. Additionally, it has not been determined whether trehalose could improve heat tolerance by protecting PSII. In this study, winter wheat seedlings with trehalose pretreatment were incubated at 40 and 25 °C. Then, their photosynthetic parameters, including the content of D1 protein, PSII protein secondary structures, chlorophyll fluorescence parameters, chlorophyll a fluorescence induction curve and photosynthetic electron transport rate were measured. These data improve the current understanding of the influences of trehalose on the structure and function of PSII in wheat seedlings under heat stress and the relationship between trehalose and the thermal stability of wheat seedlings. The goal of this study was to build on the studies of photosynthesis and abiotic stress tolerance of trehalose under adverse conditions and provide a theoretical basis for the response of heat-sensitive crops to high temperature stress.
Materials and methods
Culture of plants. The culture method was based on our previous study (Luo et al. 2010). Wheat seedlings (Triticum aestivum L.) were cultured with half-strength Hoagland solution until the second leaf was fully unfolded (about 15 d) and the climate chamber was set at 25 ℃, 70 % relative humidity and 16 h photoperiod (100 μmol·quanta m-2·s-1). Afterwards, the seedlings were divided into two groups, with one group treated with half-strength Hoagland solution containing 1.5 mM trehalose for 3 d and the other treated with half-strength Hoagland solution without trehalose as the control. Control and treated seedlings were then treated at 40 ± 0.5 ℃ for another 24 h. Then, they were grown at room temperature (22 ºC) again for collecting the second leaves of the seedlings for the following experiment.
Incubation of detached leaves with streptomycin. The synthesis of D1 was blocked by 3 mM streptomycin (SM). The separated leaves, together with their petioles, are immersed in a 3 mM aqueous solution of SM for 4 h, and then put in a climate chamber with a light intensity of 100 µmol·quanta m-2·s-1 and a temperature of 40 ℃ for 24 h, and finally, the temperature was adjusted to 25 ℃ for another 24 h to obtain the recovery data. These treated leaves were used for determination of fast chlorophyll fluorescence induction curve western blot analysis.
Determination of chlorophyll fluorescence parameters. As previously reported (Su et al. 2014), a pulse-modulated fluorimeter (WALZ, Dual-PAM 100, Germany) was used to measure the chlorophyll fluorescence parameters. Before measurement, the second leaf of each wheat seedling was placed in darkness for 20 min. The actinic light intensity of pulse-modulated fluorimeter was set to 400 μmol·quanta m-2·s-1, and the saturated flash intensity was set to 8,000 μmol·quanta m-2·s-1. The maximum photochemical efficiency of PSII (Fv/Fm) was expressed as Fv/Fm = (Fm - Fo)/Fm, where Fo is the initial fluorescence, Fm is the maximum fluorescence and Fv is the variable fluorescence. The actual photochemical efficiency of PSII (Y(II)) was expressed as: Y(II) = (Fm’- Ft)/Fm’, in which Ft is the steady-state fluorescence andFm’ is the maximum steady-state fluorescence. The quantum yield of regulated energy dissipation of PSII was expressed as: Y(NPQ) = (Fm - Fm’)/Fm’. The quantum yield of non-regulated energy dissipation of PSII was calculated as: Y(NO) = Ft/Fm. The electron transport rate of PSII was calculated as: ETR(II) = Y(II) × photosynthetically active radiation × 0.5 × 0.84, and the photochemical quenching coefficient (qP) was calculated as: qP= (Fm’ - Ft)/(Fm’ - Fo).
Measurement of fast chlorophyll fluorescence induction kinetics. Referring to the experiment of Strasser et al. (2000), we used a Plant Efficiency Analyzer (Pocket PEA, Hansatech, UK) to obtain Fast chlorophyll fluorescence induction kinetics (OJIP), so as to estimate the overall vitality of wheat and analyze the different partial processes of photosynthetic apparatus. The minimum fluorescence intensities were measured at 50 μs (O phase) as well as the fluorescence intensity at 2 ms (J phase), 30 ms (I phase) and 300 ms (P phase) (Strasser et al. 2000, Zhu et al. 2021).
Electron transport activity measurements. Referring to the experiment of Zhang & Liu (2016), we measured electron transport activities with a Clark-type O2 electrode (Hansatech, Chlorolab2, UK). With 0.5 mM diphenylcarbazide (DPC) as electron donor and 0.9 mM p-benzoquinone as electron acceptor, the activity of PSII was measured by O2 evolution. After adding 0.1mM methyl viologen (MV), the electron transport activity of the whole chain was determined by the absorption of O2. When no water-splitting complex was present, total electron transport activity was determined by adding 0.5 mM DPC as an electron donor in the experimental mixture. In the presence of 5 mM ascorbate, 0.1 mM 2, 6 - dichlorophenol indophenol (DCPIP), 1 mM potassium cyanide, 10 μM 3 - (3, 4-dichlorophenyl)-1,1-dimethylurea, 5 mM NaN3 and 0.1 mM MV, the activity of PSI was measured as O2 uptake.
Preparation of thylakoid membranes. The preparation of thylakoid membranes was according to the experimental method of Rintamaki et al. (1996). The leaves were milled and mixed with cool isolation buffer to homogenate, then centrifuged at 1,500 g for 4 min at 4 ℃. After that, the resulting pellets were rinsed with 10 mM HEPES - NaOH, pH 7.5, 5 mM sucrose, 5 mM MgCl2 and 10 mM NaF, then centrifuged for 3 min at 3,000 g. Then the thylakoid pellets with a small amount of storage buffer were resuspended and stored at -80 ℃ for later use.
Sodium dodecyl sulfate-polyacrylamide gel electrophoresis (SDS - PAGE) and western blot analysis. According to the experiment of Su et al. (2014), the thylakoid membrane fraction (5 μg of protein) was decomposed on 15 % SDS-PAGE and then immune-detection was performed. Chloroplast protein polyclonal antibody (Anti-psbA antibdy, Abcam) was used as the primary antibody, and alkaline phosphatase enzyme-labeled antibody IgG (H + L) (Donkey Anti-Rabbit IgG H & L (Alexa Fluor® 680), Abcam) was used as the secondary antibody. The membrane was tested by and infrared laser imaging system (Odyssey CLx, USA), and the Image J software was used to obtain relative content of D1 protein.
Determination of PSII protein secondary structure. Referring to a previous report (Xu et al. 1995), the bioactive PSII membranes particles were extracted and then stored in SMN buffer (10 mM NaCl, 0.4 M sucrose, 40 mM MeS-NaOH, pH 6.5). The concentration of chlorophyll sample was adjusted to 2 mg·ml-1, and PSII membrane particles were stored in liquid nitrogen for subsequent experiments. A Fourier transform infrared spectrometer (Type 6700, Thermo Electron Corporation) was used to measure the spectrum of membrane particle samples. The relative amount of the main protein secondary structures was calculated by Lorentz transformation in Origin 8.0 software.
Statistical analysis. Each measurement was repeated 3-5 times, with mean ± standard deviation as the result. The results were statistically analyzed by IBM SPSS statistics 20.0 software (IBM, New York, USA), followed by one-way analysis of variance of all the data to verify the variability of the data and the validity of the results. The results are plotted using Excel 2013, taking a significance level 0.05 (P < 0.05).
Results
Effects of exogenous trehalose on fast chlorophyll fluorescence kinetics characteristics in wheat seedlings under different treatments. When subjected to heat stress, electron transport flux per sample cross section (ETo/CSo) and electron transport flux per reaction center (ETo/RC) were observed to decrease observably, and parameters including Fv/Fm, Fv/Fo and PIabs were also significantly reduced (Figure 1). However, parameters including trapped energy flux per reaction center (TRo/RC), absorption flux per reaction center (ABS/RC), absorption flux per cross section (ABS/CSo), dissipated energy flux per reaction center (DIo/RC) and dissipated energy flux per cross section (DIo/CSo) increased. These observations indicated that the excitation energy was significantly dissipated, the energy used for electron transfer was decreased and the photosynthetic capacity of PSII was inhibited. Compared with control plants, higher Fv/Fm, Fv/Fo and PIabs in trehalose pretreated seedlings were seen (Figure 1).

Figure 1 Effects of exogenous trehalose on JIP-test parameters radar chart in wheat leaves under different treatments. Four groups of seedlings were cultured with half-strength Hoagland solution, and the other four groups were cultured with half-strength Hoagland solution containing 1.5 mM trehalose for 3 d, then treated as follows: cultured at 25 ℃ (H0 CK or H0 TRE); exposed to 40 ℃ (± 0.5 ºC) for 24 h (H24 CK or H24 TRE); exposed to 40 ℃ (± 0.5 ºC) for 24 h and then recovery at room temperature for 24 h (R24 CK or R24 TRE) or 48 h (R48 CK or R48 TRE). All parameters were measured by Plant Efficiency Analyzer (Pocket PEA, Hansatech, UK). Fv/Fm: the maximum photochemical efficiency of PSII; Fv/Fo: potential photochemical activity of PSⅡ; ABS/RC: absorption flux per reaction center; DIo/RC: dissipated energy flux per reaction center; TRo/RC: trapped energy flux per reaction center; ETo/RC: electron transport flux per reaction center; ABS/CSo: absorption flux per cross section; DIo/CSo: dissipated energy flux per cross section; TRo/CSo: light energy capture per excited state area; ETo/CSo: electron transport flux per sample cross section; PIabs: photosynthetic performance index, a comprehensive reflection of the maximum photochemical efficiency of PSⅡ and the number of active reaction centers.
A typical OJIP curve was observed in control at room temperature (Figure 2A). While under heat stress conditions, the curve tended to be a smooth straight line, with Fo rising then decreasing, and the inflection point between J and I almost disappearing. The peak amplitude of J, I, P and their differences in fluorescence values were also reduced (Figure 2A). J, I and P peak values gradually recovered and the I peak reappeared, when the seedlings recovered from heat stress for 24 and 48 h (Figure 2A). J, I and P peaks in trehalose-pretreatment groups were more stable and significantly higher than control plants.

Figure 2 Effects of exogenous trehalose on chlorophyll fluorescence induction curve of control (A), 3 mM streptomycin (SM) treatment (B) in wheat leaves under different treatments. Two groups of seedlings were cultured with half-strength Hoagland solution, and the other two groups were cultured with half-strength Hoagland solution containing 1.5 mM trehalose for 3 d, then then treated as follows: cultured at 25 ℃ (H0 CK or H0 TRE); exposed to 40 ℃ (± 0.5 ºC) for 24 h (H24 CK or H24 TRE). Then, incubate the leaves with 3 mM SM which was used to block the synthesis of D1. Fast chlorophyll fluorescence induction kinetics (OJIP) was measured (room temperature, light intensity of 120 μmol· m-2 ·s-1) by Plant Efficiency Analyzer (Pocket PEA, Hansatech, UK). Different letters show significant differences (P < 0.05). 1 - H0 TRE; 2 - H0 CK; 3 - H24 TRE; 4 - H24 CK
Incubation of leaves with SM resulted in greater differences between trehalose pretreated and control plants, which brought about greater initial fluorescence slope and higher J, I and P peaks in trehalose pretreated seedlings than control plants at heat stress temperature and room temperature (recovery condition), with the exception of R24 (Figure 2B). After recovery of 24 h from heat stress in trehalose-pretreatment groups, the inflection point between J and P almost disappeared (Figure 2B).
Effects of exogenous trehalose on chlorophyll fluorescence parameters in wheat seedlings under different treatments. Chlorophyll fluorescence parameters are commonly used to examine changes in PSII activity under stress conditions. Y(NO) (Figure 3E) increased, while Fv/Fm (Figure 3A), Y(II) (Figure 3B), ETR(II) (Figure 3C) , Y(NPQ) (Figure 3D) and qP (Figure 3F) obviously decreased under heat stress. When subjected to heat stress, although there was no significance in Y(NPQ) between trehalose pretreated seedlings and control, higher Fv/Fm (Figure 3A), Y(II) (Figure 3B), ETR(II) (Figure 3C), qP (Figure 3F) and lower Y(O) (Figure 3E) were observed in the trehalose pretreatment group, indicating that trehalose pretreatment reduced the non-photochemical quenching level and increased the efficiency of photochemical. No differences in chlorophyll fluorescence parameters between 24 h recovery (R24) and 48 recovery (R48) were seen, so 24 h was selected as the recovery time.
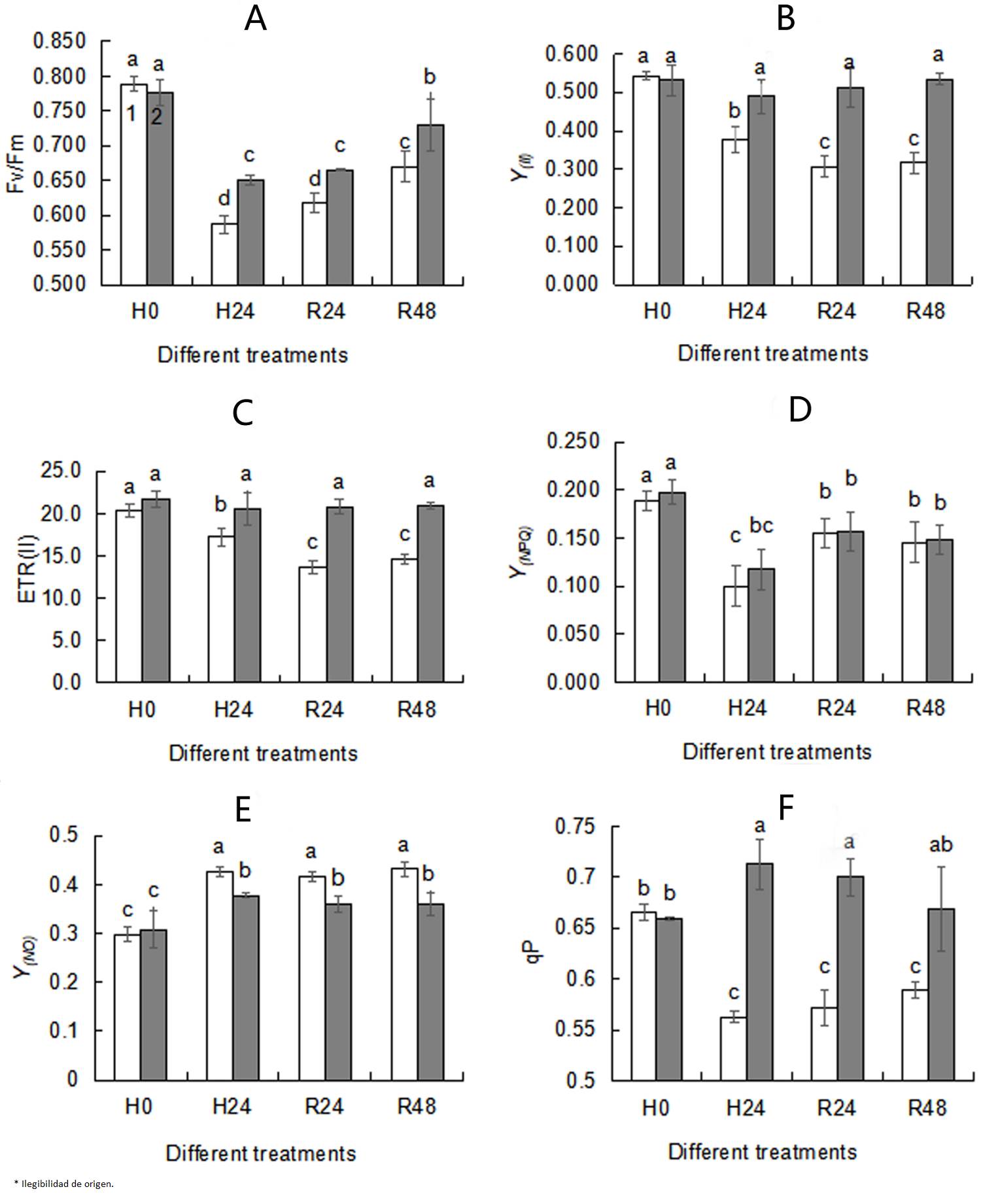
Figure 3 Effects of exogenous trehalose on chlorophyll fluorescence parameters Fv/Fm(A), Y(II)(B), ETR(II)(C), Y(NPQ)(D), Y(NO)(E), qP(F) in wheat leaves under different treatments. After seedlings pretreated with 1.5 mM trehalose for 3 d (TRE), with half-strength Hoagland solution as control (CK), then they were grown under the following conditions respectively: a temperature of 25 ºC (H0); high temperature (40 ºC ± 0.5 ºC) treatment for 24 h (H24). After that, they recovered at 22 ºC for 24 h (R24) and 48 h (R48) . Chlorophyll fluorescence parameters were measured using a pulse-modulated fluorometer (WALZ, Dual-PAM 100, Germany). The second leaf of each wheat seedling was kept in the dark for 20 min before measurement. Different letters show significant differences (P< 0.05). Fv/Fm: the maximum photochemical efficiency of PSII; Y (II) : the actual photochemical efficiency of PSII; ETR(II): the electron transport rate of PSII; Y(NPQ): the quantum yield of regulated energy dissipation of PSII; Y(NO): the quantum yield of non-regulated energy dissipation of PSII; qP: the photochemical quenching coefficient. 1 - CK; 2 - TRE.
Effects of exogenous trehalose on the electron transport chain activities in wheat seedlings under different treatments. When subjected to heat stress, the electron transfer activities of the whole chain and PSII showed an obvious decrease in control wheat seedlings, while no significant change in PSI activity was obtained (Figure 4). During heat stress, trehalose pretreatment substantially increased the electron transport activities of PSI , PSII and the whole chain (Figure 4). However, after 24 h of recovery at room temperature (R24), only the electron transport activities of PSI and the whole chain were dramatically enhanced in trehalose pretreated plants (Figure 4), demonstrating that the increase in the electron transport activity of the whole chain was owing to the enhanced activity of PSI in trehalose pretreated plants.

Figure 4 Effects of exogenous trehalose on electron transport activities of wheat leaves under different treatments. PSII activity was determined by O2 evolution with 0.5 mM diphenylcarbazide (DPC) as an electron donor and 0.9 mM p-benzoquinone (BQ) as an electron acceptor. Electron transport activity of the whole chain was determined from the O2 uptake following addition of 0.1 mM methyl viologen (MV). PSI activity was measured as O2 uptake in the presence of 0.1 mM 2, 6 - dichlorophenol indophenol (DCPIP), 0.1 mM MV, 5 mM NaN3, 10 μM 3 - (3, 4 -dichlorophenyl) - 1, 1 - dimethylurea, 5 mM ascorbate and 1 mM potassium cyanide. The electron transport activities under different treatments were measured with a Clark-type O2 electrode. 1 - PSII (DPC-BQ) TRE: the electron transport activity of PSⅡ in seedlings pretreated with trehalose (DPC as electron donor and BQ as electron acceptor). 2 - PSII (DPC-BQ) CK: the electron transport activity of PSⅡ in seedlings pretreated without trehalose (DPC as electron donor and BQ as electron acceptor). 3 - PSI (DCPIP-MV) TRE: the electron transport activity of PSⅠ in trehalose pretreated seedlings (DCPIP as electron donor and MV as electron acceptor). 4 - PSI (DCPIP-MV) CK: the electron transport activity of PSⅠ in seedlings pretreated without trehalose (DCPIP as electron donor and MV as electron acceptor). 5 - Whole chain (H2O-MV) TRE: the electron transport activity of the whole chain in seedlings pretreated with trehalose (H2O as electron donor and MV as electron acceptor). 6 - Whole chain (H2O-MV) CK: the electron transport activity of whole chain in seedlings pretreated without trehalose (H2O as electron donor and MV as electron acceptor).
Effects of exogenous trehalose on PSII protein secondary structures in wheat seedlings under different treatments. During heat stress, the amount of orderly α-helix was reduced, while the amount of β-sheet, β-turns and β-antiparallel increased in control wheat seedlings (Table 1). However, the amount of orderly α-helix increased, while the amount of β-sheet, β-turns and β-antiparallel reduced in the seedlings of trehalose pretreatment group (Table 1). After recovery from heat stress, the amount of α-helix and β-turns were reduced, while the amount of β-sheet returned to normal in trehalose pretreated seedlings (Table 1).
Table 1 Effects of exogenous trehalose on the amide I band of PSII in wheat leaves under different treatments. CK: without trehalose pretreatment; TRE: trehalose pretreatment; H0: without high temperature treatment; H24: high temperature treatment for 24 h; R24: recovery of 24 h from high temperature treatment.
Treatments | H0 | H24 | R24 | |||
---|---|---|---|---|---|---|
Secondary structure | CK | TRE | CK | TRE | CK | TRE |
a-helical (%) | 7.98 ± 0.15 a | 6.78 ± 0.17 c | 6.63 ± 0.19 c | 7.58 ± 0.23 b | 8.10 ± 0.12 a | 6.65 ± 0.13 c |
β-sheet (%) | 23.49 ± 0.37 c | 22.88 ± 0.24 cd | 24.13 ± 0.25 b | 20.38 ± 0.30 d | 22.43 ± 0.28 cd | 24.71 ± 0.32 a |
β-turns (%) | 32.02 ± 0.29 c | 33.88 ± 0.35 a | 33.38 ± 0.31 a | 32.84 ± 0.42 b | 33.92 ± 0.37 a | 30.03 ± 0.28 d |
β-antiparallel (%) | 26.18 ± 0.34 c | 29.44 ± 0.39 a | 29.47 ± 0.28 a | 28.47 ± 0.34 b | 28.15 ± 0.25 b | 29.13 ± 0.41 ab |
Effects of exogenous trehalose on D1 protein content in wheat seedlings under different treatments. Wheat seedling leaves in the absence of SM showed a distinct D1 protein band in comparison with those in the presence of SM (Figure 5A). D1 protein content in control wheat seedlings decreased by 44.19 % under heat stress conditions, while in trehalose-plus-heat seedlings, D1 had an apparent increased by of 60.94 % compared with control plants, demonstrating trehalose pretreatment mitigated the decline of D1 protein content (Figure 5B). Under recovery conditions, D1 protein recovered in all treatment groups (Figure 5B). In the presence of SM, the reconstruction of active reaction centers by de novo synthesis of D1 protein was restrained while D1 protein degradation was still in process. No difference was seen in D1 protein content between trehalose pretreatment and control seedlings under normal temperature conditions in the presence of SM. However, the D1 protein content of trehalose pretreated seedlings was lower than that of control seedlings under high temperature and recovery conditions (Figure 5C).

Figure 5 A: Western-blot analysis of D1 protein from CK and TRE under different treatments. B,C: Influence of exogenous trehalose on D1 protein content without SM (B) or with SM treatment (C) in wheat leaves under different treatments, respectively. After seedlings pretreated with 1.5 mM trehalose for 3 d (TRE), with half-strength Hoagland solution as control (CK), then they were grown under the following conditions respectively: a temperature of 25 ºC (H0); high temperature (40 ºC ± 0.5 ºC) treatment for 24 h (H24). After that, they recovered at 22 ºC for 24 h (R24). Then, the treated seedlings were divided into two groups, one group treated with streptomycin (SM) and the other group treated without SM. Effects of heat stress on D1 protein in wheat leaves was measured by Western-blot analysis with chloroplast protein polyclonal antibody as the primary antibody and alkaline phosphatase enzyme-labeled antibody IgG (H + L) as the secondary antibody. The relative content of D1 protein was obtained by Image J software. Different letters show significant differences (P < 0.05). 1 - CK; 2 - TRE
Discussion
Chlorophyll fluorescence parameter analysis is a accurate and rapid technique to test and quantify the tolerance of the composition and function of photosynthetic apparatus to abiotic stresses (Kalaji et al. 2011, Killi et al. 2020). Compared with seedlings in the control group, trehalose pretreatment enhanced Fv/Fm, Fv/Fo, PIabs, TRo/RC, TRo/CSo, ETo/RC and ETo/CSo under heat stress conditions (Figure 1), indicating that trehalose-pretreated wheat seedlings had higher photochemical activity. It was observed that under heat stress, the Fv/Fm (Figure 3A), Y(II) (Figure 3B), ETR(II) (Figure 3C) and qP (Figure 3F) of trehalose pretreated seedings were higher and Y(NO) (Figure 3E) was lower than that of control seedings, suggesting that trehalose pretreatment alleviated the suppression of electron transfer rate and increased the proportion of active PSII reaction centers, photochemical activity and light energy conversion efficiency to maintain a high activity of PSII and relatively stable energy utilization efficiency (Essemine et al. 2017).
The amount of Fv was proportional to the degree of QA reduction, and the appearance of a J peak resulted from the reduction of QA to QA - (Hill et al. 2004). The slope of the initial rise and the height of J and P peaks were associated with the activity of PSII reaction centers. Suppressed P value was used as a qualitative indicator of OEC activity inhibition under heat stress (Jiang et al. 2006). In this research, elevation of fluorescence yield at the J-band showed that PSII reaction centers and electron transfer were inhibited (Figure 2A). Inhibition of PSII activity was confirmed by the decline of PSII actual photochemical efficiency (Figure 3F). Heat stress led to the suppression of PSII electron transport activity (Figures 3C, 4), thereby reducing the photochemical reaction rate and photosynthetic activity. Meanwhile, similar to the result in marine cyanobacteria (Zhang & Liu 2016), reduced electron transport activity of PSII (Figure 3C) was consistent with lowered activity of the whole chain (Figure 4). Reduced ETR(II) is crucial for plants to respond to heat stress, since the excessive decrease of the electron transport chain increases the generation of ROS, causing damage to highly sensitive D1 protein under heat stress (Chen et al. 2004, Marutani et al. 2012). The D1 protein acts a pivotal role in the photochemical reaction of PSII, so when subjected to heat stress, its damage could lead to the decrease in Fv/Fm (Figures 3A, 5) and the inactivation of PSII (Figures 1, 3).
Pretreatment of plants with trehalose improved the stability of D1 protein and alleviated the damage caused by heat stress (Figure 5B), which enhanced the recovery of PSII and maintained normal photosynthetic characteristics (Figures 1, 3A). If it is not affected by de novo D1 protein synthesis, the absolute rate of D1 protein degradation in vivo will be determined (Schnettger et al. 1994). When SM inhibited D1 synthesis, trehalose pretreated seedlings had lower D1 protein content at high temperature (H24) and room temperature (R24) (Figure 5C), indicating that trehalose pretreatment resulted in more degradation of D1 protein. However, higher net D1 protein content was seen in trehalose-pretreatment groups under heat stress in the absence of SM (Figure 5B), which suggested that trehalose pretreatment likely maintained high D1 levels by accelerating the D1 protein turnover rate. When the de novo synthesis of D1 protein was blocked by SM, its degradation was accelerated by trehalose. This phenomenon indicated that trehalose promoted both the degradation and synthesis of D1 protein under heat stress, resulting in higher content of D1 protein in the absence of SM.
PSII is known as the most temperature-sensitive component of the photosynthetic apparatus. Heat stress destroyed the PSII protein secondary structures, leading to a reduction in D1 protein turnover rate (Figure 5B). Additionally, the amount of α-helix, one component of D1 protein, was reduced, but the amounts of β-sheet and β-turn were enhanced when subjected to heat stress (Table 1). These variations in protein conformation reflected the degeneration of the PSII complex under heat stress, mostly caused by damage to amino acid side chains in the internal protein and hydrogen bonds stabilizing protein secondary structures. The increase in the content of β-antiparallel content might be caused by increased polymerization. Trehalose can influence the hydration degree of proteins, causing them to adopt a more optimal conformation (Mamedov et al. 2015). Under heat stress, protein secondary structures in trehalose-pretreated seedlings remained relatively stable, especially ordered α-helix content, which was observably higher than in the control (Table 1). This indicated that trehalose pretreatment could protect the protein secondary structures of PSII reaction center complex, which was similar to a previous report involving spray dried poly-L-lysine (Mauerer & Lee 2006). The protective effect of trehalose on the PSII reaction center complex may be due to the stimulation of circulating electron flow (Luo et al. 2021).