Land use and cover change leading to deforestation and fragmentation of habitat is one of the global environmental changes that most negatively affects Earth’s ecosystems (Sala et al. 2000). Such massive and omnipresent loss of habitat is a major driver of the current and committed biological extinction (i.e., extinction debt) of the Anthropocene (Dirzo & Raven 2003, Laurance & Useche 2009, Ceballos et al. 2017), affecting biodiversity in terms of genetic erosion (Young et al. 1996), population extinctions and declines (Hughes et al. 1997, Ceballos et al. 2017), promoting global species loss (Pimm & Brookx 2000, Ceballos et al. 2017) and leading, potentially, to the entire loss of some ecosystems (López & Dirzo 2007). Biodiversity loss associated with land use change is particularly critical in tropical ecosystems since they concentrate a disproportionate amount of the Earth’s biodiversity and are being, at the same time, heavily impacted (Achard et al. 2002, Dirzo & Raven 2003, Lepers et al. 2005, Malhi et al. 2014). Currently, the predominant spatial configuration of tropical landscapes is that of an archipelago of vegetation remnants of different size immersed in a sea of transformed terrains consisting of agricultural fields, cattle grasslands, plantations, urbanization or other human-dominated systems (Malhi et al. 2014, Camelo et al. 2017). While the massive conversion of tropical forests into cleared lands for agriculture and cattle grazing leads to a rapid loss of populations and species, the remaining fragments and some local management types (e.g., silvopastoral systems, live fences) have the potential to maintain some indigenous species (Ruíz-Guerra et al. 2014).
Literature reviews of the patterns and biological consequences of tropical fragmentation (e.g., Laurance & Bierregaard 1997, Fahrig 2003, Zemanova et al. 2017, Storck-Tonon & Peres 2017) show that most of the studies have been conducted in the South American Neotropics (e.g., Central Amazonia: Laurance & Bierregaard 1997, Laurance et al. 2002) and most of the studied organisms are vertebrates (particularly birds), with a lower representation of studies dealing with other organisms. In the case of plants, studies in Central Amazonia and other tropical rain forests (e.g., Laurance & Bierregaard 1997, Laurance et al. 1998a, b, Laurance et al. 2000, Hill & Curran 2003), have documented increased mortality rates of canopy trees in fragments compared to continuous forest (Laurance & Bierregaard 1997, Laurance et al. 1998a, b,Santo-Silva et al. 2016), and increased abundance of pioneer trees and lianas in fragments or fragment edges (Laurance et al. 1998a, b, Laurance et al. 2000, Thier & Wesenberg 2016). Apart from these studies, largely focusing on adult trees, other studies with juvenile plants (including seedling and saplings) in Central Amazonia and in Los Tuxtlas rainforest (Benítez-Malvido & Martínez-Ramos 2003, Sánchez-Gallen et al. 2010) show that fragmentation significantly reduced species richness and reveal species composition shifts among fragments. This suggests that, by affecting the plants of the understory, fragmentation has the potential to disrupt forest regeneration and, subsequently, overall plant species diversity (Martínez-Ramos et al. 2016b). With the exception of these studies and few isolated investigations elsewhere (e.g.,Zhu et al. 2004), research examining the consequences of fragmentation on plant species composition and recruitment of young plants is limited. The need to examine how fragmentation affects species richness, composition and the relative representation of plants of different growth forms and functional groups and involving individuals of a wide range of size classes that become established and transition from understory saplings to the size class of mature trees motivates the present study. The rationale for our comparison of a range of sizes is that assemblages of adult trees should have been similarly diverse throughout the forest prior to fragment separation. Therefore, fragmentation should not have as marked an effect on the richness of this size class—unless significant edge—related mortality has proceeded (Laurance et al. 1998a,b, Laurance et al. 2000). In contrast, small-sized plants reflecting post-fragmentation recruitment, should show stronger decline (Benítez-Malvido & Martínez-Ramos 2003). This pattern should be particularly the case of mature-forest, shade tolerant species, many of which are especially vulnerable to changes in abiotic factors (e.g., water stress, soil or air humidity or wind damage: Turner 1996, Scariot 1999, Benítez-Malvido & Martínez-Ramos 2003, Lasky et al. 2013, Jin et al. 2020) and biotic factors such as pollinators and dispersers (e.g., Cordeiro & Howe 2001, Osuri et al. 2017). This latter expectation is consistent with documentation of the negative impact of fragmentation on animals that play such roles (e.g., Aguilar et al. 2006, 2008, Cramer et al. 2007, Moran & Catterall 2014). In contrast, light-demanding species are less likely to be affected by the abiotic changes associated with fragmentation. Thus, we posit that these species should have the potential to recruit in small fragments (Tabarelli et al. 2008, Santo-Silva et al. 2016).
We examine such expectations by quantifying several metrics of plant diversity associated with fragmentation in a tropical rainforest area in southeast Mexico. We aimed to specifically answer the following questions: i) How does species richness vary across a range of fragment sizes representative of the current fragmentation in the area, and does it vary with plant size (diameter) structure of the community? In addressing this question, we hypothesized that if forest fragmentation reduces plant species richness, the effect should be more pronounced when including the saplings (established post-fragmentation), and that such effect should be diluted or not evident in the larger trees; ii) to what extent is species composition and floristic similarity affected by forest fragmentation? For this question, we hypothesized that, if plant species differ in their ability to recruit or withstand the effects of fragmentation, species composition should change with fragmentation, and floristic similarity should decrease (i.e., floristic differentiation); and iii) does habitat fragmentation affect the relative representation of species with different regeneration patterns? For this question we hypothesized that if we separate species according to their light requirements for regeneration —that is light-demanding versus shade-tolerant species— the former should be better represented in the smaller fragments while the latter should have a greater relative representation in the largest fragments or continuous forest.
Materials and methods
Study site. This study was carried out in Los Tuxtlas, Veracruz, Mexico. This area is the easternmost extension of the Trans-Mexican Volcanic Belt and constitutes the northernmost limit of tropical rainforest distribution on the Americas (Dirzo & Miranda 1991). The topography is complex, with elevational changes from sea level to 1,600 m asl. within a short distance (García-Aguirre et al. 2010). Given its latitudinal position (18º 39’) and location, the area experiences high precipitation — around 4,700 mm/yr and mean annual temperature is 25 ºC— (Dirzo et al. 1997). The predominant vegetation is tropical rain forest in the lowlands, with variations along the elevational range, including cloud forest and mixed forests at higher elevations (García-Aguirre et al. 2010). Floristic diversity in the area includes a total of 950 known vascular plants (Ibarra-Manríquez et al. 1997). The flora is largely composed of plants of neotropical origin, but higher elevation sites include combinations of Neotropical and Nearctic taxa (Dirzo & Mendoza 2004).
The area has a long history of human occupation. Significant anthropogenic impacts became apparent starting in the 16th century with the Spanish colonization (Dirzo & Mendoza 2004). The predominant land use in the area is conversion of forest to cattle grasslands. Rates of annual deforestation in the decades of the 60’s-late 80’s were ~ 4.3 % (Dirzo & García 1992). Current deforestation rates have decreased because forest remnants are restricted to the most inaccessible areas of the region and the protected lands of the Los Tuxtlas Biosphere Reserve. The current spatial configuration of the landscape consists of a large number (92 % out of 1,005 in an area of ca. 1,000 km2) of small/very small fragments (< 1.0 ha), with only a few extensive areas still remaining (Mendoza et al. 2005, García-Aguirre et al. 2010). In the northern part of Los Tuxtlas, corresponding to our study site, the only remnant of continuous forest corresponds to the Los Tuxtlas Tropical Research Station (700 ha), and its extension towards the San Martín Volcano, encompassing an area of ca. 9,500 ha. For details of the natural history of the region see González-Soriano et al. (1997).
The selected fragments. Given the skewed frequency-distribution to small size fragments in Los Tuxtlas (Mendoza et al. 2005), we chose a group of four fragments and one relatively continuous forest site, using digital aerial photography (scale 1:75,000) processed using ILWIS (ITC 1993) to calculate areas of each fragment and distances between fragments and continuous forest. With this information and complementary ground-truthing we chose isolated fragments that: i) bore the same vegetation type, tropical rain forest, and therefore were located within a restricted elevation (0-150 m); ii) had a similar time since the area’s fragmentation occurred, and iii) large enough to retain adult plants present in the forest previous to fragment separation. Our selection included fragments of 2, 4, 19.4, and 34.6 ha, and an extensive area (700 ha) here referred to as continuous forest (CF; see Aguirre & Dirzo 2008). The overall area where fragments and CF are located extends from the lowlands westward towards the San Martín Volcano and harbors the same vegetation type.
Sampling protocol. We used the method developed by Gentry (1982, 1988) to assess plant species diversity, with one modification regarding the size of plants he included (diameter at breast height [1.3 m], DBH > 2.5 cm). In each fragment and CF we established ten independent, randomly selected, 50 × 2 m transects located toward the physical center of each site. Thus, edge effects (e.g., Laurance et al. 1998a, 2000) would be commensurate to the size of the fragments. Within each transect, we recorded all woody rooted plants with DBH ≥ 1.0 cm (cf., Gentry’s 2.5 cm). For all trees, we measured their DBH except in the case of trees with large buttresses (≥ 1.3 m height), for which we measured diameter immediately above the buttresses. For lianas we measured the diameter at the base of the stem. For hemi-epiphytic plants (e.g., strangler figs) we measured (at 1.3 m) the thickest trunk that reached the ground. In terms of contrasting the plant diametrical structure across the fragments, we established three categories: a) all plants, including all individuals with DBH ≥ 1.0 cm (those that most likely colonized and recruited into the study sites post-fragmentation); plants with DBH > 2.5; and large trees, those with DBH ≥ 10.0 cm (potentially old individuals surviving the original fragmentation process). All plants were identified to species, following the nomenclature of Ibarra-Manríquez & Sinaca (1997). Voucher specimens were deposited in the Herbarium of the Los Tuxtlas Station.
Metrics of floristic diversity. With the obtained data set we calculated the following metrics:
Species richness.- Defined as the number of plant species/0.1 ha considering all three size categories, separately or collectively. To examine the variation of species richness across fragments we used linear regression. Given our field sampling effort of 0.1 ha we assessed sampling effort via non-parametric estimates of species richness using EstimateS 7.5 (Colwell 2005). We calculated a non-parametric estimator of overall richness, Chao-2, commonly used in species richness studies (Chazdon et al. 1998).
Changes in floristic composition among fragments.- With the identity of the species in the ten transects of each fragment we developed a matrix of abundance and performed a non-metric multi-dimensional scaling (NMDS) using the Bray-Curtis similarity index, a widely method used to generate distance matrices in vegetation ordination studies (Gotelli & Ellison, 2004). Significant differences in composition across fragments were tested using Analysis of Similarities (ANOSIM) and Similarity Percentages Analysis (SIMPER) was used to identify the species that contributed most to between-fragment differences. All tests were based on 9,999 permutations performed with Past 4.03 (Hammer et al. 2001).
In addition, we estimated the floristic similarity of all possible pairs of fragments, using a modification of the classical indices of compositional similarity, as proposed by Chao et al. (2005). The proposed estimators consider species abundance, the effect of unseen/rare species (as is the case of our study site, Ibarra-Manríquez et al. 1997) and produce values that are considerably less biased than traditional indices. We calculated Chao-Sorensen similarity indices using EstimateS 7.5 software (Colwell 2005). Further details of the advantages and accuracy of the modified indices are described by Chao et al. (2005). In order to analyze possible species groups using Chao-Sorensen indices, we applied UPGMA grouping analysis with Past 4.03 (Hammer et al. 2001).
Relative representation of mature-forest species and early successional species.- The definition of these two regenerating strategies was based on our previous knowledge of the species of this forest and on published information for plants specifically from Los Tuxtlas (Martínez-Ramos 1985, Bongers et al. 1988, Ibarra -Manríquez et al. 1997). This analysis was performed with both the number of species and individuals of the two strategies across fragment sizes. Relative representation of the two regeneration strategies was tested for independence from fragment size using a Chi-square test of independence.
Importance Value (IV) of the species.- We calculated, for each of the species: density (no. plants/0.1 ha), frequency (the number of transects, out of ten, in which a given species appeared in each fragment or CF) and dominance (the sum of all basal areas obtained from DBH). The relative values of these three measures were summed to calculate IV for each species (Curtis & McIntosh 1951). We used the ten species with the highest IV for each fragment to assess changes in relative dominance of light- demanding/shade-tolerant species with fragmentation.
All these metrics of floristic diversity were obtained from sampling of 0.1 ha plots in fragments and CF.
Results
Changes in floristic diversity. Considering all plants with DBH ≥ 1.0 cm, the number of species per 0.1 ha plot (i.e., site) ranged from 102 (in the second smallest fragment) to 135 (CF). Our values of completeness (by Chao-2) were always above 60 %, with values of 62 % for the fragment of 34.6 ha, and 75 % for the 19.4 ha fragment. The values for plants ≥ 2.5 cm DBH ranged from 79 % (second smallest fragment) to 95 % (CF). For large plants (DBH ≥ 10.0 cm) the range of variation in richness was 31 (smallest fragment) to 40 species (second largest fragment). The observed variation was consistent with our expectation of a reduction in species richness as remaining habitat area decreases considering all plants (DBH ≥ 1 cm) (F = 66.02, P = 0.003; Figure 1), and fragment size explained 95 % of the variation. When we considered the non-parametric estimate of species richness, Chao2, the estimated range was 145-180; however, the regression Chao2-fragment size reveals no significant relation between fragment size and species richness (R = 0.59, F = 4.4, P = 0.15) (Figure 1).

Figure 1 The relationship between area of fragment/site and number of species per 0.1 ha in a lowland tropical rain forest in Los Tuxtlas, southeastern Mexico. Species numbers are derived from a non-parametric estimator, Chao-2, and field observations for plants in three size categories: DBH ≥ 1 cm, DBH ≥ 2.5 cm, and DBH ≥ 10 cm.
A similar trend in observed species was detected when we excluded the smallest plants and only considered plants with DBH ≥ 2.5 (Figure 1), but this relationship became non-significant (F = 2.64, P = 0.20), although 46 % of the variation in species richness was explained by fragment size. Finally, when we considered only the largest plants (DAP ≥ 10.0) (Figure 1), species richness was clearly independent of fragment size (F = 0.09, P = 0.78).
Changes in species composition. The NMDS (Figure 2) detected a differentiation across fragment sizes (Stress = 0.19429), with an evident separation of CF and the 2-ha fragment from the rest, while the remaining constituted a constellation of points situated at the center of the axes. The ANOSIN analysis confirmed this contrast, also showing an overall significant difference among fragments (R2 = 0.3256, P < 0.001) in terms of composition. The SIMPER analysis showed that these differences are explained, at least partly, by the presence of a set of mature-forest, shade-tolerant species (e.g., Astrocaryum mexicanun, Chamaedorea spp., Psychotria spp., together representing 10 % of the total dissimilarity of all groups) and, on the other hand, the presence of a contingent of light-demanding species (e.g., Myriocarpa longipes, Siparuna andina and Trophis mexicana with 8 % of total dissimilarity). The resulting dendrogram reveals two distinguishable floristic groups (Figure 3) with no overlapping in similarity values: group b has values greater than 0.84 (Mean = 0.877, SD = 0.025), while those of group a are all lower than 0.81 (Mean = 0.785, SD = 0.027). In addition, values in group b do not include a single paired comparison involving CF and we refer to this as “the fragments group”. In contrast, four out of six paired comparisons in the group a involve CF, suggesting that the lower floristic similarity in this group is largely explained by the comparisons between CF and fragments. We refer to this as “the continuous forest group”. Although two of the paired comparisons in this group do not involve CF, a U-test showed that the median floristic similarity is significantly lower in the CF group than in the fragments group (Z = 2.56, P = 0.01) indicating that, in general, similarity was greater among fragments than between fragments and continuous forest.

Figure 2 A non-metric multi-dimensional scaling (NMDS) analysis based on the abundance of species in the 10 transects from each fragment and continuous forest in a lowland tropical rain forest in Los Tuxtlas, Mexico.
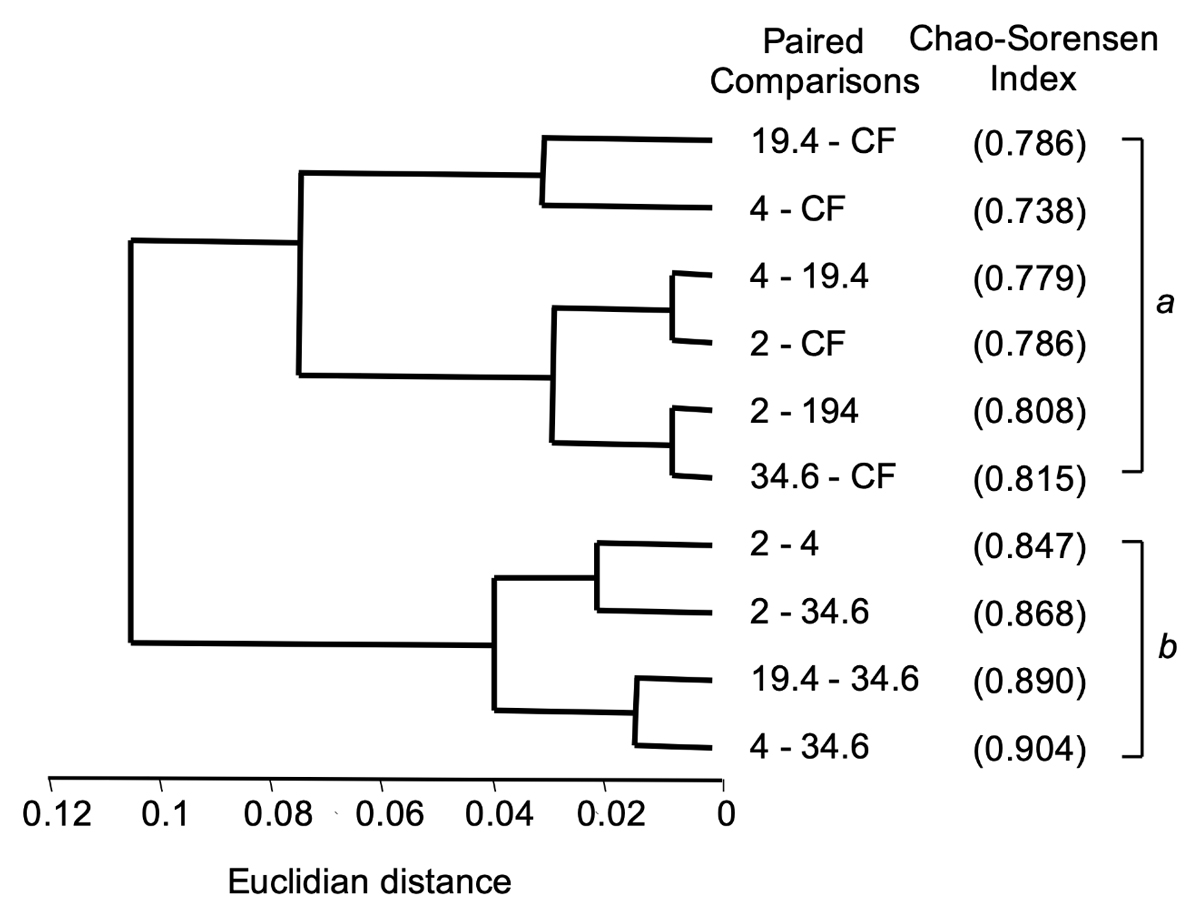
Figure 3 A grouping analysis, UPGMA, of the Chao-Sorensen similarity index applied to all possible pairs of fragments and continuous forest (CF). Letters a and b denote the two major clusters corresponding to the continuous forest group of pairs and the fragments group of pairs. In addition, both clusters (a and b) denote statistically significant differences in Chao-Sorensen values.
Changes in species representation according to regeneration strategy. The number of species belonging to the two regeneration strategies varied across fragments and such heterogeneity also differed in their magnitude depending on plant diameter category (Figure 4, left-hand side panels). Considering all plants with DBH ≥ 1.0 cm (Figure 4A), the relative abundance of plant regeneration type showed an overrepresentation of shade-tolerant species in CF as compared to light-demanding species. Such contrast in the proportion shade-tolerant:light-demanding species is lower in the smallest fragment, as well as in fragments of intermediate size. We found a significant heterogeneity in the distribution of regeneration strategy across fragment sizes (χ2 = 11.6, P = 0.02). In the case of plants with DBH ≥ 2.5 cm (Figure 4B) a similar tendency was observed: the overrepresentation of shade-tolerant species as compared to light demanding taxa was greater in CF than in all forest fragments (χ2 = 32.0, P < 0.001). Plants of the larger diameter size category (DBH ≥ 10.0 cm) (Figure 4C) did not show a significant contrast across fragment sizes (χ2 = 3.1, P = 0.54).
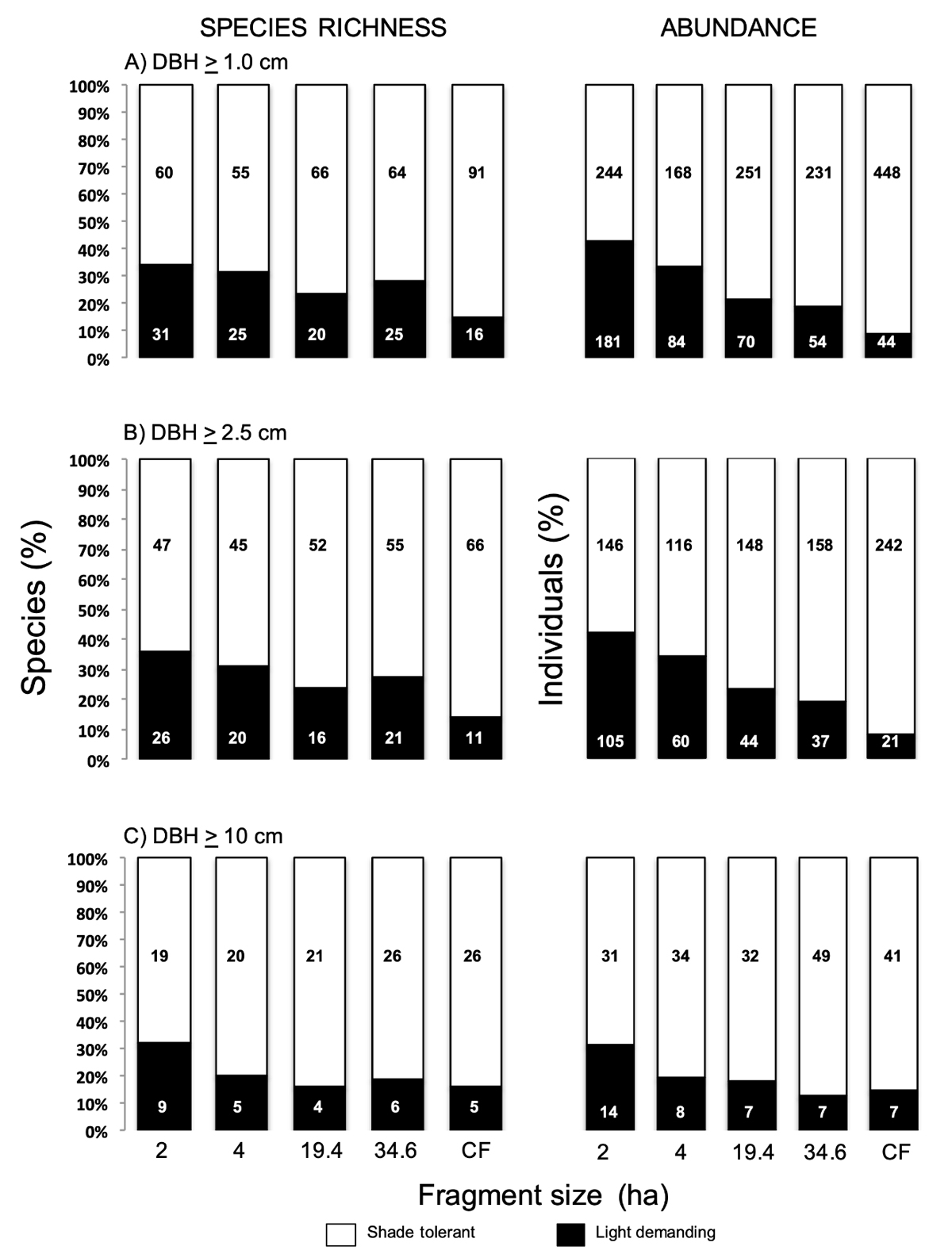
Figure 4 Relative representation of species richness and abundance of shade-tolerant and light-demanding species in fragments of different size and continuous forest (CF) from a lowland tropical rainforest at Los Tuxtlas, separated according to plant size category: A) DBH ≥ 1 cm, B) DBH ≥ 2.5 cm, C) DBH ≥ 10 cm. Panels on the left correspond to percent of species richness (number of species per 0.1 ha) and panels on the right correspond percent to abundance (number of individuals per 0.1 ha). For details of contingency analyses comparing the distribution of both plant types in relation to fragment size see text.
The observed pattern of relative representation of plants of the two regeneration modes becomes more evident when considering plant abundance. In the smallest size category, the contrast was highly significant (χ2 = 156.6, P < 0.001), followed by a still highly significant heterogeneity in the case of plants ≥ 2.5 DBH (χ2 = 91.0, P < 0.001), while the relative representation of the two strategies does not differ significantly with fragment size in plants ≥ 10.0 cm DBH (χ2 = 6.5, P = 0.16).
A contrasting pattern is also evident when comparing the Importance Value across sites (Figure 5), particularly considering the two extremes of fragment size. In the smallest fragment, two light-demanding species were present among the ten most important taxa (collectively accounting for 48 % of the total sum IV of this site), with IV of one of them (Myriocarpa longipes) being > 4-times higher than that of the other species. In great contrast, no light-demanding species is present among the ten most important taxa of CF in this site. In CF the ten most important species included two understory palms and eight tree species typical of mature forest. The other three fragments insinuated a gradient of dominance of light-demanding species. Overall, the sum of the IV of the ten most important light-demanding species shows a clear gradient across fragment sizes in the direction 2 ha (47.6 %) > 4 ha (34.3 %) > 19.4 ha (26.2 %) > 34.6 ha (6.2 %) > CF (0 %).

Figure 5 The ten species with the greatest value of importance in each of the studied fragments, including shade-tolerant and light-demanding species. (*) Correspond to Astrocaryum mexicanum (Arecaceae), the most abundant mature-forest plant species in the understory at Los Tuxtlas. Species codes correspond to the first two letters of the genus and species.
Discussion
Our study, based on the accurate identification, measurement of size and analysis of the distribution of 3,200 plants in 282 species across fragments and continuous forest provided consistent information regarding our expectations and three major questions.
Regression analyses of the observed species richness vs. fragment size show that richness decreased by about 22 % from CF to the smallest fragment. Species richness decline may be related to the prevalence of species with intrinsically low local population size (rare species) and mature-forest species unable to withstand the effects of fragmentation (Hubbell & Foster 1983). This is consistent with the fact that a large proportion of the species we sampled (45 %) comprised singletons, and singletons plus doubletons constituted an even larger proportion (65.2 %). Even if a moderate proportion of these species were intolerant of the environmental changes brought about by fragmentation, this would translate into a significant decline in species richness in fragments.
Regarding our hypothesis that the effect of forest fragmentation would be diluted as plant diameter increases, we found that, indeed, for the plants in the largest size class (which include a contingent of species present prior to fragmentation), the effect was muted. Although winds can be strong in this region (González-Soriano et al. 1997), windthrow does not seem to have affected large trees yet, in contrast to what has been observed in Amazonia (Laurance et al. 1998b, 2000). Our findings of a lack of relationship between species richness and fragment size for the larger plants are contrasting with those of other authors (Turner 1996, Hill & Curran 2003, Zhu et al. 2004, Page et al. 2010), yet it remains to be seen whether the lack of effect in the large trees we observed is a transient state of a trajectory of species richness decline already in motion. Studies of long-term monitoring and comparisons among fragments that have been isolated for longer periods (e.g., Turner 1996, Hill & Curran 2003) are warranted.
Our findings may represent a next stage in the fragmentation-driven decline of seedling species richness, as was documented by Benítez-Malvido & Martínez-Ramos (2003). Sánchez-Gallen et al. (2010) found opposite trends: major species richness and diversity of seedlings in smallest fragments. This might be due, the authors argue, to the high connectivity afforded by some elements in the anthropogenic matrix (e.g. living fences, riparian vegetation) that could facilitate seed dispersal. A long-term experimental study to determine if such seedlings can survive and become recruited to a subsequent stage in remnant forest fragments is clearly warranted.
We also detected a shift in species composition. In general, consistent with our expectation, the species from CF comprised a floristic contingent distinguishable from the assemblages of the fragments, and such shifts were associated with the prevalence of light-demanding species in the fragments. In general, similarity was greater among fragments than between fragments and continuous forest, as in other studies (see Scariot 1999, Benítez-Malvido & Martínez-Ramos 2003). However, floristic composition/similarity can be influenced by distance among fragments (spatial autocorrelation) or the landscape configuration (Arroyo-Rodríguez et al. 2009, 2013). We argue that this is unlikely in our case, as floristic differentiation in our study site is statistically independent of distance of fragments (rank correlation distance-similarity rs = 0.12, P = 0.72). We therefore posit that fragmentation sets the vegetation in a trajectory of floristic differentiation.
Our comparison of plants of two regeneration strategies (light-demanding pioneers and shade-tolerant, mature forest species) suggests differential susceptibility to fragmentation: proportional impoverishment of mature-forest species and increased richness of pioneer species in fragments. Indeed, in contrast to shade tolerant species, pioneer species have life history traits that reflect selection for resistance to fragmentation-related effects (edge effects, light availability, dessication) (Arroyo-Rodríguez et al. 2007, 2009, Chazdon et al. 2007, Martínez-Ramos et al. 2016a). Therefore, when we analyzed the ranking of Importance Value across fragments and CF, we found that while the prevalent species in CF were shade-tolerant, mature-forest taxa, the smallest fragment had a marked dominance of light-demanding plants. This suggests that the future physiognomy of fragmented landscapes will not be given only by the prevalence of light-demanding species in edges, roadsides, and clearings, but by forest remnants dominated by such species. Such dominance of pioneer species in small forest fragments will reflect not only reduced species richness, but reduction in functional diversity too (Carneiro et al. 2016, Lohbeck et al. 2016, Martínez-Ramos et al. 2016 b, Muscarella et al. 2017).
The observed changes in plant diversity metrics described here may also cascade into indirect effects on animals that depend on plants for their survival (e.g., vertebrate and invertebrate pollinators, frugivores and herbivores; Aguirre & Dirzo 2008, Ruíz-Guerra et al. 2010, Aguirre et al. 2011). Another aspect related to higher-order effects, is the likelihood of invasion by exotic species, a recognized driver of biodiversity loss (Sala et al. 2000). However, remarkably, out of the 3,200 plants we observed and measured, not a single one belonged to an exotic taxon, even in the case of transects located close to the edge of the smallest fragment. Resistance to invasions in diverse ecosystems has been documented in other studies at local and global scales (Whitmore 1991, Sala et al. 2000, Joshi et al. 2015).
Our main findings, including a reduction in species richness; a significant shift in species composition; and a shift in the relative representation of plant regeneration strategies associated to forest fragmentation, reveal the intricacies of the amply recognized importance of land use change for tropical biodiversity maintenance (e.g., Laurance et al.2002). Although our emphasis was on diversity metrics based on plant species and regeneration strategies at a local scale, implicit in our findings is another critical aspect of biodiversity decay: local population loss and declines in abundance (see Hughes et al. 1997, Ceballos et al. 2017), with potential local-level consequences on ecosystem processes and services (Dirzo & Raven 2003).
These rapidly changing floristic configurations may have profound effects on our efforts to conserve, manage, and restore ecosystems, as well as the services they provide to local populations. Going forward, we need to consider how to preserve biodiversity and ecosystem services amid anthropogenic landscapes, and to carefully examine how remnant forest fragments can play a role (e.g., as biological corridors) in tropical ecosystem restoration efforts. Finally, the study of synergies between land use and other anthropogenic impacts, such as climate change (Esperon-Rodríguez et al. 2019), and their effects on biodiversity in several tropical regions and considering multiple taxa represents a critical, pending agenda.