Tillandsia recurvata L. (ball moss) is a common epiphyte in America, distributed from the southeastern United States to southern Argentina (Smith & Downs 1977). Ball moss grows on many forest species. In recent years, Guevara-Escobar et al. (2011) suggested that forest disturbance and climate change may increase the number of individuals on different hosts. Excessive growth of T. recurvata can cause injuries to the hosts (Montaña et al. 1997, Soria et al. 2014) or reduce host growth (Flores-Palacios et al. 2014), thus modifying reproductive effort (Castellanos-Vargas et al. 2009). Other studies on epiphytic flora, report higher abundances of Tillandsia species in open or perturbed sites (Aguirre et al. 2010, Poltz & Zotz 2011). In semiarid areas, this particular interaction has received less attention (García-Suárez et al. 2003, Bernal et al. 2005, Escutia-Sánchez 2009).
The interaction between Tillandsia spp. and their hosts is a well-known phenomenon (Benzing 1990, Wagner et al. 2015); however, bark structural characteristics at the bark-root interface have been poorly documented. For example, detailed bark structural changes associated with T. recurvata establishment, i.e. a wounded periderm, were described in Parkinsonia praecox and Prosopis laevigata (Páez-Gerardo et al. 2005, Aguilar-Rodríguez et al. 2007). However, the nature of the chemical compounds present in this bark-root interface is unknown. Our hypotheses were that structural and chemical changes similar to those produced by damage can be equally documented in the bark of different hosts when ball moss is present. The aims of this study were to describe bark morpho-anatomical and histochemical changes caused by the interaction between ball moss and their hosts.
Material and methods
Four host species (Crataegus mexicana, Parkinsonia praecox, Prosopis laevigata, and Pittocaulon praecox, Table 1, Figure 1) were selected for this study because of their contrasting bark surfaces and because in recent years, abundance of Tillandsia recurvata seems to have increase in these species (Ernesto Aguirre-León, unpublishing data). Sixty nine branches were collected in total during dry season. Three branches were collected per tree, in two to 12 trees per host species (Table 1, Figure 1D-F). The three branches had ca. 3 cm in diameter and were sectioned with a saw in cylindrical samples (2 × 3 cm), free from epiphytes or hosting epiphytes. We ensured that epiphyte roots remained fixed to the bark and in those branch regions without epiphytes, areas free of scars and thorns were selected to avoid any effect they may have. All segments were fixed in formalin-alcohol-glacial acetic acid (0.5:9.0:0.5, Ruzin 1999) for 48 h.
Table 1 Species, localities and vegetation types where samples were collected. All collection numbers correspond to S. Aguilar-Rodríguez and were deposited in the herbarium FESI. The collection number corresponds to each individual sampled.


Figure 1 Ball moss growing on different hosts. A) Prosopis laevigata. B) Parkinsonia praecox. C) Crataegus mexicana. D) Detail of branch cut, Parkinsonia praecox. E) Detail of Prosopis laevigata branch with and without epiphyte. F) Detail of Pittocaulon praecox branch with and without epiphyte. Arrows indicate the place where branch with and without ball moss were sectioned for anatomical studies.
Anatomy. Transverse and longitudinal sections (20-25 µm thick) were cut using a sliding microtome, including bark and wood. For anatomical descriptions, selected sections were stained with safranin-fast green and mounted with synthetic resin. In the case of Pittocaulon praecox, samples were dehydrated in a Leica automatic TP1020 changer and embedded in paraffin, sections 15 µm thick were cut with a rotary microtome, stained with safranin-fast green (Ruzin 1999), and mounted with synthetic resin. In order to maintain the epicuticular wax layer of P. praecox, some sections were stained with safranin-crystal violet and mounted in glycerin jelly. Other sections were used to perform the histochemical tests. The anatomical descriptions were made following the terminology of Trockenbrodt (1990) and the histochemical techniques were performed according to Ruzin (1999). Sudan IV was used to detect lipids, vainillin was used to test for condensed tannins (catechins/proanthocyanids, Broadhurst & Jones 1978), ferrous sulfate to test for hydrolysable tannins, tannic acid-ferric chloride to test for calcium pectate, and Lugol’s solution to test for starch. To detect lignin, sections were treated with phloroglucinol and hydrochloric acid. The intensity of the reactions was recorded according to a ranked scale (0: negative, 1: faint, 2: moderate, 3: intense).
Statistical analysis. Fifteen variables (Tables 3) were analyzed with a principal coordinate analysis (PCoA), because we had a combination of 14 categorical variables and one continuous one. PCoA is an exploratory technique in which the inertia is analogous to the concept of variance in principal component analysis and it is proportional to the chi-square. For each condition with and without ball-moss, the 23 hosts studied were included in the analysis. We observe for those variables that better contributed to explain the variance associated with ball moss presence. The analysis was performed using SAS statistical program (Release 9.2 SAS Institute Inc., Cary, NC).
Results
Traces of damaged bark tissues in the bark-root interface in Parkinsonia praecox, Prosopis laevigata, Crataegus mexicana, and Pittocaulon praecox were found, when compared to unoccupied bark. These findings were at the histochemical and anatomical level (Tables 2, 3, Figures 2-4) (3).
Table 2 Bark characteristics of Parkinsonia praecox (from Páez-Gerardo et al., 2005), Prosopis laevigata (from Aguilar-Rodríguez et al., 2007), Crataegus mexicana, and Pittocaulon praecox. STE-CC = sieve tube element-companion cell, PA = parenchyma.

Table 3 Comparison of the histochemical observations and anatomical characteristics in the bark of the four host species. WB: without ball moss, BM: with ball moss. Histochemical reactions. 0 = negative, 1 = positive faint, 2 = positive moderate, 3 = positive intense. Anatomical characters. Texture: 0 = smooth, 1 = weakly fissure, 2 = weakly ridged, 3 = modified. Rhytidome: 0 = absent, 1 = present. Number of phellem layers: 0 = absent, 1 = < 10 strata, 2 = ± 15 strata, 3 = > 20 strata. Phellem cells: 0 = absent, 1 = no collapsed, 2 = collapsed. Number of phelloderm layers: 0 = absent, 1 = 1-3 strata, 2 = ≥ 5 strata. Crystals in the outer cortex: 0 = absent, 1 = present. Wounded periderm: 0 = absent, 1 = present. Lipidic substance: 0 = absent, 1 = present.

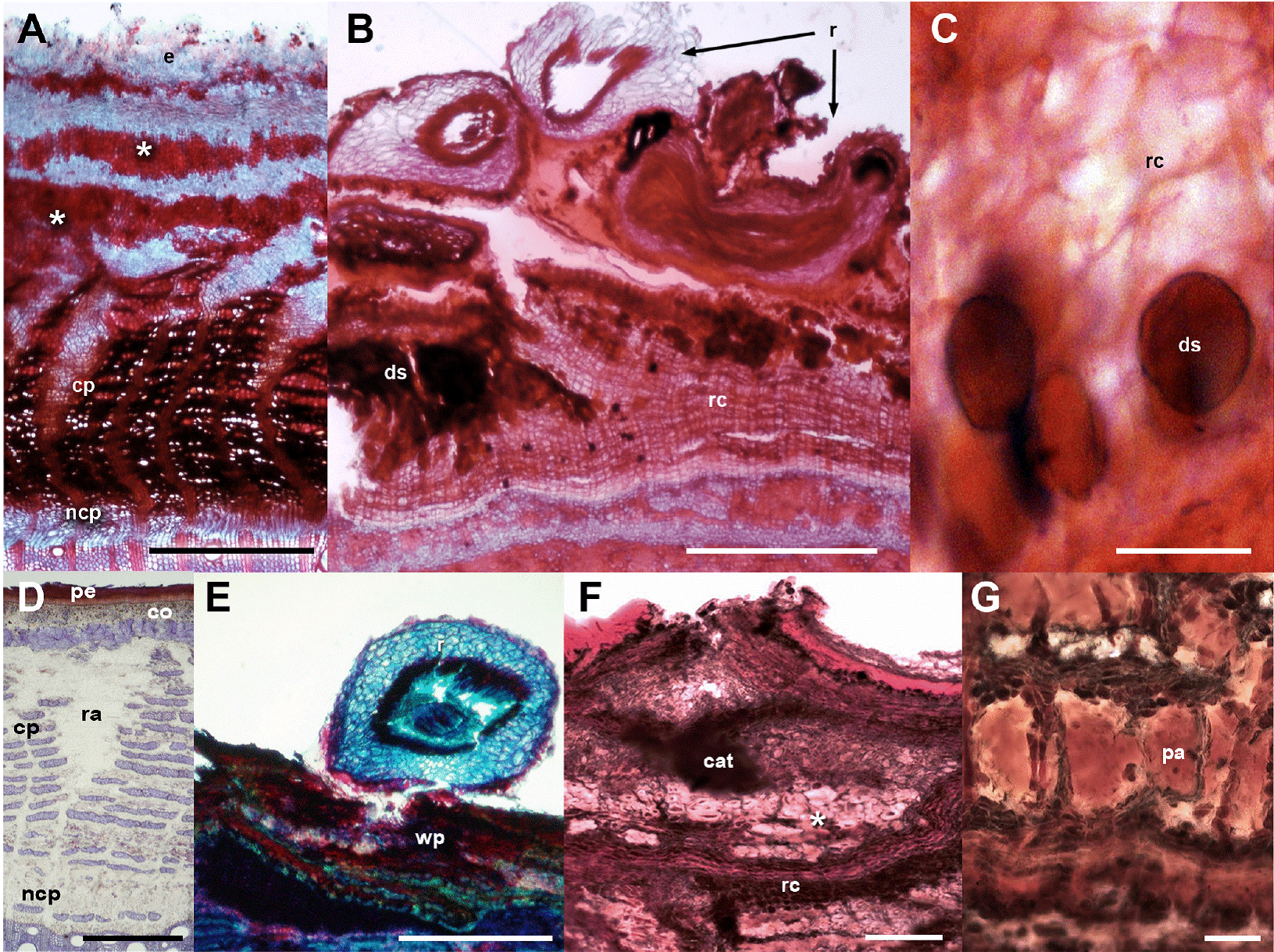
Figure 2 Fabaceae barks with and without ball moss. A-C. Parkinsonia praecox. A) Bark free of ball moss, transverse-section. B) Attached roots of ball moss. C) Positive suberin reaction in radially expanded cells. D-G. Prosopis laevigata. D) Bark free of ball moss, transverse-section. E) Root of ball moss attached and proliferation of multiple cell layers. F) Positive reaction to condensed tannins associated to reaction bark. G) Detail of parenchyma cells with condensed tannins. Scale bar: A = 250 μm, B, C, F = 500 μm, D, E = 50 μm, G = 100 μm. Asterisk = sclerenchyma bands, cat = condensed tannins, co = cortex, cp = collapsed phloem, ds = dark-staining deposits, e = epidermis+wax, ncp = noncollapsed phloem, pa = parenchyma, pe = periderm, r = root, ra = ray, wp = wounded periderm.

Figure 3 Crataegus mexicana and Pittocaulon praecox barks with and without ball moss. A-E. C. mexicana. A) Bark free of ball moss, cross-section. B) Periderm and thick-walled cortical cells. C) Bark-root interface. D) Positive reaction of calcium pectates in parenchyma cells. E) Positive reaction to starch grains. F-H. P. praecox. F) General view. G) Periderm with several layer of phellem. H) Detail of the bark-root interface. Scale bar: A = 300 μm, B, D = 50 μm, C, G = 150 μm, E = 100 μm, F = 500 μm, H = 400 μm. asterisk = mucilage channel, ch = collenchyma, co = cortex, cp = collapsed phloem, ncp = noncollapsed phloem, pd = phelloderm, pe = periderm, ph = phellem, r = root, sc = sclerenchyma.

Figure 4 Lipidic substance developed in the bark-root interface, transverse sections. A, B) Crataegus mexicana. C, D) Pittocaulon praecox. Scale bar: A, B = 100 μm, C = 200 μm, D = 50 μm. Arrow = hyphae, r = root.
Bark anatomical changes in the bark-root interface. In contact with the roots of Tillandsia recurvata, Parkinsonia praecox showed a wounded periderm, 300-400 µm thick (Figure 2B), developed by the most superficial cortical cells, underneath the epidermis (Figure 2B). The wounded periderm had radially expanded cells with thin walls and wide lumina, occluded with dark-staining deposits (Figure 2C). Neither the epidermal papillose cells nor the epicuticular wax layer was observed where the ball moss roots were attached (Figure 2B). The Sudan IV reaction was positive for lipids (Table 3). In Prosopis laevigata the peridermal cells lost their radial organization and a wounded periderm was developed by the cortical cells (Figure 2E). Some collapsed cells between the wounded periderm and the original periderm were observed (Figure 2F). In the collapsed phloem, there were groups of cells with abundant intercellular spaces and dark-staining deposits occluding their cellular lumen (Figure 2G). In some cases damage extended deeper, modifying secondary xylem. In Crataegus mexicana, few phellem layers lost their radial arrangement, but no other structural change was distinguished (Figure 3C). Finally, in Pittocaulon praecox no structural changes were observed (Figure 3H). The roots of ball moss were confined to the surface of the phellem. However, in some cases, roots penetrated into phellem layers, separating them radially (Figure 3H).
Statistical analysis. The multivariate analysis showed that the first two dimensions explained 67.75 % of the total inertia (variance, Table 4). More than 47 % of the total variance was explained by the first dimension (Table 4), therefore only two dimensions were selected (Figure 5). The plot shows that particular host species, with and without ball moss, are separated according to the histo-chemical and anatomical characteristics. Both, Parkinsonia praecox and Prosopis laevigata individuals with and without ball moss were clearly separated across the first two dimensions. In contrast, Crataegus mexicana and Pittocaulon praecox individuals, with and without the epiphyte are barely separated from each other (Figure 5). Groups of Parkinsonia praecox and Prosopis laevigata were distinctly divided because of the evident structural and chemical changes possibly caused by the presence of ball moss. Among the variables reflecting these differences are occurrence of condensed and hydrolyzable tannins, reduction of lignin, presence of a lipidic substance, increase in bark thickness, and in number of phelloderm and phellem cell layers (Table 5, Figure 5). The variables wounded periderm, collapsed phellem cells and texture were weakly related to the presence of ball moss (Table 5). Other characters, such as calcium pectates, rhytidome, and crystals in the outer cortex are some of the host specific traits that were not modified by the presence of ball moss.
Table 4 Singular values, principal inertia, Chi-square, and percentage of variance of coordinate analysis for bark changes in the bark-ball moss interface.


Figure 5 Ordination plot grouping the four host species studied according the characters considered (those with the highest values of partial inertia, Table 5); based on 69 bark samples. Full symbols represent hosts individuals with ball moss and empty symbols represent those without ball moss; symbol thickness represents the number of host individuals: slender = 1, thick = 2, thicker = 3. Triangles = Crataegus mexicana, Squares = Parkinsonia praecox, Diamonds = Pittocaulon praecox, Circles = Prosopis laevigata. Abbreviations: condtann = Condensed tannins, lipidicsubs = Lipidic substance, nuphelle = Number of phellem layers, thick = Bark thickness, hydrotann = Hydrolyzable tannins, nuphello = Number of phelloderm layers, lignin = Lignin.
Discussion
The most important differences of the bark-root interface to the bark alone in all studied hosts were the occurrence of a lipidic substance (Table 3, Figure 4) and some structural or chemical modifications. Structural and chemical differences were evident in the legumes bark (Figure 5), in other cases, a chemical or structural (anatomical) protection was intrinsic to each host species, and these differences were observed in the ordination.
Bark morphology. It is thought that rough surfaces on branches favor germination and anchorage of ball moss seedlings because smooth surfaces retain less water and nutrients from rain (Catling & Lefkovitch 1989, García-Suárez et al. 2003). However, our observations agreed with those of Benzing (1990) and Wagner et al. (2015), who state that surface does not always has a decisive effect on epiphyte anchoring. The studied species, independently of their rough (Crataegus mexicana, Parkinsonia praecox and Prosopis laevigata) or smooth (Pittocaulon praecox) barks, hosted an elevated number of ball moss individuals. Moreover, Escutia-Sánchez (2009) mentioned that Pittocaulon praecox can also host an elevated number of ball moss as a result of disturbance. Some authors have noticed that due to global warming, populations of Tillandsia recurvata are increasing (Caldiz et al. 1993, Guevara-Escobar et al. 2011). Since no specific relationship exists between bark texture and epiphyte anchoring; we assume that ball moss and other Tillandsia species may be able to interact with many different hosts and expand to other habits effectively as epiphytic weeds (Claver et al. 1983, Caldiz et al. 1993). However, many other factors such as host specificity or habitat filtering may have an effect on anchoring and establishment (Wagner et al. 2015).
Anatomy and chemistry of barks without ball moss. In the host species, some differences in bark structure are related to their taxonomic group. Branches of the four host species had non-collapsed phloem, collapsed phloem, and cortex. A periderm was observed in three host species, while the other one, Prosopis laevigata develops a rhytidome. Instead of a typical periderm, Parkinsonia praecox has a multiple epidermis covered by a thick epicuticular wax layer. Both, P. laevigata and P. praecox (both Fabaceae) have abundant prismatic crystals, dilated rays, and sclerenchyma bands (Roth 1981). Anatomical differences between these legumes (Table 2) included the absence of sclerenchyma in the collapsed phloem and a multiple epidermis (sensu Roth 1981) in P. praecox, and a typical periderm, rhytidome and sclereids in the collapsed phloem in P. laevigata. Independently of the strong differences in outer bark, both legumes had similar anatomical and histochemical changes as a consequence of the presence of ball moss.
Pittocaulon praecox (Asteraceae) and Crateagus mexicana (Rosaceae) barks also showed features typical of their taxonomic group. Pittocaulon praecox had a wide phellem (> 20 layers thick), a wide cortex with numerous canals, and scanty secondary phloem. The absence of sclerenchyma in its cortex and the presence of collenchyma beneath the periderm agreed with the observations of Olson (2005) for P. praecox. Parenchymatous barks without sclerenchyma are interpreted as adaptations for water accumulation (Roth 1981, Olson 2005). Crataegus mexicana shares with other Maloideae the presence of phloem parenchyma in bands, wavy phloem rays, thicker periclinal walls in phellem, and tanniniferous deposits (Donghua & Xinzeng 1993).
Chemical compounds such as starch grains in cortical cells and phloem rays, lignin in fibers, and suberin in phellem cells, were present in the four host species studied. A strong reaction to hydrolysable and condensed tannins and calcium pectates in the phellem and in some cortical layers of Crataegus mexicana, indicated that these compounds were synthesized by the plant. Hence, they were interpreted as constitutive (passive) chemical barriers observed even without epiphytes.
Anatomy and chemistry of the barks with ball moss. The establishment of epiphytes caused structural modifications and chemical differentiation among the studied host species. The legumes showed the strongest structural and histochemical changes in response to the interaction with ball moss, as confirmed by the multivariate analysis. The anatomical changes in the legumes support previous observations of wounded periderm development that isolates the most internal cortical cells as a response to damage (Páez-Gerardo et al. 2005, Aguilar-Rodríguez et al. 2007). Biggs et al. (1984) recognized the development of a wounded periderm after mechanical damage or the action of a pathogen. This response is well documented in studies where barks are infected by fungi and other microorganisms and is known as compartmentalization (Bramble 1936, Biggs et al. 1984, Best et al. 2004). Our results showed that the strongest modifications beyond bark, reaching the secondary xylem, occurred only in Prosopis laevigata as it has been observed by Aguilar-Rodríguez et al. (2007). This is in agreement with Ruinen (1953) who mentions that epiphytic ferns and orchids can damage hosts by occluding vessels in xylem and by necrosis in vascular and cortical tissues. The dark-staining deposits observed in the injured areas of P. laevigata and Parkinsonia praecox corresponded to condensed tannins. These compounds are usually produced as a chemical response to predators (Zucker 1983, Franceschi et al. 2005) as an active response of the plant (Shain 1995). In P. laevigata and P. praecox these condensed tannins likely act to prevent or stop the entry of pathogens. In contrast, lignin was not accumulated at any injured tissue in our study, unlike what has been reported in other studies, in which lignin is present in the injured and infected cortex by pathogens (Biggs & Peterson 1990).
Pittocaulon praecox and Crataegus mexicana have a single periderm, but differences in their bark organization and chemical compounds seemed to be important to avoid changes under the presence of epiphytes. For example, in Pittocaulon praecox, some of the most superficial phellem layers occasionally break in order to hold the roots of ball moss, and all adjacent cells collapse. The presence of numerous phellem layers probably represents a defense mechanism that inhibits (at first) any possible effect that ball moss could have on the bark. The absence of structural alterations in the bark of C. mexicana was related to the chemical compounds of its bark, which are abundant before ball moss establishes. The tannic acid-ferric chloride test for calcium pectates had a positive and intense reaction (Figure 3D) and could be related to a high proportion of amylopectin (Ruzin 1999). It is well-known that calcium pectate is a component of the middle lamina and prevents cellular membrane damage. In addition, an intense reaction also occurred with the ferrous sulfate test, indicating the presence of hydrolysable tannins in this host species, which likely act as a chemical defense of plants (Vivanco et al. 2005). The high natural occurrence of pectin and hydrolysable tannin contents may be responsible for protecting C. mexicana in the presence of epiphytes, since it is a passive chemical barrier in the bark (Shain 1995).
The interface. We found a lipidic substance present in the bark-root interface in all hosts studied. Türkowsky & López (1983) mentioned a gummy substance in roots of other Tillandsia species which function they interpreted as attaching the roots to the substrate. However, this function has not been tested, to support these conclusions. Moreover, we did not detect a gummy substance; according to our histochemical tests, it is a lipidic substance; however, we do not know if this substance is produced by the Tillandsia roots or the host species barks. Further research is needed to examine whether the roots of ball moss or the hosts are responsible for the occurrence of this lipidic substance that may contribute to their interaction.
We conclude that bark responses to ball moss establishment in the studied host species are strategies related to the taxonomic group they belong to, as it has been demonstrated in conifers reacting against different pests (Franceschi et al. 2005). They can be divided into mechanical and chemical. Both legumes, responded to ball moss developing active mechanical and chemical barriers. In contrast, in Pittocaulon praecox, the phellem represents a passive (intrinsic) mechanical barrier that prevents bark modifications due to the establishment of ball moss and it could be considered as a structural constitutive defense mechanism; whereas in Crataegus mexicana, the passive (intrinsic) barrier is chemical and corresponds to a chemical constitutive defense mechanism. When structural changes exist, these are interpreted as bark injuries. Hence we could suggest that an excessive number of epiphytes may cause branch or even the individual’s death in the host species studied. Investigating the anatomical bark characteristics will allow the identification of woody species more susceptible to the establishment of ball moss, this is especially important since ball moss populations are suggested to increase due to climate change.