Services on Demand
Journal
Article
Indicators
Related links
Share
Tecnología y ciencias del agua
On-line version ISSN 2007-2422
Tecnol. cienc. agua vol.10 n.5 Jiutepec Sep./Oct. 2019 Epub Feb 15, 2020
https://doi.org/10.24850/j-tyca-2019-05-11
Articles
Green roofs for comprehensive water management: Case study in Chapinero, Colombia
1Universidad Católica de Colombia, Bogotá, Colombia, ocontreras25@ucatolica.edu.co
2Universidad Católica de Colombia, Bogotá, Colombia, pavillegas@ucatolica.edu.co
The city of Bogotá (Colombia) has been immersed in flooding problems caused by rainwater, and issues from the increase in average temperature and air pollution. Green technologies are a mechanism that can mitigate these problems. This research analyzes the behavior of urban flooding in the northern sector of the Chapinero locality when replacing the existing roofs with green roofs through computer modeling. Additionally, its develops a green roof prototype, which analyzes the quality of rainwater before and after being filtered through different substrates (mixtures of garden soil, rice husk, humus, sand and/or sawdust) and plants (Sedum, Asparagus plumosus and Soleirolia), in order to determine the materials with which the conditions of rainwater quality are controlled once it filters through said roofs. The increase in the amount of green roofs contributes to mitigating flooding, as well as to the capacity of the area’s storm drain pipes to transport rainwater. In turn, laboratory results indicate that sawdust is 46% more efficient than the other analyzed materials in controlling the rainwater’s pH. Sedum plants demonstrated a satisfactory resistance to the conditions of frequent rainfall. Using green technologies not only decreases urban vulnerability to flooding, but also creates mechanisms for comprehensive water management.
Keywords: rainwater quality; integral management of water resources; flood; modeling; precipitation; simulation; green roof; green technologies
La ciudad de Bogotá, Colombia, se ha visto inmersa en problemas de inundaciones por empozamientos de agua pluvial y aumento tanto en la temperatura promedio como en la contaminación del aire. Las tecnologías verdes representan un mecanismo para la mitigación de tales problemas. Esta investigación analiza el comportamiento de la inundación urbana en el sector norte de la localidad de Chapinero, al sustituir las cubiertas existentes por techos verdes mediante modelación computacional. Asimismo, se construye un prototipo de techo verde en donde se analiza la calidad del agua de lluvia antes y después de filtrarse por diferentes sustratos (mezclas de tierra de jardín y cascarilla de arroz, humus, arena y/o aserrín), y plantas (Sedum, Asparagus plumosus y Soleirolia), con el fin de determinar los materiales con los cuales se controlen las condiciones de calidad de agua de lluvia una vez se filtre a través dichas cubiertas. El aumento en la cantidad de techos verdes contribuye a la atenuación tanto de las inundaciones como de la capacidad de transporte de agua de lluvia de los ductos del alcantarillado pluvial de la zona. Por otro lado, los resultados del laboratorio indican que el aserrín es un 46% más eficiente que los demás materiales analizados en el control del pH de la precipitación. Las plantas Sedum demostraron una resistencia satisfactoria a un régimen frecuente de lluvia. El uso de tecnologías verdes no sólo disminuye la vulnerabilidad urbana frente a eventos de inundación sino también crea mecanismos para la gestión integral del recurso hídrico.
Palabras clave: calidad del agua pluvial; gestión integral del recurso hídrico; inundación; modelación; precipitación; simulación; techo verde; tecnologías verdes
Introduction
Bogotá, the capital of Colombia, is a growing city, where a population increase of around 24% is expected by 2020 (Secretaría de Hacienda, 2017). Its geographical development is rapidly increasing (Peña-Guzmán, 2016). In 2015, 8.2 million square meters were recorded under construction (Herrera, 2016), with construction being one of the sectors that contributes the most to the city’s economy. The increase in the construction industry is directly related to the capacity for rainwater drainage, given the increase in the waterproof surfaces in the city.
Currently, localities such as Antonio Nariño, Chapinero, Engativá, Kennedy, Fontibón and Usaquén (Almanza, 2017) are vulnerable to floods due to the accumulation of garbage (Departamento de Prevención y Atención de Emergencias, 2012) and subsequent saturation of storm drain pipes. These problems are caused by the increase in rainfall that the pipes transport (Granada & Secretaría Distrital de Ambiente, 2008) as a result of the expansion of impermeable areas in the city, which channel rainwater directly to the storm drains. Twenty-two households in the Fragua neighborhood of the Antonio Nariño locality in Bogotá were specifically affected by belongings and domestic property losses (El Tiempo, 2015. Additionally, there have been major traffic jams that obstruct the flow of vehicles to the south of the city (Radio Cadena Nacional, 2017) and a containment wall in a residential area in Rosales, Chapinero locality collapsed (El Espectador, 2016). Floods have also been reported in the sector of Simón Bolívar Park, in the neighborhoods of Las Ferias, La Granja and Barrios Unidos and around Boyacá Avenue (El Tiempo, 2016). The described effects show that society cannot keep waiting for intervention in water resource management (Bernal-Pedraza, 2010). Otherwise, there could be more serious consequences.
The information some citizens have on topics related to water, the environment and the problems that result in inefficient resource management in Bogotá is limited. Therefore, it is important to raise awareness on preservation with water saving programs or automated systems to help prevent its waste (López, García, Gameros, & De la Rocha, 2015). In the city of Mexicali, Mexico, it is expected that in a period of three to five years, the water supply will reduce by around 30% (López et al., 2015). In the plains in the north of China, for example, water tables are decreasing. This resource used to produce 25% of the country’s cereal crops (Fernández-Colón, 2009). Additionally, in North Africa, the Egyptian Ministry of Environment recognized that vast areas of the northern area of the Nile Delta, the largest and most important agricultural region in this country, is already suffering from two major dangerous consequences: salinization and flooding (Kamal, 2016). In Italy, more than 50% of water quality samples from the rivers do not achieve a good quality (Conte et al., 2012). These studies and the current situation of Bogotá show that the crisis is not only due to trying to meet demands with scarce resources, but also to deficient comprehensive management that incorporates rational use of water (Rivera & Aguila, 2015). The findings show that the incidence of events such as floods and droughts will gradually become more frequent in different parts of the world.
Therefore, urban floods related to deficiency in the maintenance of the drainage systems in Colombian cities are increasingly more frequent (Banco Mundial, 2012). Therefore, this research presents an alternative to the urban problem in the sphere of integrated water management based on the analysis of a green roof prototype for rainwater management. A strategy that has been used globally and nationally for using water is comprehensive rainwater management. This kind of strategy mainly seeks to reduce run-off, control the contamination of rainwater and expand the rainwater infrastructure (Peña-Guzmán, 2016).
Additionally, the city needs to reduce pollution and the temperature, factors that green technologies are capable of counteracting. The case study analyzes the city of Bogotá, where floods have affected the city due to intense rainfall in recent years (Niño-Fierro, 2012). The efficiency of this technology in the northern area of the Chapinero locality, where flooding occurs during the rainy seasons, is analyzed. In addition, incorporating new mechanisms for controlling rainwater quality is proposed, aiming to reuse water in domestic chores.
Developing sustainable technology is proposed through studying a prototype, understood as a real phase of the design through developing and executing experimental trials (Kroes, 2012). This research hopes to help empower the benefits of green roofs for society, and for it to be used as an example for cities that have problems such as those that occur in some communities in the city of Bogotá.
The concept of sustainability is one of the essential approaches of this research, given that it is the current direction of water management (Martínez-Austria & Vargas-Hidalgo, 2016). Green roofs are proposed as a sustainable technology because they have the capacity to absorb rainwater, reducing the risk of floods from precipitation. For example, in Auckland, New Zealand, 66% of rainwater was reported to have been retained in a large green roof (Nawaz, McDonald, & Postoyko, 2015). The use of rainwater to replace potable water for certain uses (flushing toilets, watering plants or washing clothes) can be taken into account as an alternative to improve efficiency in using water (López-Patiño, Martínez-Solano, Fuertes-Miquel, & López-Jiménez, 2011).
Green roofs are an emerging technology in the city. These roofs reduce the probability of flooding (Oviedo & Torres, 2014), since their capacity to take in rainwater gradually reduces the rate of filtration to rainwater drains, preventing the pipes from becoming saturated and subsequently silting. A green roofs vegetation's carbon dioxide and oxygen exchange produces sufficient oxygen for one year for one person (Kravits, 2007). Likewise, a surface of 15 m x 15 m is sufficient to supply oxygen to a family of four people every day (Psencik & Heller, 2012). A success story in counteracting this problem took place in Hong Kong, where on a sunny day, a large green roof reduces the carbon dioxide concentration by up to 2% (Zielinski, Carlos, Paternina, & Marta, 2012). Green roofs clearly benefit a city with pollution problems. Additionally, they decrease the temperature in urban areas (Yan, 2011). In the city of Buenos Aires, for example, an increase in the average maximum temperatures is forecast from 0.6 ºC to more than 2 º C in the period from 2020 to 2029 (Rosatto, Botta, Becerra, Tardito, & María, 2016). Circumstances like these increase the importance of implementing these technologies as a mitigation mechanism for the problems.
This document starts with the conceptual framework, which includes general information about green roofs, and the topics are contextualized related to the benefits, rainwater quality, structural impact on a building, the private and public cost, and the LEED (Leadership in Energy and Environmental Design) certifications that can be granted to systems with this kind of roof. Subsequently, the results of the work with the community are presented, conducting a survey on the residents of the study area in September 2015. This demonstrates the importance of implementing green roofs as an alternative to prevent flooding in the sector. Using the Storm Water Management Model (SWMM) software, the study models the features of the current roofs on the area’s buildings, the inspection chambers and the pipelines for the area’s rainwater drainage, and the precipitation of the area. Traditional roofs are replaced with green roofs in order to compare the flooding behavior with the two kinds of roofs. Implementing this technology is a new method for traditional rainwater management, which helps “make cities and human settlements inclusive, safe, resilient and sustainable.” (Organización de las Naciones Unidas, 2015) These models' results are analyzed and work as a reference for developing a green roof prototype with different kinds of plants and combinations of substrates. This design works as a basis to compare the quality of rainwater and water filtered by the green roof for its use in households.
What are green roofs?
A green roof is a construction system that sustainably maintains a plant landscape on the roof of a building (Secretaría Distrital de Ambiente, 2011). Green technologies vary in their characteristics depending on the implemented substrate and plants. Usually, it is comprised in ascending order starting with a waterproofing membrane, which aims to prevent contact between the water and roof support because pathogens could develop that affect the structural integrity of the building. This is followed by the drainage layer, which channels the surplus rainwater and prevents the passage of the material of the roof’s growth medium. Next, there is the filter layer, which prevents the roots from reaching the roof structure. The substrate is the growth medium of the vegetation and works as a nutritious material that stores water and must have a sufficient volume of air in pores to give the roots the possibility attach themselves (Minke, 1992). Finally, the plant layer provides a vegetation cover (see Figure 1), and given the different weather and chemical conditions the roof’s plants are exposed to (Secretaría Distrital de Ambiente, 2011), the vegetation characteristics must be analyzed in order to ensure the maintenance and sustainability of the roof.
Planting vegetation on the roof not only beautifies the environment (Sun, Lin, Sung, Ou, & Lu, 2012), but it can also provide the benefits inherent to green roofs. Prior research has demonstrated the potential for some items to improve the conditions of these roofs to increase their effects. For example, using moss can improve the performance of rainwater management and the temperature of green roofs (Anderson, Lambrinos, & Schroll, 2010).
Experiments with different green roof substrates have reached similar conclusions. The slower the rate of run-off, the higher the concentrations of all the components studied in the run-off water of the green roofs (Teemusk & Mander, 2011). Additionally, the quality of the run-off from green roofs depends on the differences in the structure of the roof’s layers (Hashemi, Mahmud, & Ashraf, 2015).
To date, the benefits of green roofs have been named, which are currently still under study. Implementing this technology is connected to the conditions of the buildings, where the structure plays an important role in the limitations of installing a green roof, the weight of the substrates and the planting. For example, they must be considered with respect to the load capacity of the roof (Wilkinson et al., 2015). If the building does not meet the structural requirements to bear the weight, adaptations need to be made for the roof’s implementation. The inclination of the roof is an important factor, because a strong slope in areas of heavy precipitation could lead to erosion and damage to the vegetation. Additionally, wind and solar radiation can affect evaporation and, consequently, are important to consider in selecting the plants (Minke, 1992).
It is worth noting that implementing these roofs is recommended in all the properties of a community that has the problems described above, given that the additional cost of a green roof is a private cost and in turn, many of its benefits are public and external to the construction itself (Blackhurst, Hendrickson, Asce, Matthews, & Asce, 2010). Therefore, the cooperation of the community is required to have technologies such as green roofs adapted to the environment and fulfill their functions. In Switzerland, it is required by law for every new construction is offset with green areas (Kravits, 2007). In Bogotá, every new public construction must include sustainable technology in its design (Consejo de Bogotá, 2009).
Buildings that have appropriate water management, consume less energy and reduce greenhouse gases can receive the LEED certification. These sustainability strategies are changing the way in which buildings and communities are planned (Green Building Consul, 2017). This helps improve green technologies that counteract the environmental problems that are currently being seen throughout the world. For example, Germany is the world leader in implementing green roofs, where 10% of buildings use this technology (Saadatian et al., 2013). The increase in commercial value is a determining factor in incorporating green roofs. Tokyo has started to install green roofs to increase property value per square meter (Zielinski et al., 2012). Similarly, the USA records an increase in the value of buildings with green roofs of between 6% and 15% (Johnston & Newton, 2004). In turn, in the private sector, the Novartis building in Bogotá can be highlighted, which was one of the pioneers in using green roofs as an ecological emblem to encourage this model (Hoyos-Giraldo, 2014).
Purpose, scope and limitations
This research aims to analyze a mechanism that helps to gradually reduce cases of urban flooding and contribute to the comprehensive water management of in the northern area of the Chapinero locality. This will be done through two strategies comprised of computer modeling, which aims to analyze the changes in urban flooding by implementing green roofs, and physical modeling, which aims to analyze the behavior of the characteristics of rainwater quality and retention with different green roof alternatives.
This work is an initial study that aims to achieve results related to flooding behavior in a sector susceptible to this urban problem in Bogotá, in order to observe the impact of the sustainable technology of green roofs on the flooding problems in a community. In addition, variations in rainwater quality and the retention capacity of the substrate are expected to be found in order to propose an alternative that contributes to comprehensive water management.
Moreover, the accuracy of the model is affected by the lack of accurate information with respect to leaks, incorrect drainage connections in the storm drain system or garbage problems. During the period in which precipitation simulations were carried out, there was no rainfall in the area where the laboratory that developed the experimental process was located. Therefore, the acidity of the water used for the simulations increased.
Characterization of the study area
There are urban flooding problems in various areas of Bogotá as a result of saturation of the storm drain pipes or the overflow of surrounding channels or rivers. Green roofs have the capacity to counteract said accumulations of water. After analyzing the sectors of Bogotá that suffer from floods, using a map of flooding spots prepared by the District Institute of Risk and Climate Change Management (IDIGER, for the Spanish original), in 2015, the area between Calle 94 and Calle 106, the Nueva Granada Military School and Carrera 15 Avenue was selected.
Parks and green areas act a natural rainwater filter. This is a principle to implement with the installation of green roofs. Parks and green areas are scarce in this part of the city, which means there are many impermeable surfaces that channel the run-off to the storm drains. In Figure 2, you can see the location of the affected area chosen for analysis, from right to left with Colombia, Bogotá, the locality of Chapinero and the north of the Chapinero locality.
A survey was conducted on September 12 and 13, 2015, on 40 citizens of the sector to find out the information that the surveyed citizens have with respect to the floods that occur in the locality and the mechanisms to mitigate the problem. The survey included questions such as: “How often is there flooding in this sector due to rainfall?”, “Do you know what a green roof is?”, or “Would you be willing to install a green roof on your property to counteract the levels of flooding and increase the environmental quality of Bogotá?”.
The residents of the sector are aware of the floods generated during the rainy seasons, normally between October, November and December. They are unaware of the causes of the flooding or of any sustainable mechanism to counteract this problem. The benefits and disadvantages of implementing a green roof were explained to the surveyed citizens, who were enthusiastic about the idea of implementing this technology in the area. Figure 3 shows the results of the seventh question in the survey, where the majority of the residents said they would be willing to install a green roof on their property.
The community’s interest in solving the flooding problem in the sector through sustainable technology is shown, which not only counteracts the levels of flooding, but also contributes to improving the environment and works as an example for other communities that have similar problems. The city of Bogotá recorded levels of 877 mm, 506.5 mm and 872.8 mm of precipitation for 2014, 2015 and 2016, respectively (Observatorio Ambiental de Bogotá, 2016), with the last months of the year having the highest rainfall.
Methodology
This research consists of two phases. The first presents the conceptual model of the problem being studied, where the flooding conditions in the city of Bogotá are assessed, locating the best affected area for the installation of green roofs. Subsequently, the computing model carried out in the SWMM software is described from the modeling of each element (precipitation, conventional roofs, green roofs and rainwater drainage system) and the relationship between them (precipitation - roof, relationship between the adjacent roofs and roof - rainwater drainage system), consolidating the first phase of the research. The second phase consists of a simulation through a physical model, which analyzes the rainwater retention and quality conditions before and after being filtered by different compositions of green roofs and a sand filter.
Conceptual model
Figure 4 explains the behavior of rainwater in traditional roofs. The image on the left presents the precipitation (a) that generates an elevated rate of run-off through the traditional roofs (b). This water flow is directed to the street drains and finally sent to the storm drain pipes. As the pipes do not have the capacity to drain said volume, the system collapses and results in a flood (d). In turn, the image on the right shows the behavior of precipitation when penetrating a green roof (c). This reduces the rate of rainwater run-off, alleviating the capacity for transporting the liquid through the pipelines, and preventing the saturation of the pipes, subsequent flooding and storage of water in internal reservoirs (e) of the building.
Computer model
Precipitation reaches the building roofs by gravity. The number of millimeters of precipitation is estimated through the intensity-duration-frequency curve (IDF curve) of the study area. Through Equation 1, the scale of the rainfall intensity is measured with different durations (1 hour, 2 hours, 3 hours, 4 hours and 5 hours) and periods of return (3 years, 5 years, 10 years, 25 years, 50 years and 100 years):
Where i = intensity in millimeters per hour; C1 y C2 = parameters of the most suitable equation for the method of the square minimums; X0 = duration in minutes; d = duration of precipitation in minutes; C1, C2, y X0 are values extracted from the coefficients of the IDF curves calculated by the Institute of Applied Hydrology of Empresa de Acueducto y Alcantarillado de Bogotá. Subsequently, a hyetograph is created with the duration of the precipitation and its corresponding intensity.
Conventional roofs as well as green roofs are modeled as sub-basins to assign a treatment to each roof for precipitation, whether in the form of run-off (conventional roof) or infiltration (green roof). Modeling these elements depends on the area, the slope and the width of the roof, which is obtained through an analysis of maps with the Google Earth tool, understanding the width as the characteristic dimension of the water flow due to run-off. The difference that exists between the modeling of the conventional roof and the green roof lies in the impermeable area and infiltration. For the conventional roof, the impermeable area is the total area, with a Manning coefficient of 0.012 (Environmental Protection Agency, Water Supply and Water Resourses Division, Efr, & M. Inc., 2004), because of the relatively smooth materials that do not allow infiltration. In turn, for the green roof, there is no impermeable area, because the installation of the green roof is assumed in the whole area available, with a Manning’s n coefficient of 0.24 (Environmental Protection Agency et al., 2004) due to the dense plant layer. Finally, characteristic values of the substrate are assigned with respect to the height of suction, conductivity and initial deficit to consider the infiltration action of precipitation in the green roof.
For modeling the rainwater drainage system, the guidelines of lengths, levels and materials of the plans granted by Empresa de Acueducto y Alcantarillado de Bogotá (EAAB) were followed. The drainage of the area is comprised of PVC pipes as well as a stretch of box culvert. Additionally, characteristics were assigned related to the shape (circular, box culvert), depth, length and roughness (PVC, concrete). There is an inspection chamber (manhole) in the joint of each duct that receives water from the roofs.
Table 1 summarizes the characteristics of the roofs, drainage and precipitation implemented for modeling in the SWMM.
Table 1 Elements of the modeling in SWMM.
Modeling in SWMM | |||||
---|---|---|---|---|---|
Adjacent | Roofs | Drainage System | Rain | ||
Sub-adjacent | Green roofs | Conventional roofs | Pipes | Box culvert | Rain meter |
Characteristics | Discharge Area Slope Width Permeability coefficient Flow between areas Run-off |
Discharge Area Slope Width Impermeable coefficient Flow between areas Infiltration |
Shape Depth Length Manning coefficient |
Shape Depth Length Manning coefficient |
Intensity |
The existing relationship between the roofs, drainage system and rainwater is described below. Figure 5 presents a systemization of the model carried out. In the first instance, the rain meter is the idealization of the precipitation process. Said element assigns the aforementioned hyetograph to each one of the modeled areas to simulate contact between rain and the surface of the roofs in the study area. Once the precipitation is in the areas, it runs off the roof (case: conventional roof) or is infiltrated into the roof (case: green roof). Two models: the first without considering green roofs and the second taking them into account. Each area that the roof covers is connected to an inspection chamber through a discharge line, which channels the precipitation from the roof to the chamber. Subsequently, the rainwater is transported by gravity through the pipes to the discharge points at the end of the drainage system’s lines.
Green roof prototype
The prototype is comprised of an unwoven polypropylene geotextile in the roof’s drainage layer. Humus, rice husk, sawdust and sand are used as an alternative substrate material. The Sedum, Asparagus plumosus and Soleirolia species are used in the plant layer. Additionally, a rain simulator is designed, which provides rainwater to the prototype, extracting the liquid from an underground tank through a connection with a centrifuge pump. Likewise, a sand filter is designed, which the rainwater passes through once it is filtered by the green roof’s substrate. Figure 6 shows an overhead view of the prototype with its provided number in order to understand the location of each combination of plants and substrates present in Table 2.
Table 2 Composición de los cajones del prototipo de techo verde.
Drawer | 1 | 2 | 3 | 4 |
---|---|---|---|---|
Plant | Sedum 1 | Sedum 2 |
Asparagus plumosus |
Soleirolia |
Material alternativo |
Humus (10%) |
Rice husk (10%) | Sawdust (10%) |
Sand (10%) |
Drawer | 5 | 6 | 7 | 8 |
Plant | Sedum 1 | Sedum 2 |
Asparagus plumosus |
Soleirolia |
Alternative material |
Humus (5%) |
Rice husk (5) | Sawdust (5%) | Sand (5%) |
Finally, the rainwater absorbed by the green roof’s substrate passes through a sand filter, comprised in descending order of activated charcoal (10 cm), washed sand (10 cm), fine gravel (6 cm) and thick gravel (5 cm). The purpose of the filter is to reduce the turbidity with which rainwater is extracted from the green roof.
Figure 7 presents the green roof prototype (a). All of the substrates are comprised of a combination of garden soil and alternative materials of 10% and 5% of the weight of the soil in order to obtain a 10 cm thick substrate. Additionally, it shows the sand filter (b) implemented in the process of improving the rainwater quality.
The trials were carried out between January 30 and February 24, 2017.
The prototype trials include the simulation of five characteristic precipitation levels of Bogotá (30.6 , 48 , 64.56 , 86.7 and 128.4 mm, respectively), which are within the range of precipitation levels recorded in the Colombian capital, over each one of the eight green roof sections that the prototype contains. The supply of rainwater is stopped half an hour after the start of the simulation and the exit flow is measured every five minutes for another hour, recording the amount of water collected in said time through a rain meter. Additionally, the characteristics of water quality (conductivity, resistivity, suspended solids, salinity, temperature, pH, oxygen and turbidity) are measured before and after being filtered by the green roof substrate.
Results
The results obtained in the SWMM program as well as the results obtained from the trials with the green roof prototype are analyzed. These are presented below:
SWMM Modeling of the Affected Area afectación
When comparing the flood areas notified by the residents with the flooded places resulting from the SWMM, the lack of some variables that were not taken into account is acknowledged, such as garbage, incorrect drainage connections or leaks in the system. The behavior of each flooded chamber in the model is analyzed through the comparison of the level of flooding of said chambers with the results of the levels of the same chambers with different percentages of implemented green roofs. Likewise, the saturation of the current pipes is analyzed before and after the replacement of the conventional roofs with green roofs.
The results produced by the SWMM program firstly indicate the flood points for a period of return of three years. Figure 8 compares the flood points reported by the community (small circles) with the flooded areas as a result of the modeling (large circles). Eight areas of flooding are presented as a result of the model. According to the map of Figure 8, a greater amount of floods actually occurs. In the modeling carried out, only the parameters described in Table 1 were taken into account, but the existence of variables such as leaks, incorrect drainage connections or the presence of garbage, which increase urban flooding, have repercussions on the accuracy of the model and reality.
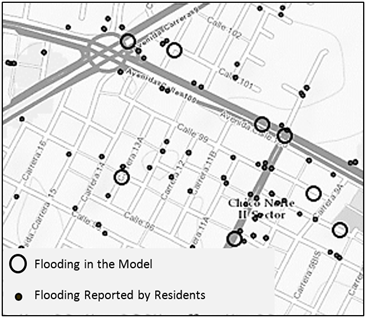
Figure 8 Comparison between the Actual Flooded Areas (points) and the Areas of Flooding according to the Model (circles).
Additionally, the following analysis is conducted. Two critical chambers are taken into account according to the results of the previous simulation (chamber 90 and chamber 146). An inspection chamber has contributions from different areas of roofs. The analysis consists of replacing a certain percentage of conventional roofs with green roofs and observing the level of flooding. Subsequently, the percentage of green roofs increases until it mitigates the flooding in the chambers.
Figure 9 shows the ratio between the amount of green roofs implemented and the level of flooding in the chamber. The roofs that directly contribute rainwater to the chambers are replaced. Chamber 90 had an 88.9% reduction in flooding when replacing all of the roofs that had a direct impact on the chamber, for a total replacement of 4732.21 m2 of roof area. The decrease in the flooding of this chamber when replacing the roofs that drain rainwater directly onto it is considerable. However, chamber 146 had a 9.5% reduction, maintaining the problem of urban flooding.
In a system of rainwater drainage that works by gravity, the accumulation of rainwater tends to increase at lower levels, because the pipes must have the capacity to maintain the volumes of water that the impermeable areas in the surrounding area contribute as well as the water volumes of the impermeable areas at higher levels. The probability of flooding tends to decrease with the implementation of green roofs not only in the surrounding areas of the area of flooding, but also in areas with a higher level than the one of the areas with the problem.
With the aim to counteract urban flooding, green roofs are deemed an alternative that must involve the whole community. Collaboration and cooperation are the starting point from which work needs to be implemented.
To illustrate the discharge performance of rainwater in the pipes with conventional roofs and green roofs in the model, the rainwater drainage system of the affected area is compared at the time of maximum flooding. In Figure 10, the dark-colored ducts indicate a normal drainage capacity, while the light-colored ducts indicate the saturation of the stretch.
Green roof prototype
In terms of the green roof prototype, the variation in water quality is analyzed depending on the type of plant used and the alternative materials mixed with garden soil, which comprises the substrate of each section. The rainwater retention capacity of the prototype’s substrates is also analyzed.
The function of the green roof lies in its capacity to retain rainwater as an alternative to reduce the probability of urban flooding from the accumulation of rainwater. This research proposes reusing rainwater in households as a measure for managing water.
When filtering through the substrate of the green roof, the rainwater’s properties are altered, as well as the conditions of the resource’s quality. The indicators that show a clear alteration are conductivity, resistivity, suspended solids and pH.
Figure 11 and Figure 12 present the variation in the conductivity and resistivity of the filtered water, respectively. Five rainwater simulations are carried out in which the properties of the rainwater before entering the green roof and once filtered by the green roof are analyzed.
In drawer 1, comprised of garden soil and 10% humus, it is observed that once filtered through the green roof, the conductivity of the rainwater increases and its resistivity decreases. After carrying our five simulations, the values of both conductivity and resistivity stabilized, achieving a similar value to that of rainwater before being filtered by the green roof.
When rainwater passes through the green roof, it takes in particles of garden soil and humus, which increases its capacity to conduct electricity. Therefore, its conductivity increases and its resistivity decreases. Figure 13 shows the behavior of the suspended solids in both the rainwater and water filtered by the green roof. It shows that in the first simulations, the suspended solids increase in the rainwater, although, as in the first two parameters, after five simulations of precipitation, the values tend to stabilize.
pH is a measure that indicates the level of alkalinity or acidity of water; it is an important characteristic to take into account in consuming drinking water, which must have a pH of “between 6 and 8.5” (Ministerio de Ambiente Vivienda y Desarrollo Territorial, 2000). The results produced indicate that sawdust with the Asparagus plumosus plant and sand with the Soleirolia plant are efficient in controlling the pH of rainwater. However, these plants do not have the characteristics to resist extreme environments.
To supply rainwater to the prototype, a tank is used that stores the precipitation through a pump that pushes the liquid into the compartments. As there was no precipitation during the trial period, the acidity of the stored water increased. Therefore, pH values gradually decreased.
A similarity is shown between the pH of filtered water and the pH of rainwater. This indicates that sawdust (see Figure 14) and sand (see Figure 15) in a greater proportion could efficiently control the pH and could elevate this parameter when filtered by the roof. However, it is possible that when increasing the percentage of sand in the substrate of the roof, its drainage capacity would be affected.
The behavior of water turbidity is due to the sand filter, which was backwashed for the second simulation of precipitation. From this point, the turbidity decreased, because a greater percentage of activated charcoal was included and there was no more backwashing in the rest of the simulations.
Apart from controlling the water conditions, as analyzed, green roofs must also enable the passage of rainwater at a rate that guarantees the lowest amount of run-off generated, because the main reason for adopting green roofs is to counteract the problems of urban flooding.
The alternative materials with the greatest rate of run-off are sand and humus. In turn, rice husk and sawdust obtained high levels of infiltration with respect to the other two studied materials. Figure 16 compares the characteristic performance of rice husk as an infiltration material exposed to a precipitation of 128.4 mm/min.
Drawer 2 (10% rice husk) presents a greater intake of rainwater. Although it could be concluded that a greater capacity of water retention is efficient, the surplus in the intake of the resource could generate internal floods on the roof, which would generate elevated run-off. Therefore, it is considered that Drawer 6 (5% rice husk) presents satisfactory infiltration conditions in the green roof prototype.
Discussion and conclusions
The modeling of the affected area conducted in the SWMM software simulates a flooding process, which is lessened by implementing green roofs. However, there is a greater number of flood incidents reported by the residents in the area than the incidents resulting from the modeling. Garbage, incorrect drainage connections or leaks are parameters that are not taken into account for the model. These elements are potential generators of floods, because they affect the water flow in the ducts or contribute an extra flow to the pipes of the storm drain.
Although green roofs belong to the urban systems of sustainable drainage (SUDS, for the Spanish original), their function to counteract flooding is limited by the inward flow at the point of flooding. As analyzed in the case study of the Chapinero locality, the roofs replaced by green roofs in the surrounding area of the one where the flood occurs are sometimes not sufficient to mitigate this problem. The inflow to the duct needs to be reduced in order to reduce the probability of its saturation. Therefore, in implementing this technology, the participation of structures located at higher levels helps counteract this problem.
When testing different green roof substrates with different precipitation intensities, data on the rainwater quality before and after the filtration process through the green roof were obtained. It was found that after five simulations of precipitation, the substrate changes to a condition where characteristics such as conductivity, resistivity, salinity, suspended solids and oxygen content remain constant after filtering. However, pH tends to decrease. Sawdust and sand as alternative materials were efficient in controlling the pH level of rainwater.
The factors that affect the run-off water quality include the substrate, plants, local sources of pollution, amount of rainfall, fertilization and maintenance, age of the green roof and the physical and chemical properties of the contaminants (Vijayaraghavan, Reddy, & Yun, 2019).
The pH values obtained tend to be in the range of 6.19 to 6.24 for green roofs that included the Asparagus plumosus and Soleirolia plants and the substrates comprised of sand and sawdust, given that they are the alternatives that most efficiently control this indicator compared to the other ones proposed. Other research proposes average pH levels for green roofs with Sedum plants of 6.64 (Todorov, Driscoll, Todorova, & Montesdeoca, 2018) in the city of New York, USA; 6.95 (Buffam, Mitchell, & Durtsche, 2016) in the state of Ohio; 7.05 (Wang, Tian, & Zhao, 2017) in the city of Tianjin, China; and 6.4 (Sultana, Akib, & Ashraf, 2017) in Malaysia. It is worth adding that pH levels also depend on the level of pollution in the city.
The rainwater’s turbidity after being filtered by the substrate tends to increase given the presence of material from the growth medium. A measure to control said parameter is implementing a sand filter, which requires a backwash process after frequent use. It is recommended to use an efficient mechanism for backwashing the filter every time there is precipitation to ensure its effectiveness.
In turn, it was observed in the experimentation of this research that the initial level of conductivity has values of approximately 320 µS/cm for a green roof with humus and Sedum plants, which after five simulations of precipitation, stabilizes at 106.2 µS/cm; a value close to that of the rainwater calculated at 103 µS/cm. In other research, values of around 100.3 µS/cm stand out (Sultana et al., 2017) considering a plant layer of the Plylathus species in Malaysia, of 225 µS/cm (Zhang et al., 2014) in Chongqing, China, for a roof with Sedum plants, and of 152 µS/cm (Buffam et al., 2016) in the state of Ohio, USA.
Green roofs have the capacity to retain rainwater in their substrate. When they come into contact with water, both humus and sand create a type of impermeable seal that obstructs the flow of water, slowing down its passage through the roof. Alternatively, rice husk and sawdust have high levels of infiltration with respect to humus and sand. Rice husk generates 33% less run-off than sawdust in precipitations of 128.4 mm/min.
The retention characteristics widely depend on the thickness and the materials used for the growth medium and on the level of precipitation in the place. The substrate that has rice husk as an alternative material presents variations in terms of the retention percentage, which ranges between 23% (drawer 2) and 42% (drawer 6). Different studies have been found that analyze the retention capacity of green roofs, producing a wide range of percentages, from 12% to 17% for a green roof with a substrate thickness of 15 cm, Sedum plants and monitored in the city of Portland, Oregon, USA (Spolek, 2008); 51% for a substrate of 20 cm analyzed in the city of Genova, Italy (Palla, Gnecco, & Lanza, 2010); 65.7% in a growth medium of 17 cm and biomass plants analyzed in the city of Manchester, UK ( Speak, Rothwell, Lindley, & Smith, 2013); and 76.4 with a 15 cm substrate and Sedum plants analyzed in the city of Beijing, China (Yang, Li, Sun, & Ni, 2015).
Acknowledgments
The author thanks the Administrative Department of Science, Technology and Innovation (COLCIENCIAS, for the Spanish original) for its trust and support during the six months of the research as part of call for proposals no. 743 “Young Researchers and Innovators 2016”; the residents of the localities of Usaquén and Chapinero for their participation in the conducted surveys; Empresa de Acueducto y Alcantarillado de Bogotá (EAAB) for providing essential information in the development of the model of the affected area in the SWMM; and the members of the EcoCivil research incubator for their contributions to the development of the research.
REFERENCES
Speak, A. F., Rothwell, J. J., Lindley, S. J., & Smith, C. L. (2013). Rainwater runoff retention on an aged intensive green roof. Science of the Total Environment, 461-462, 28-38. Retrieved from http://ovidsp.ovid.com/ovidweb.cgi?T=JS&PAGE=reference&D=emed11&NEWS=N&AN=2013359896 [ Links ]
Almanza, G. (2017). Caracterización general del escenario de riesgo por inundación. Bogotá, Colombia: Instituto Distrital de Gestión de Riesgos y Cambio Climático. [ Links ]
Anderson, M., Lambrinos, J., & Schroll, E. (2010). The potential value of mosses for stormwater management in urban environments. Urban Ecosystems, 13(3), 319-332. Retrieved from https://doi.org/10.1007/s11252-010-0121-z [ Links ]
Banco Mundial. (2012). Análisis de la gestión del riesgo de desastres en Colombia: un aporte para la construcción de políticas públicas. En: Sistema Nacional de Información Para La Gestión del Riesgo de Desastres, 438. Retrieved from https://doi.org/333.3109861/A56 [ Links ]
Bernal-Pedraza, A. Y. (2010). Gestión del agua - una preocupación de las empresas ambientalmente responsables. Universidad & Empresa, (19), 87-106. [ Links ]
Blackhurst, M., Hendrickson, C., Asce, D. M., Matthews, H. S., & Asce, A. M. (2010). Cost-effectiveness of green roofs. Journal of Architectural Engineering, 16(December), 136-143. Retrieved from https://doi.org/10.1061/(ASCE)EE.1943-7870.0000589 [ Links ]
Buffam, I., Mitchell, M. E., & Durtsche, R. D. (2016). Environmental drivers of seasonal variation in green roof runoff water quality. Ecological Engineering, 91, 506-514. Retrieved from https://doi.org/10.1016/j.ecoleng.2016.02.044 [ Links ]
Consejo de Bogotá. (2009). Acuerdo 418 de 2009. Bogotá, Colombia: Consejo de Bogotá. [ Links ]
Conte, G., Bolognesi, A., Bragalli, C., Branchini, S., De Carli, A., Lenzi, C., Masi, F., Massarutto, A., Pollastri, M., & Principi, I. (2012). Innovative urban water management as a climate change adaptation strategy: Results from the implementation of the project “Water against climate change (wataclic)”. Water, 4(4), 1025-1038. Retrieved from https://doi.org/10.3390/w4041025 [ Links ]
DPAE, Departamento de Prevención y Atención de Emergencias. (2012). Inundaciones Bogotá. Bogotá, Colombia. [ Links ]
El Espectador. (2016). Inundaciones en Bogotá por fuertes lluvias. Bogotá, Colombia: El Espectador. [ Links ]
El Tiempo. (2015). Barrios de seis localidades afectados tras fuerte granizada en Bogotá. Bogotá, Colombia: El Tiempo. [ Links ]
El Tiempo. (2016). Fuertes inundaciones por lluvia en Bogotá. Bogotá, Colombia: El Tiempo . [ Links ]
Environmental Protection Agency, Water Supply and Water Resources Division, Efr, C. D. & M. Inc. (2004). Manual del usuario-SWMM. Washington, DCM, USA: Water Supply and Water Resources Division, Efr, C. D. , & M. Inc. [ Links ]
Fernández-Colón, G. (2009). La crisis del agua en América Latina. Revista Estudios Culturales, 2(4), 80-96. [ Links ]
Universidad Militar Nueva Granada, & Secretaría Distrital de Ambiente. (2008). Formulación del plan de ordenación y manejo de la cuenca del río salitre en el perímetro urbano del distrito capital, (080), 438-757. . [ Links ]
Green Building Consul. (2017). LEED. Washington, DC, USA: Green Building Consul. [ Links ]
Hashemi, S. S. G., Mahmud, H. B., & Ashraf, M. A. (2015). Performance of green roofs with respect to water quality and reduction of energy consumption in tropics: A review. Renewable and Sustainable Energy Reviews, 52, 669-679. Retrieved from https://doi.org/10.1016/j.rser.2015.07.163 [ Links ]
Herrera, J. A. (2016, March 16). Construcción en Bogotá registró 8,2 millones de m2 en proceso de construcción en 2015. Observatorio de Desarrollo Económico. Retrieved from http://observatorio.desarrolloeconomico.gov.co/construccion-construccion-bogota/construccion-en-bogota-registro-82-millones-de-m2-en-proceso-de [ Links ]
Hoyos-Giraldo, R., Acevedo Correa, L.M., & Álvarez Cuadrado, A.D. (2014). Application of green roofs locally as a solution to the deficit of green areas in Medellín, 1-18. Medellín, Colombia: Universidad de Medellín. [ Links ]
Johnston, J., & Newton, J. (2004). Building green. A guide to using plants on roofs, walls and pavements, The Queen´s Walk, London. London, UK: Greater London Authority. [ Links ]
Kamal, B. (2016). Falta de agua preocupa a Medio Oriente y Magreb. New York, USA: Inter Press Service. [ Links ]
Kravits, R. (2007). Green begins from the roof down. Campus Facility Maintenance, 4(2), 34-36. [ Links ]
Kroes, P. (2012). Technical artefacts: Creations of mind and matter. A Philosophy of Engineering Design, Philosophy of Engineering and Technology, 6, 127-161. [ Links ]
López, G., García, M. M., Gameros, L. A., & De la Rocha, A. P. (2015). Uso de un sistema de ahorro de agua para conservar ecosistemas para el recurso hídrico. Tecnología y ciencias del agua, 6(6), 125-135. Retrieved from http://www.scielo.org.mx/pdf/tca/v6n6/2007-2422-tca-6-06-00125.pdf [ Links ]
López-Patiño, G., Martínez-Solano, J., Fuertes-Miquel, V., & López-Jiménez, A. (2011). Dimensionado de depósitos domésticos de aguas pluviales utilizando series temporales de datos. Tecnología y ciencias del agua , 2(1), 51-64. [ Links ]
Martínez-Austria, P. F., & Vargas-Hidalgo, A. (2016). Modelo dinámico adaptativo para la gestión del agua en el medio urbano. Tecnología y ciencias del agua , 7(4), 139-154. Retrieved from https://www.scopus.com/inward/record.uri?eid=2-s2.0-85030454836&partnerID=40&md5=23b782c675ac16be3d810bc077da05dc [ Links ]
Ministerio de Ambiente Vivienda y Desarrollo Territorial. (2000). Reglamento técnico del sector de agua potable y saneamiento básico. Bogotá, Colombia: Ministerio de Ambiente Vivienda y Desarrollo Territorial. [ Links ]
Minke, G. (2004). Techos verdes. Planificación, ejecución, consejos prácticos. Montevideo, Uruguay: Editorial Fin de Siglo. [ Links ]
Nawaz, R., McDonald, A., & Postoyko, S. (2015). Hydrological performance of a full-scale extensive green roof located in a temperate climate. Ecological Engineering, 82, 66-80. Retrieved from https://doi.org/10.1016/j.ecoleng.2014.11.061 [ Links ]
Niño-Fierro, K. N. (2012). Análisis para la gestión del riesgo de inundaciones en Bogotá: un enfoque desde la construcción social del riesgo. Bogotá, Colombia: Pontificia Universidad Javeriana. [ Links ]
Observatorio Ambiental de Bogotá. (2016). Precipitación Mensual - PMPLL. Bogotá, Colombia: Observatorio Ambiental de Bogotá. [ Links ]
ONU, Organización de las Naciones Unidas. (2015). Objetivos de Desarrollo Sostenible. San Francisco, USA: Organización de las Naciones Unidas. [ Links ]
Oviedo, N., & Torres, A. (2014). Atenuación hídrica y beneficios hidrológicos debido a la implementación de techos verdes ecoproductivos en zonas urbanas marginadas. Ingeniera y Universidad, 18(2), 291-308. Retrieved from https://doi.org/10.11144/Javeriana.IYU18-2.hahb [ Links ]
Palla, A., Gnecco, I., & Lanza, L. G. (2010). Hydrologic restoration in the urban environment using green roofs. Water, 2(2), 140-154. https://doi.org/10.3390/w2020140 [ Links ]
Peña-Guzmán, C. A. (2016). El ciclo urbano del agua en Bogotá, Colombia : estado actual y desafíos para la sostenibilidad. Tecnología y ciencias del agua , 7(6), 57-71. [ Links ]
Psencik, C., & Heller, R. (2012). Is your building conducive to the installation of a green roof ? Operations Management, 17(2), 30-31. [ Links ]
RCN, Radio Cadena Nacional. (2017). Fuertes lluvias en Bogotá dejaron deslizamientos, vehículos atrapados e inundaciones. Bogotá, Colombia: Radio Cadena Nacional. [ Links ]
Rivera, P., & Aguila, A. G. (2015). La gestión integral del agua en zonas urbanas: caso de estudio Zacatecas-Guadalupe, México. Tecnología y ciencias del agua , 6(3), 125-142. [ Links ]
Rosatto, H., Botta, G., Becerra, A., Tardito, H., & María, L. (2016). Problemáticas del cambio climático en la Ciudad Autónoma de Buenos Aires - aportes de las cubiertas vegetadas en la regulación térmica/Climate change difficulties in the Buenos Aires city contribution of green roofs in regulating the thermal change. Fca Uncuyo, 48(1), 197-209. [ Links ]
Saadatian, O., Sopian, K., Salleh, E., Lim, C. H., Riffat, S., Saadatian, E., Toudeshki, A., & Sulaiman, M. Y. (2013). A review of energy aspects of green roofs. Renewable and Sustainable Energy Reviews, 23, 155-168. [ Links ]
Secretaría de Hacienda. (2017). Tendencia demográfica. Retrieved from http://www.institutodeestudiosurbanos.info/endatos/0100/0120/01211.htm [ Links ]
Secretaría Distrital de Ambiente. (2011). Guía de techos verdes en Bogotá. Bogotá, Colombia: Secretaría Distrital de Ambiente. [ Links ]
Spolek, G. (2008). Performance monitoring of three ecoroofs in Portland, Oregon. Urban Ecosystems , 11(4), 349-359. Retrieved from https://doi.org/10.1007/s11252-008-0061-z [ Links ]
Sultana, M. N., Akib, S., & Ashraf, M. A. (2017). Thermal comfort and runoff water quality performance on green roofs in tropical conditions. Geology, Ecology, and Landscapes, 1(1), 47-55. Retrieved from https://doi.org/10.1080/24749508.2017.1301058 [ Links ]
Sun, C. Y., Lin, Y. J., Sung, W. P., Ou, W. S., & Lu, K. M. (2012). Green roof as a green material of building in mitigating heat island effect in Taipei City. Applied Mechanics and Materials, 193-194, 368-371. Retrieved from https://doi.org/10.4028/www.scientific.net/AMM.193-194.368 [ Links ]
Teemusk, A., & Mander, Ü. (2011). The Influence of green roofs on runoff water quality: A case study from Estonia. Water Resources Management, 25(14), 3699-3713. Retrieved from https://doi.org/10.1007/s11269-011-9877-z [ Links ]
Todorov, D., Driscoll, C. T., Todorova, S., & Montesdeoca, M. (2018). Water quality function of an extensive vegetated roof. Science of the Total Environment , 625, 928-939. Retrieved from https://doi.org/10.1016/j.scitotenv.2017.12.085 [ Links ]
Vijayaraghavan, K., Reddy, D. H. K., & Yun, Y. S. (2019). Improving the quality of runoff from green roofs through synergistic biosorption and phytoremediation techniques: A review. Sustainable Cities and Society, 46(September 2018). Retrieved from https://doi.org/10.1016/j.scs.2018.12.009 [ Links ]
Wang, X., Tian, Y., & Zhao, X. (2017). The influence of dual-substrate-layer extensive green roofs on rainwater runoff quantity and quality. Science of the Total Environment , 592, 465-476. Retrieved from https://doi.org/10.1016/j.scitotenv.2017.03.124 [ Links ]
Wilkinson, S., Lamond, J., Proverbs, D. G., Sharman, L., Heller, A., & Manion, J. (2015). Technical considerations in green roof retrofit for stormwater attenuation in the Central Business District. Structural Survey, 33(1), 36-51. Retrieved from https://doi.org/10.1108/SS-07-2014-0031 [ Links ]
Yan, B. (2011). The research of ecological and economic benefits for green roof. Applied Mechanics and Materials , 71-78, 2763-2766. Retrieved from https://doi.org/10.4028/www.scientific.net/AMM.71-78.2763 [ Links ]
Yang, W. Y., Li, D., Sun, T., & Ni, G. H. (2015). Saturation-excess and infiltration-excess runoff on green roofs. Ecological Engineering , 74, 327-336. Retrieved from https://doi.org/10.1016/j.ecoleng.2014.10.023 [ Links ]
Zhang, Q., Wang, X., Hou, P., Wan, W., Li, R., Ren, Y., & Ouyang, Z. (2014). Quality and seasonal variation of rainwater harvested from concrete, asphalt, ceramic tile and green roofs in Chongqing, China. Journal of Environmental Management, 132, 178-187. Retrieved from https://doi.org/10.1016/j.jenvman.2013.11.009 [ Links ]
Zielinski, S., Carlos, J., Paternina, V., & Marta, S. (2012). Green roofs: A feasible tool for environmental management in the hospitality sector of El Rodadero, Santa Marta? Gestión y Ambiente, (1), 91-104. [ Links ]
Received: August 14, 2017; Accepted: April 29, 2019