Introduction
Riparian communities (e.g., fish fauna, benthic insects and riparian trees) typically contain diverse species assemblages that play a vital role in the health and productivity of aquatic ecosystems. Since rivers of semi-arid and subtropical landscapes are classified as intermit-tent and some segments as permanent streams (Levick et al., 2008), riparian communities adapt well to variable environments by developing resistance traits. High spatial and temporal river flow variability as well as a strong longitudinal zonation in temperature, discharge, water quality, substratum and biota feature semi-arid and sub-tropical rivers; with high flows quite often synchronized with seasonal precipitation events. In the dry season, low-velocity baseflow travel for short distances along the river chan-nel building waterholes and stagnant ponds that are often the only source of freshwater for fragmented riparian communities (Williams, 2006). In the wet season, high flow or flood pulses re-connect river streams allowing sev-eral riparian faunal species to migrate locally and regionally to re-colonize neighboring and distant streams (Bunn, Davies, & Winning, 2003; Nadeau & Rains, 2007). The high spatial and temporal river flow variability, water quality, and predator-prey interactions make riparian species and communities to adapt well across these longitudinal gradients of river flows. However, resistance traits to river flow variability must be different between biological groups (e.g., fish fauna and benthic insects) and linked to e.g., migration distances, river flow requirements, among others.
Fish are ecologically important in rivers because they are often the apex predator in aquatic ecosystems. However, the diversity and abundance of fish species vary due in part to the patchy distribution of phreatic vegetation (Marsh-Mathews & Mathews, 2000; Pusey & Arthington, 2003) as well as due to the high temporal flow variability in semi-arid and subtropical rivers (Levick et al., 2008). Riparian vegetation is frequently correlated to fish faunal species assemblages as it provides (a) energy for primary and secondary productivity food webs for several terrestrial and aquatic biota (Pusey & Arthington, 2003; Pettit et al, 2012); (b) food, cover, and refugee sites for aquatic as well as for terrestrial faunal species (Arthington, Balcombe, Wilson, Thomas, & Marshall, 2005; Entrekin, Tank, Rosi-Marshall, Hollein, & Lam-berti, 2009); (c) shade for moderating air and water temperatures (Bunn et al., 2003); (c) bank stability (Levick et al, 2008); and (d) filters for reducing contaminants, sediments, and debris (Roy, Rosemond, Leigh, Paul, & Wallace, 2003).
Temporal stream flow variability, discontinuous riparian vegetation patches and fish faunal assemblages have also been linked to the diversity-abundance of benthic insects (Johnson & Ringler, 2014); as these insects control nutrient cycles, primary productivity, decomposition and translocation of river materials (Peeters, Gylstra, & Vos, 2004). Several benthic insect species are important consumers and important prey organ-isms (Whatley et al., 2014); controling the relative abundance of other faunal species in streams (Whatley et al., 2014). In summary, amongst the most important process shaped by the benthic insect community are: detrivory, grazing, and predator-prey interactions. Detrivory is the process of feeding on decaying organic matter and it is a major insect-mediated process. Benthic insects graze in nearly all aquatic ecosystems contributing largely to primary productivity of riparian ecosystems. Many benthic insects of all major groups function as predators but predatory fish often control the benthic insect community structure (Verdonschot, Didderen, & Verdonschot, 2012; Whatley et al, 2014).
Regardless of the importance of riparian ecosystems of semi-arid landscapes (Harper, Sanderson, & McArthur, 1992; Levick et al, 2008) some river components have not been properly described in Mexico's northeastern San Juan River (Návar, 2004, 2011). Important efforts had been previously carried out to describe the fish faunal species diversity along major tributaries of the San Juan River (Contreras-Balderas, 2000). However, more systematic studies are required in order to understand sources of fish faunal assemblage spatial variability across longitudinal gradients. There is also a lack of local information on the benthic insect community and how diversity and abundance of riparian fish fauna and benthic insect communities transition along longitudinal gradients of river flow as well as across different kinds of riparian vegetation. This information is critical to understand local controls of species diversity and abundance in order to eventually set appropriate restoration measures. Therefore, this report aimed: a) to describe the fish, benthic insect, and tree riparian communities and b) to associate diversity and abundance of fish fauna and benthic insect communities to riparian trees, stream flow variability, river flow quality parameters and constituents for 10 sampling sites placed along three tributaries of Mexico's northeastern San Juan River.
Materials and methods
Description of the study area
This research was conducted in Ramos and Pilon rivers, and the main stem of the San Juan River of Mexico's northeastern State of Nuevo Leon. These rivers rise in the upland watersheds of the Sierra Madre Oriental Mountain Range of Nuevo Leon and Coahuila. The main stem of the San Juan River flows through the Plains of the Northern Gulf of Mexico and drains into the lower Rio Bravo/Rio Grande at Camargo, Tamps., Mexico. Ten sites were systematically chosen for sampling. When selecting sampling sites, the following sources of variation were considered: presence of riparian vegetation; water in the form of stream flow, waterholes or stagnant ponds; and a well defined channel. Sampling sites and major features of the land-scape are depicted in Figure 1.

Figure 1 The location of the sampling sites along the San Juan River watershed in northeastern, Mexico. ChP = Cortijo- El Chapotal; BSJ = La Boca; ChSJ = Ejido Chihuahua; FR = El Fraile-Terreros; HCP = Hacienda-La Corona; PSJ = El Porvenir; RR = Raíces; FSJ = La Fragua; MSJ = San Miguelito; TR = Las Trancas; P = Pilón; R = Ramos; SJ = San Juan. Note: Upstream sites are ChP, TR, RR, FR, HCP; downstream sampling sites are: BSJ, ChSJ, PSJ, FSJ, MSJ. HCP was placed just before ites confluence with the major stem of the San Juan River.
Sampling
Sampling consisted of measuring the physi-cal, chemical, and bacteriological parameters and constituents of streams, trapping fish and benthic insects, and measuring the dimensional characteristics of riparian trees. Sampling of fish, benthic insects, and riparian trees was car-ried out during the day from March to June of 1999. At least one biological group was sampled weekly during the study period. Trees and shrubs were measured in two plots placed at the first terrace, immediately outside the river bank with dimensions of 40 m x 20 m. In each plot, we recorded diameter at breast height, dbh, top height, H, and canopy cover, Cc, for each individual tree.
Nets dragged perpendicular to river flow for one hour collected fishes, taking care to drag on refugee sites beneath the river bank. Net was 5 m in length and 1 m width. Surber-type sam-plers with 1 m in width collected benthic insects by dragging the bottom part of the stream across the channel or perpendicular to the river flow. Dragging was carried out three times at different places immediately below where the fish was collected. Collected macroinvertebrates were sorted and placed in large buckets filled with water and taken to the laboratory for counting and taxonomical identification. Collected fish and benthic insects were taxonomically identified in Mexico's Laboratory of the Facultad de Ciencias Biologicas, UANL, in Monterrey, N.L., Mexico.
Water samples were collected at midstream by way of wading and submerging plastic containers with a volume of 4 L to a depth of 20 to 30 cm, following the methodology proposed by Flores-Laureano and Návar (2002). Chemical constituents, and physical and bacteriological parameters were determined using laboratory methods of the Mexican Water Commission (CNA, 1993, 1997) following the standards of the Ministry of Urban Development and Ecology, denominated the NMX-AA-XX (Method Number)-YY(year) protocols for water analysis. For example, the method used for alkalinity de-termination is NMX-AA-36-1980. Measurements of stream velocity and river cross sectional area estimated discharge (Q) during each sampling event. Discharge measurements followed the Mexican Water Commission standard as well. In addition, we recorded channel slope and limnological measurements for each sampling site.
Data analysis
We evaluated the alpha diversity by quantifying the species richness, Shannon, and Shannon's equitability parameters (Magurran, 2004). Shannon's index accounts for both species abundance and species evenness and can be calculated by dividing H by Hmax (here Hmax = ln(S)). Equitability assumes a value between 0 and 1 with 1 being complete evenness. Diversity indices were estimated for each biological group for each sampling site. Equations described below evaluated each diversity index. The diversity index of Shannon - Weiner is:
Where pi = proportion of the total sample belonging to the ith species (ni /N), ln = natural logarithm. The Shannon's equitability (EH ) is (Pielou, 1975):
Four diversity-abundance models fitted each sampling site for each biological group to explain partition of limiting resources within the species and to draw conclusions on potential succession processes that shapes the community structure of each biological group. The geometric series, the log series, the truncated log normal distribution, and the broken stick diversity-abundance equations fitted the community assemblage structure. The geometric series is:
Where ni = abundance of the ith species; N = total number of individuals or abundance; Ck = a constant, which assures that Σni = N; i = species rank. The logarithmic series is:
Where αx = the number of species with one individual; αx2/2 = the number of species with two individuals; α = (N(1-x))/x, and S/N = (1-x)/x(/ln(1-x)). The truncated log normal series is:
Where α = (2σ2n) = a constant describing the amount of spread in the distribution; S(R) = number of species to occur in the Rth octave (=class) to the right or left of the symmetric curve; So = number of species in the modal octave (the largest class). The broken stick model is (McArthur, 1988):
Where S(n) = number of species in the abundance class that present n individuals; S = number of species; N = number of individuals or abundance.
The similitude index of Morisita-Horn evaluated the beta diversity index, which is based on the richness and abundance of the most common species.
The index of Morisita-Horn, as described by Magurran (2004) is model (7):
Where aN = total abundance of individuals in site A; bN = total abundance of individuals in site B; an. = abundance of the i-th species in site A; bn. = abundance of the -th species in site B:
The equal diversity null hypothesis between sampling sites was tested by the Shannon Weiner diversity index, since Hutcheson (1970) described the t test given by equation (10):
Where H1,2 = indices of Shannon in samples 1 and 2; Var H1 and H2 = deviance of the index of Shannon in samples 1 and 2 with degrees of freedom given by equation (11):
Where N1 and N2 = number of individuals in samples 1 and 2.
Detrended canonical correspondence analy-sis was performed for each biological group using river flow discharge and quality parameters as the environmental variables to understand clusters and patterns of species and sampling sites as well as the major sources of variation explaining clusters and patterns. In addition, several regression equations were tested to predict e.g., fish fauna species assemblages as a function of the riparian tree or the benthic insect species as exogenous variables using several alpha diversity indices.
Results
Stream water parameters and constituents
Several constituent concentrations and parameters exceeded the US-EPA National Primary Standards for drinking water for chloride, fecal coliform bacteria, total coliform bacteria, color, total dissolved solids, and sulfates (Table 1). Tributaries El Pilon (ChP, HCP) and Ramos (FR, RR, TR) had the fewest number of exccedances in contrast to the main stem of the San Juan River (BSJ, CHSJ, PSJ, FSJ, and MSJ). Water quality parameters and constituents deteriorated from upper (ChP and FR) to middle stream (ChSJ, HCP, and PSJ) and to lower sites (FSJ and MSJ).
Table 1 Concentration statistics for measured constituents and parameters of stream water quality of the San Juan River, N.L., Mexico.

Bold and Italic values of constituents and parameters exceed the US-EPA National Primary Standards for drinking water. ChP = Cortijo, El Chapotal; BSJ = La Boca; ChSJ = Ejido Chihuahua; FR = El Fraile-Terreros; HCP = Hacienda, La Corona; PSJ = El Porvenir; RR = Raíces; FSJ = La Fragua; MSJ = San Miguelito; TR = Las Trancas; P = Pilón; R = Ramos; SJ = San Juan. T = total; Env. environmental; SW = surface water; S = suspended.
A principal component analysis reduced the multiple dimensions of the water quality parameters and constituents and indicated that 92% of the total variance was explained by the first seven components. Therefore variables with the largest weight on each component were selected to explain the intrinsic sources of variability. These included phenolic alkalinity, electric conductivity, oil content, nitrite-nitrate concentration, total and dissolved solids, stream discharge, temperature, and turbidity.
The riparian tree community
Tree communities of Mexico's northeastern San Juan River are diverse and abundant (Table 2). We recorded a total of 788 trees of 24 genera, 16 families, and 13 orders. Average stand den-sity was only 500 trees ha-1, with a standard deviation of 488 trees ha-1 per sampling stand. Taxodium mucronatum, a typical riparian tree and an abundant tree species, was absent in four out of ten downstream sites (PSJ, RR, FSJ, and MSJ). T. mucronatum was also quite rare along sampling site TR with only four trees in two plots of 800 m2 each. Riparin tree communities with this typical phreatophitic tree as the dominant tree species are located in upper stands of main tributaries. A mixture of riparian and xerophytic tree species dominates lower riparian corridors.
Table 2 Diversity-abundance of riparian tree communities in 10 sites placed along the San Juan River of northeastern Mexico.

ChP = Cortijo, El Chapotal; BSJ = La Boca; ChSJ = Ejido Chihuahua; FR = El Fraile-Terreros; HCP = Hacienda, La Corona; PSJ = El Porvenir; RR = Raíces; FSJ = La Fragua; MSJ = San Miguelito; TR = Las Trancas; P = Pilon; R = Ramos; SJ = San Juan.
In lower sampling stands, we measured a large number of xerophitic tree species. Acacia farnesiana was frequently observed in 7 out of 10 sampling sands, although it accounted for less than 10% of the total abundance. Other xerophitic tree species found preferentially in lower stands (P. aculeata, E. anacua, E. ebano, L. leucocephala, H. pallens, C. laevigata, C. pallida, Z. fagara, P. laevigata, S. greggii, and D. texana) accounted for less than 50% of the recorded diversity.
Tree diversity varied between sampling sites. The t test on the Shannon-Weiner diversity index showed tree diversity was smaller at the middle stream, PSJ, sampling site since it had only 4 tree species and 90 trees ha-1. Evenness is larger at lower (HCP, FSJ, MSJ, and TR) than at the upper sampling sites (ChP, BSJ, and FR). All diversity-abundance models predicted well the community structure pointing at a succes-sional equilibrium of the plant community. In spite of the presence of xerophitic species, the tree riparian community has sufficient late successional tree species (T. mucronatum, P. occidentalis, P. tremuloides, S. nigra) in most upper and middle sampling sites for fitting any diversity-abundance model (Table 3).
Table 3 Diversity indices and diversity-abundance models fitted to the riparian tree community of the San Juan River of northeastern Mexico.

ChP = Cortijo, El Chapotal; BSJ = La Boca; ChSJ = Ejido Chihuahua; FR = El Fraile-Terreros; HCP = Hacienda, La Corona; PSJ = El Porvenir; RR = Raíces; FSJ = La Fragua; MSJ = San Miguelito; TR = Las Trancas; P = Pilón; R = Ramos; SJ = San Juan. HO and HA = null and alternate hypotheses.
The beta diversity described by the Morisita-Horn index was quite small for all sampling sites, with an average (confidence interval) of 0.28 (± 0.09). That is, the riparian tree community is quite different between sampling sites with only a few species colonizing neighboring stands. In general, sampling sites placed at a similar relative location, e.g., the upper sites, recorded large beta indices (ChP, BSJ, ChSJ, FR). The beta diversity dropped sharply at lower sites of the same tributary.
The large variability of the riparian tree assemblages makes to fit well all successional distributions: the logarithmic series (intermediate); the log-normal (intermediate); the geometric (pioneer) or the broken stick (late successional) models.
The detrended canonical correspondence analysis (DCCA) indicated the first two tree species axis accounted for 35% of the total species deviance and 60% of the total tree species-environment relation. Two longitudinal gradients could be noted in Figure 2.

Figure 2 Results of the DCCA analysis on the riparian community of trees of the San Juan River, N.L., Mexico. ChP = Cortijo, El Chapotal; BSJ = La Boca; ChSJ = Ejido Chihuahua; FR = El Fraile-Terreros; HCP = Hacienda, La Corona; PSJ = El Porvenir; RR = Raíces; FSJ = La Fragua; MSJ = San Miguelito; TR = Las Trancas; P = Pilón; R = Ramos; SJ = San Juan.
The first one moves along the Y axis from the center (ChSJ, ChP), to the middle (FR, HCP), to the upper (RR) part of the Y axis. Tree species Taxodium mucronatum, Populus tremuloides, Juglans spp., Morus microphylla, and Leucaena leucocephalla clustered around upper sampling sites (FR, ChP, ChSJ, RR, BSJ) featured by low stream temperature, high discharge, best water quality parameters and constituents, and small channel width, area and depth (Figure 2). The second longitudinal gradient is located along the X axis, moving from the center (BSJ, ChSJ, ChP) to the right (MSJ, FSJ, PSJ) of Figure 2. The tree species, Platanus occidentalis, Dodonea viscosa, Salix nigra, Bacharis spp., and Caesalpinia mexicana clustered around lower sites (ChSJ, FSJ, PSJ, and MSJ). Lower sampling sites (FSJ, PSJ, MSJ, and TR) are characterized by increased stream flow temperature and pollution levels, channel depth, and reduced discharge. Acacia farnesiana, Ehretia anacua, Diospyros texana, Pithe-cellobium pallens, and Fraxinus spp. agglomer-ated around middle and lower sites (TR, MSJ, FSJ, PSJ).
The fish community
The San Juan River hosts an important fish diversity and abundance (Table 4), since we captured a total of 5 608 fishes classified in 17 genera and 8 families. Gambusia affinis, a grazer fish species that colonizes the lower parts of rivers accounted for close to 50% of the total fish abundance. Of the 18 fish species sampled, 7 are grazers, 3 are carivores, 5 are invertivores, and the remaining 3 species have unknown feeding habits. Gambusia affinis, Poecilia formosa, and Cichlasoma cyanoguttatum have large ecological plasticity or widespread habitat since these species were captured at 7 out of 8 sampling sites. On the other side, the fish species L. macrochirius, E. grahami, H. jonesi, and C. lutrense have a more restricted longitudinal habitat since they were captured at only one site.
Table 4 Diversity-abundance of fish species captured in 10 sites of three tributaries of the San Juan River, Nuevo Leon, Mexico.
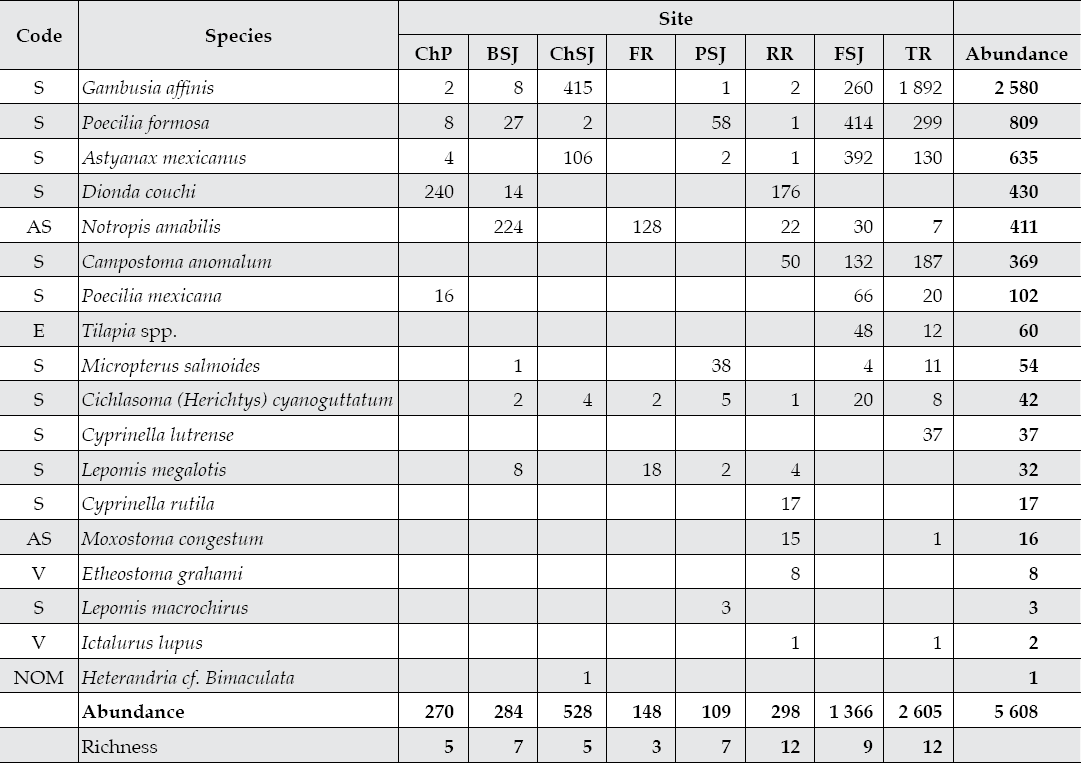
ChP = Cortijo, El Chapotal; BSJ = La Boca; ChSJ = Ejido Chihuahua; FR = El Fraile-Terreros; HCP = Hacienda, La Corona; PSJ = El Porvenir; RR = Raíces; FSJ = La Fragua; MSJ = San Miguelito; TR = Las Trancas; P = Pilon; R = Ramos; SJ = San Juan. S = secure, AS = apparently secure, V = imperiled, NOM = NOM-059-SEMARNAT-2001.
Most captured fish species are classified as secure in terms of the round global status (Nature Server Explorer, 2008). However, two fish species are classified as apparently secure (AS) in southern USA (Notropis amabilis and Moxostoma congestum). Etheostoma grahami, Ictalurus lupus, and Compostoma anomalum are endemic species to Mexico's northeastern San Juan River and southern Texas. The first species is locally classified in the NOM-059-SEMARNAT-2001, and it was captured only at RR, a sampling site with the best river flow quality parameters. Heterandria cf. jonesi is an invasive species first recorded during this study in the San Juan River watershed, since it was absent on the list reported by Contreras-Balderas (2000) and it was captured only at ChSJ, a heavily polluted sampling site.
The fish diversity indices and diversity-abundance models are reported in Table 5. Sampling sites placed at lower sampling sites such as the Ramos River (RR and TR) recorded the largest fish diversity with 12 out of the 18 species. Sampling sites placed along the upper Ramos River (FR) and Pilon River (ChP) had the lowest fish diversity-abundance.
Table 5 Diversity indices and diversity-abundance models fitted to the fish communities of the San Juan River in northeastern Mexico.
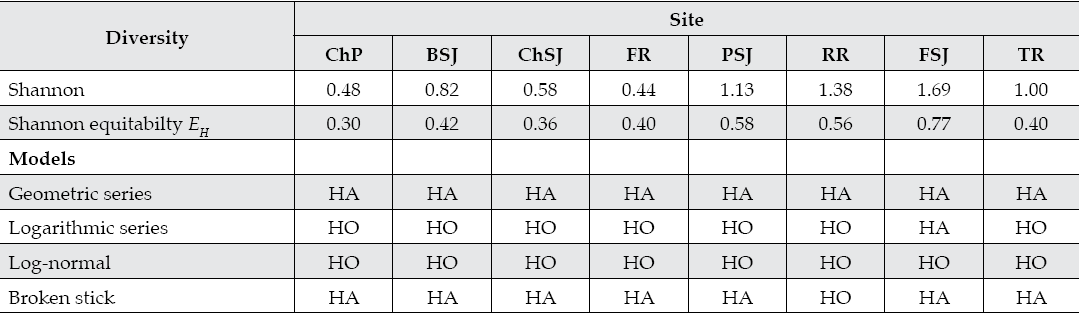
ChP = Cortijo, El Chapotal; BSJ = La Boca; ChSJ = Ejido Chihuahua; FR = El Fraile-Terreros; HCP = Hacienda, La Corona; PSJ = El Porvenir; RR = Raíces; FSJ = La Fragua; MSJ = San Miguelito; TR = Las Trancas; P = Pilón; R = Ramos; SJ = San Juan; HO and HA = null and alternate hypotheses.
The Shannon index varies notoriously between sampling sites. The fish diversity was lowest at the upper sites (ChP, ChSJ, and FR) and largest at the lower sites FSJ and RR. FSJ and RR recorded one of the largest abundance and species richness of all sampling sites. The rest of the sampling sites differ in a significant manner from these two groups. Evenness had a tendency to increase from upper to lower sampling sites. The log normal and logarithmic series diversity-abundance models fitted the fish community structure better. The pioneer and late succesional models represented by the geometric series and the broken stick models did not fit the fish assemblage structure for any particular site. Since the geometric series and the broken stick model apply well to pioneer and late successional stages or well balanced communities at any developmental stage, recorded data shows and fitted models predict the fish community is in the secondary stage of succession.
The beta diversity index was also quite small for all sampling sites, with an average (confidence interval) of 0.18 (± 0.12). That is, the fish community differs between sampling sites with only a few species distributing and colonizing neighboring sampling sites. Similar dominant fish species were observed at sites of similar location; e.g., upper sites ChP and RR and middle sites BSJ and FR. Sampling sites ChSJ and TR recorded the largest beta index (> 0.85). However, fish fauna collected in the same tributary (e.g., FR, RR, TR; or ChP, HCP) had small beta diversity indicating a lack of connectivity between fish species.
The fish assemblages fitted better the logarithmic series and the log-normal model unlike the geometric (pioneer) or the broken stick (late) models stressing the community is found within the intermediate stages of succession.
The DCCA analysis carried out on the logarithmic transformation of the abundance data showed the first two species axis accounted for 47% of the total fish species variance and 35% of the total fish species-environment relation (Figure 3).

Figure 3 Results of the DCCA analysis on the fish community of the San Juan River, N.L., Mexico (TD Solids = total disolved solids, Feno Alkalinity = fenolic alkalinity, T. Solids = total solids). ChP = Cortijo- El Chapotal; BSJ = La Boca; ChSJ = Ejido Chihuahua; FR = El Fraile-Terreros; HCP = Hacienda, La Corona; PSJ = El Porvenir; RR = Raíces; FSJ = La Fragua; MSJ = San Miguelito; TR = Las Trancas; P = Pilón; R = Ramos; SJ = San Juan.
Two longitudinal gradients were noted in the fish structure assemblages. The first gradient moves along the Y axis and is described by E. grahami, C. rutila, M. congestum, N. amabilis, D. melanops, N. jamezanus, and I. lupus, as well as by sampling upper sampling sites ChP, RR, FR, BSJ. Low streamflow temperature, high discharge rate, as well as low nitrite-nitrate concentra-tions, suspended solids, and total suspended solids characterize these places (Figure 3). The second longitudinal gradient moves parallel to the first one but on the right hand side of Figure 3. The fish species C. lutrense, P. spp., G. affinix, A. mexicanus, Tilapia spp., P. formosa, C. cyanoguttatum, L. macrochirius, and M. salmonoids colonized the lotic waterholes and ponds of lower sites FSJ, PSJ, TR featured by increased streamflow temperature, small discharge rate, larger nitrite-nitrate concentrations and suspended sediments.
The benthic insect community
The benthic insect community was the most diverse biological group found on the Mexico's northeastern San Juan River (Table 6). The data source was made of 865 individuals, which belong to 39 different species, 34 genera, 23 families, and 6 orders. Families Ephemeroptera and Coleoptera contained the highest species diversity with 38% and 37%, of the total diversity, respectively. The family Trichoptera recorded the least relative abundance, with only 3.4% of the total diversity. Middle sampling sites showed the largest benthic insect diversity (PSJ) ac-counting for approximately 50% of the total observed diversity. However, the upper, most pristine sampling site along the Ramos River recorded the largest diversity of rare species. The Ramos River had the largest benthic insect diversity with 12 out of the 18 species observed here. Sampling sites distributed along the upper Ramos and Pilon rivers had the smallest benthic insect diversity and abundance.
Table 6 Diversity-abundance of benthic insect species captured in 10 sites of three tributaries of the San Juan River, Nuevo Leon, Mexico.

ChP = Cortijo- El Chapotal; BSJ = La Boca; ChSJ = Ejido Chihuahua; FR = El Fraile-Terreros; HCP = Hacienda, La Corona; PSJ = El Porvenir; RR = Raíces; FSJ = La Fragua; MSJ = San Miguelito; TR = Las Trancas; P = Pilón; R = Ramos; SJ = San Juan.
Most frequently observed benthic insect species (Argia spp. (8), Baetis spp. (8), Psephenus spp. (6) and Tricorythodes spp. (6). Macrothemis spp., Callibaetis spp., Tanytarsus spp., and Phyllogomphoides spp.) are classified as rare species in these rivers of northeastern Mexico. In this riparian ecosystem, diversity generally increased with abundance. The least polluted Ramos River reported the highest insect diversity and one of the largest abundances with greater than 30% of the total number of benthic insects captured.
The Shannon-Weiner diversity index for benthic insects varied between sampling sites. Sampling sites BSJ and ChSJ recorded the smallest and sampling sites FR and PSJ recorded the largest alpha diversity index. Evenness showed a tendency to decrease from upper to lower sampling sites. The logarithmic series model fitted the benthic insect diversity-abundance structure for 9 out of 10 sampling sites. This community is in a secondary stage of succession. Sampling sites with intermmediate diversity-abundance parameters (FSJ, MSJ, TR) had a tendency to show species of the secondary stages of succession. Sampling sites with the largest Shannon diversity index (FR and PSJ) as well as with the highest water quality parameters tended to be fit by all diversity-abundance models. That is, the abundance distribution of species is well balanced and appears that resources are well distributed between the few species observed.
The beta diversity index was quite small for all sampling sites, with an average (confidence interval) of 0.22 (± 0.08). That is, the benthic insect community diversity varies greatly between sampling sites. The probability of finding values > 0.75 was similar between rivers and quite low in neighboring sampling sites and the probability diminished even more in sampling sites farther appart. That is, there is a lack of spatial tendencies or patterns of species con-nectivity in the beta diversity index between and among sampling sites. The patchy structure of benthic communities given by the small beta index shows the existence of place to place dif-ferences in environmental features such as food distribution, substrate, discharge, water quality, among others. Stream disturbances may also be controlling the lack of connectivity patterns in community diversity structure.
The large variability of the benthic insect assemblages makes to fit well all successional distributions as well: the logarithmic series (intermediate); the log-normal (intermediate); the geometric (pioneer) or the broken stick (late successional) models.
The first two DCCA axes accounted for 44% of the total benthic insect species variance and 45% of the total benthic insect species-environment relation. Two longitudinal gradients could be identified in Figure 4.

Figure 4 Results of the DCCA analysis on the benthic insect community of the San Juan River, N.L., Mexico (TD Solids = total dissolved solids, Feno Alkalinity = fenolic alkalinity, T. Solids = total solids). ChP = Cortijo-El Chapotal; BSJ = La Boca; ChSJ = Ejido Chihuahua; FR = El Fraile-Terreros; HCP = Hacienda, La Corona; PSJ = El Porvenir; RR = Raíces; FSJ = La Fragua; MSJ = San Miguelito; TR = Las Trancas; P = Pilón; R = Ramos; SJ = San Juan.
Table 7 Diversity indices and diversity-abundance models fitted to the benthic insect communities of the San Juan River in northeastern Mexico.

ChP = Cortijo- El Chapotal; BSJ = La Boca; ChSJ = Ejido Chihuahua; FR = El Fraile-Terreros; HCP = Hacienda, La Corona; PSJ = El Porvenir; RR = Raíces; FSJ = La Fragua; MSJ = San Miguelito; TR = Las Trancas; P = Pilón; R = Ramos; SJ = San Juan. HO and HA = Null and alternate hypotheses.
The first one is characterized by the benthic insect families Ambrysus, Helicopsyche, Lype, Psephenus, Leucotabanus, Argia, and Ancyronyx colonizing sampling sites FR, ChP, RR, TR, and ChSJ. These families inhabit mostly upper tributaries featured by the highest stream water quality indicators, with low concentrations of suspended solids, nitrite-nitrate, oil content, fenolic alkalinity; as well as with lowest streamflow temperatures and highest discharge rates. The second longitudinal gradient is characterized by the benthic insect families Neurocordulia, Berosus, Baetis, Arisoptera, Larsia, Caenis, Ablasbemyia, Nimbocera, Chironomus, Hidrophilidae, Anopheles, and Tenagobia distributed along sampling sites ChP, PSJ, BSJ, MSJ, and HCP.
Discussion
The studied riparian communities (trees, fish and benthic insects) could be consistently classified by the DCCA analysis into upper and lower river-segment communities for all three major biological groups. A lack of connectivity of these biological groups between upper and lower sampling sites was identified as the major source of variation by several indicators; the DCCA analysis, the Shannon-Wiener alpha diversity index, and the Morisita-Horn beta diversity index.
Springs feeding on river channels in upland ridges along the piedmont characterize upper river segments; with cold flow temperatures, high water quality indicators, high flow, and a well defined channel cross section. Typical riparian tree communities (T. mucronatum, P. occidentales, Juglans spp., Populus spp.) colo-nize these stands. Fish fauna assemblages (E. grahami, C. rutila, M. congestum, N. amabilis, I. lupus, and N. jamezanus) and benthic insect families (Ambrysus, Helicopsyche, Lype, Psephenus, Leucotabanus, Argia, and Ancyronyx) thrive on these rivers and they are indicators of good water quality.
Stream temperature and pollution levels increase and flow diminishes at lower river segments. Diversity of typical riparian tree communities diminishes from upper to lower sampling sites. This tendency is related to the dissapearance of riparian phreatophitic and the appearance of xerophitic tree species. Other sources of variation such as soil type, altitude above sea level, and geology may be controling the presence of T. mucronatum across longitudinal riparian gradients (Hudak & Ketcheson, 1992; Rosentreter, 1992). The disappearance of typical riparian trees as the river flows away the piedmont can be one of the adjustments riparian vegetation use in response to the modification of river flow parameters (Johnson, 1998).
The fish diversity has a slight tendency to decline from upper to lower river segments. G. affinis, Poecilia spp., C. lutrense, A. mexicanus, L. megalotis and Tilapia spp. colonize well lower and middle sites (TR, FSJ, and ChSJ). These species assemblages are characterized by increased pollution levels, flow temperature and reduced discharge. Several chemical constituents (detergents and sulfates) and bacteriologic parameters (fecal coliform bacteria) exceed the NOM-001-ECOL-1996 standard that sets the maximum permisible limits on water quality parameters as well as the standard for aquatic organisms CE-CCA-001/89. De Lange (1994) and Villarreal (1983) noted several fish species described immediately above are indicators of poor stream water quality with depleted oxygen, high sedi-mentation rates, and degraded canopy cover.
The benthic insect communities thriving along lower river segments (Neurocordulia, Berosus, Baetis, Arisoptera, Larsia, Caenis, Ablasbe-myia, Nimbocera, Chironomus, Hidrophilidae, Anopheles, and Tenagobia) have been described as good indicators of deteriorated stream water quality (Phillips & Rainbow, 1993; Merrit & Cummins, 1996), high stream temperature and low flows (Haidekker & Hering, 2007). The presence of these species in polluted streams relates to their adaptation to these conditions as well as to the hability to degrade organic matter since Leyton and Voshell (1991) found these families can recycle up to 20% of the organic matter in streams. On the other side, Lenat (1993) noted the family Tricorytoides has the potential to be an indicator of good water quality.
River fragmentation by one of the most acute drought spells of the last century (1990's) may partially explain the lack of hydrological connectivity between upper and lower fish fauna and benthic insect communities. The decadal drought spell that affected Mexico's northern watersheds recorded consistently below average precipitation for most of the decade and started sometimes during 1989-1990 and finished some-times during 2002-2003 (Návar, 2015). During this period, river discharge stopped flowing in numerous occasions in several gauging sta-tions along perennial tributaries of the San Juan River (Návar, 2011). In spite of this acute natural disturbance that restricted long-range migration of several fish species (Ictalurus lupus, Lepomis megalotis, and Moxostoma congestum) as well as of several benthic insect species (Leptophlebia spp.) in upper river segements, the trophic structural levels are in good condition as the ecosystem contains primary consumers (Helicopysche spp. consumes algae), predators (Notropis amabilis) and preys (Chironomus spp.). The trophic chains are also well defined in lower river, polluted segments by the presence of predators (Ictalurus lupus and Gambusia affinis), preys (Anopheles spp. and Chironomus spp.) and the largest predator, the exotic fish species Tilapia spp.
Fish fauna and benthic insect communities are then well adapted to drought episodes of several time-scales by developing resistance traits. Adaptation traits appear are more variable between the benthic insect communities than between the fish fauna communities as the fish longitudinal gradient are better identified than the benthic insect longitudinal gradients. Fish is adapated to migrate short (Lepomis megalotis, Gambusia affinix, Poecilia spp.) and long-range (Ictalurus lupus, Ictalurus spp., Tilapia spp., Notropis amabilis, Moxostoma congestum) distances but they require of continuous river flow connectivity to colonize preferentially upper river segments. Benthic insects migrate but only for short distances and they may not need constant river flow connectivity all the time to meet the physiological needs.
The breaking of two fish faunal longitudinal gradients may partially be also a response to other sources of disturbance such as the presence of the introduced exotic fish Tilapia spp. This large predator was restricted predominantly to lower river segments characterized by high flow temperature and increased pollution levels. A lack of river connectivity during seasonal, interannual or decadal dry spells may modify local upper fish community assemblages as these conditions restrict migration of large fish predators such as Tilapia spp. and Ictalurus spp.
Observations between communities
The tree, fish, and benthic insect species diversity described by the EH index was larger at the sampling site scale than at the community scale. Hence, the replacement of species between neighboring sampling sites is quite large in this short longitudinal range of the studied streams. In spite of this, the species richness, S, diversity parameter was statistically related between biological groups; e.g., fish species, FS, was negatively related to riparian tree species, PCS, (FS = 12.63 - 0.62PCS; r2 = 0.30; P < F = 0.001) as well as to benthic insect species, BIS (BIS = 17.83 - 4.66 * Ln(FS); r2 = 0.23; P < F = 0.024). Then, BIS is also negatively related to PCS (BIS = 17.83 - 4.66 * ln (12.63 -0.062PCS). That is, the riparian tree community directly controls the diversity of the fish and benthic insect communities. Tree communities with a few typical riparian species colonizing upper river segments support the largest fish and benthic insect diversity and the strength of this relationship may fade away as lower river segments may have quite a small number of rare species. Lower river segments are the typical habitat of Tilapia spp. and the relationship between riparian tree species and fish may shift during unseasonal wet episodes.
Conclusions
This research points to the finding of two distinctive riparian communities defined by the tree and fish communities in upper (RR, HCP, FR, ChP) and lower (MSJ, FSJ, PSJ, TR) river segments. The former cluster is described by pristine, high river discharge, cold river flows, and high water quality. The most distinctive tree and fish species are Taxodium mucronatum-Juglans spp.-Populus tremuloides; Notropis amabilis-Etheostoma grahami-Ictalurus lupus-Moxostoma congestum; respectively. Lower river segments are characterized by deterio-rated river flow quality, small discharge, and high flow temperatures. Tree and fish species assemblages colonizing these river segments are Cephalanthus occidentalis-Bacharis glutinosa-Prosopis laevigata-Fraxinus spp.-Celtis laevigata; Lepomis megalotis-Lepomis macrochirus-Gambusia affinis-Poecilia mexicana; respectively. Tree and fish communities show a strong within-group but a lack of between-group connectivity in upper and lower sampling sites. The benthic insect community is classified into two clusters as well but they are more difuse indicating insects are better adapted to contrasting features of upper and lower river segments. It is concluded that riparian communites of Mexico's northern San Juan River are well adapted to variable river flow conditions controlled by hydro-climatic variability of several time scales.