Introduction
Conventional management practices of water resources cannot meet future water demands for development in arid, semi-arid, and subtropical countries (UNCED, 1992; Postel, 2000; Comprehensive Assessment Secretariat, 2006). Mexico's northern watersheds span the arid and semi-arid belts of North America of the boreal Hemisphere; where river flow is featured by erratic and variable temporal and spatial discharge (Ward, 1998; Návar-Cháidez, 2012). In addition, agriculture, population, industry, trade and services, among others rely upon river flow to meet conventional water demands (Conagua, 2012). In Mexico's northern drylands, these economic sectors have been steadily growing over the past 60 years due to (a) population growth and (b) economic development by increasing commercial treaties with other nations.
Climate variability coupled with steady increasing water diversions modifies river flow and takes a toll on wetland ecosystems (Postel, 2000; Návar-Cháidez & Lizárraga-Mendiola, 2013). The perturbation of river flow then requires new strategies to cope with conventional and environmental water demands, creating a positive feedback loop between increasing river perturbation and need for adaptation. For several watersheds, during acute dry episodes, river flow does not meet conventional demands and therefore society currently rely upon deep drilling and the inter-basin transfer of water (Conagua, 2012). In parallel to inter-basin water transfers, several sustainable practices of hydro-logic resources are occurring (Conagua, 2005). However, unsustainable practices remain on these watersheds, such as the high volume of irrigation vs low productivity of several irrigation districts; under valuing water resources, and highly regulating flow of most rivers (Pereyra, Oweis, & Zairi, 2002; Comprehensive Assessment Secretariat, 2006). In some cases, water use efficiency has increased, resulting in doubling productivity with the same amount of water. In addition, water withdrawals from domestic use (sometimes referred to as grey water) are being treated to further irrigate agricultural fields, along with other sustainable practices (Postel, 2000).
Climate variability is responsible for erratic and infrequent high rainfall episodes and river flow anomalies that usually start or end long drought episodes, a common feature of Mexico's northern as well as of most semi-arid drylands. Hurricanes and cyclones entering northeastern Mexico have a frequency period of close to 15 years (Návar-Chaidez, 2012). These climatic phenomena restore aquifer and reservoir storage, as well as river flow components. In dry spells, storage is frequently depleted. Drought episodes of several temporal scales; inter-annual, 3-7 years, 9-12 years, and 60-80 years have been well documented in the region and they have been correlated with large-scale climate phenomena such as El Niño/Southern Oscillation, the Pacific Decadal Oscillation, the Atlantic Multidecadal Oscillation, or by a combination of any of these synoptic-scale climate events (Cavazos & Hastenrath, 1990; Comrie & Glenn, 1998). Climate change may also in turn contribute to magnify river flow variability of most arid and semi arid watersheds. According to Mulholland et al. (1997), whose projections are based on Global Circulation Models, Northern Mexico would likely receive between 5 to 20% less rainfall and between 5 and 25% less streamflow should global warming trends continue.
The decadal drought spells of the 1950's, the 1990's, and the inter-annual droughts of the 2010's have prompted renewed efforts to quantify water storage and the length of temporal drying patterns. Hence, this report addresses whether river flow components of 172 gauging stations from five rivers show temporal monotonic patterns in the hydrologic regions of Sinaloa (RH10), Presidio-San Pedro (RH 11), Nazas-Aguanaval (RH 36), San Juan (RH 37), and San Fernando-Soto La Marina (RH 25). The working hypothesis was that there is no consistent change in any of the streamflow parameters or components over time and therefore no change in the magnitude of discharge.
Materials and Methods
The Study Area
The study area covers the basins of Sinaloa, Presidio-San Pedro, Nazas-Aguanaval, San Fernando-Soto La Marina and the Rio San Juan. These watersheds span the Mexican northern States of Sinaloa, Nayarit, Durango, Chihuahua, Zacatecas, Coahuila, Nuevo Leon, and Tamaulipas.
Present and future demographic information on each watershed is reported in Table 1.
A high diversity of climates features Northern Mexico (Conagua, 2005). Cold climate is typical of the highest peaks and the uplands of the main mountain ranges. Temperate climates characterize the central Valleys and Mesas of the Cordilleras. Subtropical and tropical climates facet the low ranges of the plains of the Pacific Ocean and Gulf of Mexico. Dry climates are typical in the Central Chihuahuan Desert and the southern Great Plains of North America.
Pine, oak, and a mixture of pine-oak forests cover extensive upland areas of the eastern and western Sierras Madre mountain ranges. Montane forests, dominated by thorny shrubs and low, broadleaved trees, distribute widely on the landscapes of the piedmonts of the main mountain ranges. Tropical and subtropical flora distribute in the plains and low lands of the piedmonts facing the oceans. Chaparral, dry, xerophytic plant cover is typical at the central Chihuahuan Desert and the southern Great Plains of North America. Tamaulipan thornscrub, acacia and mesquite forests extend into the lowlands of the Pacific Ocean, northern Gulf of Mexico and the Great Plains of North America (Rzedowskii, 1980).
Shallow Litosols are typical of the main mountain ranges. Deep Regosols, Xerosols, and Yermosols characterize the landscape of the central Chihuahuan Desert. Deep Vertisols are distributed in the plains in the lowlands of the Pacific Ocean and the northern Gulf of México.
Methodology
A total of 172 hydrometric stations placed across five major rivers were selected for breaking streamflow components and testing the time series for temporal monotonic patterns (Figure 1). The number of hydrometric stations analyzed per basin is depicted in Table 2.
Table 2 The number of hydrometric stations analyzed for temporal patterns and magnitude of river discharge in five Mexico's northern watersheds.

Data analyzed from Conagua (2005) records.
Hydrograph Separation Analysis
The separation of multiple river hydrographs into base and direct flow is a difficult task. To simplify this assignment for long term river flow time series, total daily discharge was split into base and direct or quick flow using the RORA hydrograph separation techniques (Rutledge, 1998). Before daily discharge data was fed into the RORA computer program, it was checked for: a) the presence of long periods of recession and b) the absence of intermediate missing data. Screen, a computer program, checks data for recession and continuity. The recession index is required in order to define the master curve. Once the master curve is developed, PART is used as a module of RORA to separate base and direct flow.
Monthly total discharge, standard deviation of daily discharge (within a given month), annual discharge, annual base flow, and annual direct flow or quick flow are parameters and components derived from daily discharge data. The nonparametric Mann-Kendall statistic tested for temporal monotonic trends on the river flow time-series data. This test reveals the presence or absence of temporal monotonic increasing or decreasing patterns.
The non-parametric Sen's method quantified the magnitude of slope change in all five discharge variables. The slope coefficient describes a change of the variable per unit time (annual time scale). The Sen's method assumes a monotonic tendency in the time series.
Data on land-use changes was compiled for several Mexico's northern ecosystems. In addition changes in precipitation and temperature parameters were also evaluated for several climatic stations. Correlations between river flow parameters and large-scale climate events (El Niño/Southern Oscillation, ENSO, The Pacific Decadal Oscillation, PDO, and The Atlantic Multidecadal Oscillation, AMO) previously reported aided to understand sources of river flow variability. This information, in addition to population growth, was linked to potential subtle river flow temporal patterns.
Results
Table 3 depicts the statistics of river flow components for all five studied watersheds. Base and direct flow are statistically similar for all watersheds. That is, on the average, 50% of river discharge is the low extended base flow rising from shallow (mountain range uplands) and shallow bottom-land aquifers. The remaining 50% of discharge is direct flow produced during and immediately after the rainy season that lasts from June to September. Hortonian surface and quick subsurface flow control direct flow. Watersheds draining into the Pacific Ocean (RH10 and RH11) and into the interior Central Plateau (RH 036) have a unimodal-monsoonal type of precipitation, with a major peak in July. On the other side, watersheds that drain into the Gulf of Mexico feature a bimodal type of rainfall distribution with two peaks, the first one during May-June and the last one during September-October.
Table 4 depicts the number and percentage of gauging stations with statistically significant negative (-) and positive (+) temporal monotonic tendencies.
Table 4 Number of gauging stations with statistical significant temporal monotonic patterns of several river flow parameters.

Std. Dev. = Standard deviation of monthly discharge. The last row was estimated from the ratio of the number of gauging stations with statistical significance to the total number of gauging stations analyzed.
In general, over 40% of each river flow parameter analyzed showed statistical significant temporal monotonic trends. That is, the river flow time-series are not stationary in the first momentum. However, of the total, on the average, 26% had a negative and the remaining 16% had a positive tendency. Total annual and total monthly river flow parameters are steadily declining at the San Pedro, Nazas, San Juan, and San Fernando watersheds but it is increasing at most gauging stations along the Sinaloa rivers.
Most gauging stations with declining temporal river flow patterns span the arid, semi-arid and subtropical watersheds RH11, RH36, RH37 and RH25, characterized as having an important area in the interior Valleys of the Chihuahuan Desert and the southern Great Plains of North America. In these physiographic regions, mon-sonic erratic, infrequent rainfall and high evapotranspiration losses control the hydrologic cycle. The human dominance on river flow is of least importance on these watersheds and therefore river flow is not as regulated as it is in e.g., the RH10.
Most gauging stations with positive temporal monotonic trends in total annual and total monthly river flow are distributed along the Sinaloa (RH10) basin, a watershed with most hydrometric stations placed along the lowlands of the Pacific Ocean. Most gauging stations were placed below irrigation districts, reservoirs, and urban centers, where human control exerted on water resources (increased diversions from upland reservoirs as well as on deep groundwater resources and discharge of excess water in other places) may explain the positive temporal trend of river discharge. Therefore, increasing river flow patterns over time may be partially explained by water resource management practices. This effect masks in the short run the potential subtle, changes in the local hydro - climate variables of the Pacific Ocean lowlands.
Reduced direct flows and increased base flows over time could be explained by the regulated flow effect by man-made reservoirs, which reduce discharge variation of most gauging stations below reservoirs when rivers are used as waterways to convey water to irrigation districts. Several river sections are experiencing the presence of smaller direct flows and larger base flows. Steady discharge of municipal sewerages and irrigation excess into streams may partially explain these observations.
Base flow is increasing in most gauging stations along the Sinaloa and San Pedro watersheds, but it is declining at most of the remaining gauging stations in the watersheds Nazas, San Juan, and San Fernando. Irrigation -excess, municipal discharge and increased diversions from deep aquifers of the interior watersheds partially explain this tendency. Subtle shifts in rainfall frequency, depth, and intensity may also be contributing to increasing aquifer recharge that may in turn control base flow discharge. In several watersheds, changes in the frequency and depth of rainfall coupled with increasing mean annual temperature may in turn contribute to modify parameters of river discharge.
Direct flows are also declining in most gauging stations of all watersheds. Increased storage by man - made reservoirs above 30 000 Mm3 in arid, semi-arid, and subtropical landscapes may partially explain the control exerted of precipitation-excess on river flow. The rate of change of discharge parameters is reported in Table 5.
Table 5 The median slope (and confidence intervals) for five discharge parameters with negative temporal tendency of 172 gauging stations placed in five Mexico's northern watersheds.
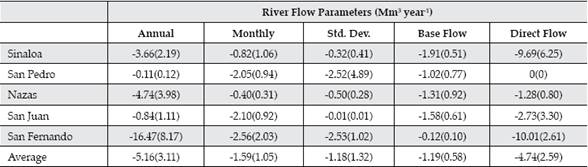
Std. Dev. = Standard deviation of monthly discharge
Most slope values statistically deviate from zero stressing the statistical significance on the declining of direct discharge over time. For total annual discharge, the San Fernando (-16.47 Mm3 year1), Nazas (-4.74 Mm3 year1) and Sinaloa (-3.66 Mm3 year-1) watersheds have the largest reductions of total annual discharge over time. The remaining watersheds present total annual discharge of less than -1.00 Mm3 year-1. For monthly river flow, discharge reductions are more important at the watersheds San Pedro (-2.05 Mm3 year-1), San Juan (-2.10 Mm3 year-1), and San Fernando (-2.56 Mm3 year-1).
Temporal patterns of peak flows had been notoriously modified (having on the average a reduction of 4.7 m3 s-1) in contrast to the small base flow reduction (average reduction of 1.4 m3 s-1) for all watersheds. The Sinaloa and San Fernando basins present the largest human control on river flow and therefore on peak flows. This, in turn, correlates well with the number of man-made reservoirs. Although base flow has been little modified in contrast to peak flow, its importance in arid climates during the dry season is out of the question.
Population is increasing in the region at a mean annual rate of 1% for all five watersheds. At the present, there are currently above 10 M inhabitants on these watersheds and it is expected they would reach above 12 M by the year 2030. This new scenario envisages an increment on water demands of above 20% for municipal and potentially for industrial and irrigation supplies, since these sectors of the economy are well linked to population growth.
Discussion
The river flow regulation by the build up of reservoirs, tapping of deep groundwater resources, municipal discharge, and irrigation excess into streams is consistently noted by the contrasting effect of increased base flows and reduced direct flows of 7% of the studied hydrometric stations. Large reservoirs had been constructed having an important footprint on river flow. The storage capacity has increased exponentially in the last four decades and currently Northern Mexico has an ordinary storage capacity of above 30 000 Mm3, placed in 10 major reservoirs that each has a maximum ordinary storage capacity larger than 1 000 Mm3 in all five watersheds.
The management of natural resources partially contributes to disturb river flow components as well. A coupled effect of increased direct and reduced base flow was observed in several gauging stations. Although there must be other causes that explain this contrasting effect, deforestation and degradation of soil resources appear to play an important role in the promotion of processes leading to reduced base flows (groundwater recharge) and increased direct flows (reduced infiltration and increased Hortonian surface runoff). Land-use changes from native forests to agriculture or grasslands lead to increased surface runoff and soil erosion rates (Návar & Synnott, 2000a; 2000b). Therefore, the disappearance of Tamaulipan thornscrub forests that correlates well with the expansion of intensive agriculture and mining in Mexico's northeastern plains may in turn reduce groundwater recharge.
Deforestation modifies the components of the hydrological cycle and river discharge shows well its fingerprint. The annual deforestation rate of Mexico's northeastern ecosystems approximates 1.7% caused primarily by intensive agriculture and gas mining (Návar-Cháidez, 2008). The deforestation rate approximates 1.70% in the Pacific Ocean coastal Plains, in the RH 10 and 11, in agreement with land-use change studies conducted on tropical dry forests. Temperate forests distribute on the high mountain ranges and have a mean annual deforestation rate of 0.50% (Ramos-Juárez, 2005). In these ecosystems, deforestation practices include overgrazing, forest wildfires, pests and diseases, illegal timber harvesting, unplanned construction of forest roads, among others. The livestock carrying capacity is sometimes between 2 to 5 times higher than the recommended one (Manzano & Návar, 2000; Manzano, Návar, Pando, & Martínez, 2000).
Deforestation practices may also further magnify anomalies of river flow regimes in the near future, since projections under conventional scenarios of the area, previously covered by Tamaulipan thornscrub up to 80 490 km2 in the 1950's (Návar-Cháidez, 2008) would be reduced to 22 000 km2 by the year 2020 and 4 974 km2 by the year 2100. Temperate forests would be reduced 10% for the year 2020 and to 40% by the year 2100 of the total forest area measured for the year 2000. Deforestation practices are expected to further reduce base flow and increase direct and peak flows of most upland watersheds.
Climate change may also partially magnify river flow oscillations. However this control of river discharge variability cannot be properly quantified and we must rely on coarse projections. Mulholland et al. (1997) and IPCC (2014) using global circulation models predicted northeastern Mexico would potentially receive less rainfall (between -5 to -20%) and produce less river flow (between 5-25% less discharge) because of increasing global temperatures. Méndez-González, Návar-Cháidez and González-Ontiveros (2008) have noted statistical evidence that the number of rainy days and total annual rainfall are steadily declining in the last four decades in several climatic stations across northeastern of Mexico.
Currently, climate variability is the most prominent source of river flow anomalies since river flow parameters are well correlated with large scale climate events. Návar-Cháidez and Lizárraga-Mendiola (2013) associated large-scale climate events to discharge variables for a Mexico's northern forested watershed; e.g., the Southern Oscillation Index, SOI was negatively correlated, the PDO was positively correlated and the AMO was negatively correlated with river discharge.
Given this state of understanding, strengthening sustainable management practices of water resources should be addressed in parallel to tapping new sources of river flow in watersheds with high erratic river discharge, high population and industrial growth. Rivers, lakes and aquifers must supply all conventional water (public, household, agricultural, and industrial) and environmental (environmental flow for ecosystems). Because of the erratic frequency of climatic events such as hurricanes, cyclones, and tropical depressions, storage in constructed reservoirs must be increased to collect and store most river flow in times of high availability. In watersheds characterized by long term dry spells and important regional development rates, when storages are depleted, other sources of water supply such as the inter-basin transfer must be sustainably tapped. River flow and groundwater recharge must also be protected in receiving and donor watersheds (Postel, 2000; Schmandt et al., 2000) and the environmental flow must be planned, conveyed and enforced in regulated rivers (Návar-Cháidez, 2014).
Productivity must also be doubled for every drop of water diverted from reservoirs, streams, and aquifers in order to meet conventional supplies as well as to conserve river flow for meeting environmental conservation (Postel, 2000). The current situation and likely future deem scenarios envisages the need for the implementation of the following sustainable practices to enhance sustainable management of water resources: (a) increasing storage by the construction of reservoirs, proper aquifer management, and other strategies such as the implementation of the environmental flow regime of regulated rivers, (b) reducing per capita water use, (c) increasing the efficiency of water use in the municipal, industrial, and the agricultural sector, (d) treating all raw sewages before they are discharged into streams and reservoirs with the objective to avoid the impairment of other sources of water supply and to be reused further below the watershed, (e) valuing correctly water resources, (f) among others.
Conclusions
River flow is in transition in several of Mexico's northern watersheds. Currently, base and direct flow account for approximately 50% each of the total daily river discharge. A little over 40% of each of five river flow parameters presents temporal tendencies with statistical significance for the period of 1940 to 1999. On the average 26% of each of the five parameters evaluated had a negative tendency stressing the river flow decline over time. Climate variability, river flow regulation, deforestation, land degradation, and potentially subtle climatic changes appear to explain the downward temporal monotonic tendencies. It is important to strengthen sustainable management practices of river flow, parallel to sustainable tapping new sources of river flow to meet conventional as well as environmental supplies in watersheds with high regional demographic and economic growth, with the objective of doubling productivity for each drop of water diverted from streams, lakes and aquifers.