Services on Demand
Journal
Article
Indicators
-
Cited by SciELO
-
Access statistics
Related links
-
Similars in SciELO
Share
Revista mexicana de ciencias forestales
Print version ISSN 2007-1132
Rev. mex. de cienc. forestales vol.11 n.62 México Nov./Dec. 2020 Epub Feb 19, 2021
https://doi.org/10.29298/rmcf.v11i62.757
Scientific article
Fungal rot effect on physical and mechanical properties of the bamboo-polypropylene composite
1Ingeniera Forestal. Universidad Nacional Agraria La Molina. Perú
2Departamento de Industrias Forestales, Facultad de Ciencias Forestales, Universidad Nacional Agraria La Molina. Perú
3Departamento de Estudios del Agua y la Energía, Campus Tonalá. Universidad de Guadalajara. México.
Biodegradation resistance of a bamboo-polypropylene composite was evaluated through exposure to two fungi: white-rot Pycnoporus sanguineus and brown-rot Rhodonia placenta (synonym: Postia placenta) for 16 weeks, according to the ASTM D2017-05 norm. Effect of biodegradation on the physical properties of density, absorption and swelling, and mechanical properties of bending, traction and impact were also assessed. The composite was made by extrusion and compression molding using bamboo particles of Guadua angustifolia of two ASTM mesh sizes (40/60 and 60/80) at two bamboo / polypropylene ratios (30/70 and 50/50) and 2 % polypropylene maleic anhydride (MAPP) as a coupling agent. It was probed that the bamboo-polypropylene composite was susceptible to biodegradation by white- and brown-rot fungi; the formulation with smaller proportion of bamboo particles (30 %) exhibited a higher resistance to it. The physical properties of anhydrous density, absorption and swelling and the mechanical properties of static bending and traction were inversely affected by exposure to P. sanguineus and R. placenta, and white and brown rot fungi did not affect the impact property.
Keywords Biodegradation; Guadua angustifolia Kunth; rot fungi; composites; physical properties and mechanical properties
Se evaluó la resistencia a la biodegradación de un material compuesto bambú-polipropileno, mediante la exposición a dos hongos: Pycnoporus sanguineus de pudrición blanca, utilizado en ensayos de durabilidad natural y Rhodonia placenta (sinónimo: Postia placenta) de pudrición café durante 16 semanas, según la norma ASTM D2017-05. Asimismo, se evaluó el efecto de la biodegradación en las propiedades físicas de densidad, absorción e hinchamiento; y las mecánicas de flexión, tracción e impacto. El material compuesto se elaboró por extrusión y posterior moldeo por compresión; para ello se utilizaron partículas de bambú (Guadua angustifolia) de dos tamaños de malla ASTM: 40/60 y 60/80; dos proporciones bambú/polipropileno: 30/70 y 50/50; además de 2 % de anhídrido maleíco de polipropileno (MAPP), como agente acoplante. Se demostró que el compuesto bambú-polipropileno fue susceptible a la biodegradación por hongos de pudrición blanca y café; la formulación con menor proporción de partículas de bambú (30 %) presentó mayor resistencia a la biodegradación. Las propiedades físicas de densidad anhidra, absorción e hinchamiento y las propiedades mecánicas de flexión estática y tracción fueron afectadas de manera inversa a causa de la exposición a P. sanguineus y R. placenta. Los hongos de pudrición blanca y café no incidieron en la propiedad de impacto.
Palabras clave Biodegradación; Guadua angustifolia Kunth; hongos de pudrición; materiales compuestos; propiedades físicas; propiedades mecánicas
Introduction
The interest in wood-plastic composite materials (WPC) has increased in recent years because of their characteristics such as high durability, low maintenance and high profitability. The applications of composites reinforced with natural fibers in panels have an enormous potential and are essential for achieving sustainability; due to their appropriate physical and mechanical characteristics, these fibers are an optimal reinforcement material for WPC (Kalia et al., 2009).
In this sense, fibers of lignocellulosic origin such as rice husk, coconut fibers, sisal and bamboo meet the ecological requirements, since they minimize energy consumption in their manufacture and contribute to the conservation of non-renewable natural resources, since they reduce pollution and, thus, contribute to the maintenance of a healthy environment. Bamboo stands out as a material with enormous economic advantage, as it reaches its full growth in a few months and its maximum mechanical resistance in a few years. In addition, it is abundant in the tropical and subtropical regions of the world (Ghavami, 2005).
In recent years, the use of lignocellulosic materials other than wood in the WPCs, among which bamboo stands out, has become widespread; it is extensively used in the construction industry, in the manufacture of furniture and paper, and in the elaboration of handicrafts. This grass stands out for its rapid growth and for exhibiting high mechanical resistance in comparison to its weight. In Peru, the most commercially used bamboo species is Guadua angustifolia Kunth; it has very resistant fibers with good physical and mechanical properties; for this reason, its study as a reinforcement material in panels made with lignocellulosic fibers is of special interest.
In the first years of marketing of WPCs, they were promoted as resistant to biological attack. However, in the Everglades National Park in Florida, United States of America, evidence of fruiting bodies of rot fungi was found in WPC products exposed during four years, which proved that wood in these materials remained susceptible to biodegradation (Morris and Cooper, 1998). Later studies confirmed this theory (Mankowski and Morrell, 2000; Ibach, 2013; Cárdenas, 2012).
Biodegradation caused by rot fungi affects the lignocellulosic materials in composite materials ―among them, the lignin-degrading Pycnoporus sanguineus (L.) Murrill. and Rhodonia placenta (Fr.) Niemelä K.H. Larss. & Schigel―, resulting in the degradation of cellulose. However, the effects on the physical and mechanical properties of bamboo/plastic composites exposed to these fungi are not known with certainty.
Experience in the use of composite materials has shown their potential deterioration due to exposure to the environment, manifested as changes in color, a loss of aesthetic appeal and mechanical properties, and the development of xylophagous fungi (Stark, 2001; Cárdenas, 2012).
The objectives of this study were to determine the susceptibility to biodegradation caused by white- and brown-rot fungi in bamboo-polypropylene composites, in addition to their effect on physical and mechanical properties.
Materials and Methods
Test specimens of a bamboo-polypropylene composite were elaborated, using particles from the apical part of a 4-year old Guadua angustifolia cane as reinforcement material. A Propilco brand polypropylene (PP) thermoplastic matrix with a fluidity index of 12.5 g.10 min-1 (2.16 kg 230 °C-1) was utilized, with Overac CA100 brand polypropylene maleic anhydride (MAPP) as coupling agent.
The G. angustifolia canes were cut and dried in a Moore oven, up to an average moisture content of 12 %, evaluated with a manual moisture detector. They were then ground (Budapesti Vegypari blade mill, 2 830 rpm) and sieved (Maccinox, 1 700 rpm) in order to obtain particles of two sizes―40/60 and 60/80―, using ASTM meshes. The particles were dried during 48 hours in a Labor Musze Ripari oven at 100 °C to reduce moisture content to an interval of 2 to 3 %. The plastic matrix (PP) and the coupling agent (MAPP) were added for mechanical mixing, prior to extrusion. The extrusion of the materials was carried out in a single-spindle machine until the yarn was obtained, at a temperature ranging between 175 - 185 °C and at 30 rpm; the extruded material (yarn) was sectioned for subsequent pressing and in order to obtain 5 cm long pellets.
The composite panels were formed from pellets from the extruded and sectioned material, using the compression molding method. A hydraulic press (TramelsaTM, capacity 60 t) was used. 64 panels were prepared for the elaboration of test specimens intended for physical and mechanical testing with 21 × 21 × 0.25 cm molds, at a pressure of 40 bars and a speed of 0.9 cm s-1, during 4 minutes, at a temperature ranging between 177 - 199 °C. In addition, four panels were prepared for the biodegradation tests with 12 × 12 × 0.9 cm molds under the same conditions, but were pressed for a longer time: 25 minutes. For the preparation of the specimens, the panels were cut with a laser cutting machine (OrionTM) at a speed of 0.78 mm min-1 and a power of 45 w.
Four formulations were developed considering two particle sizes (S): 40/60 and 60/80, as well as two bamboo/polypropylene ratios (R): 50/50 and 30/70. These formulations were part of the following treatments: without exposure (control); with exposure to moisture only, and with exposure to two types of fungi (F): white-rot Pycnoporus sanguineus and brown-rot Rhodonia placenta (Table 1).
Table 1 Formulationsand treatments of the bamboo-polypropylene composite.
Formulation No. | Particle size (Mesh) |
Bamboo (%) |
Polypropylene (%) |
Coupling agent (%) |
Treatment type |
---|---|---|---|---|---|
F1 | 40/60 | 50 | 48 | 2 | P. sanguineus |
F2 | 40/60 | 30 | 68 | 2 | |
F3 | 60/80 | 50 | 48 | 2 | |
F4 | 60/80 | 30 | 68 | 2 | |
F1 | 40/60 | 50 | 48 | 2 | R. placenta |
F2 | 40/60 | 30 | 68 | 2 | |
F3 | 60/80 | 50 | 48 | 2 | |
F4 | 60/80 | 30 | 68 | 2 | |
F1 | 40/60 | 50 | 48 | 2 | Without exposure |
F2 | 40/60 | 30 | 68 | 2 | |
F3 | 60/80 | 50 | 48 | 2 | |
F4 | 60/80 | 30 | 68 | 2 | |
F1 | 40/60 | 50 | 48 | 2 | Moisture* |
F2 | 40/60 | 30 | 68 | 2 | |
F3 | 60/80 | 50 | 48 | 2 | |
F4 | 60/80 | 30 | 68 | 2 |
*Mechanical properties of bending and tension
The dimensions of the test specimens corresponded to the norms for the tests used in this study―biodegradation, physical properties and mechanical properties―, as shown in Table 2.
Table 2 Types of tests and dimensions of bamboo/polypropylene composite specimens.
Test type | Size of the test specimens | Norm | |||
---|---|---|---|---|---|
Thickness (mm) | Length (mm) | Width (mm) | |||
Biodegradation | 9 ± 0.2 | 25 | 25 | ASTM D2017-05 | |
Physical property | Density | 2.5 ± 0.2 | 76.2 | 25 | ASTM D1037-99 |
Absorption | 2.5 ± 0.2 | 76.2 | 25 | ASTM D570-98 | |
Swelling | 2.5 ± 0.2 | 76.2 | 25 | ASTM D570-98 | |
Mechanical property | Bending | 2.5 ± 0.2 | 50 | 13 | ASTM D790-03 |
Impact | 2.5 ± 0.2 | 120 | 65 | ASTM D5420-10 | |
Tension | 2.5 ± 0.2 | 63.5 | 3.2 | ASTM D638-03 |
Source: ASTM, 1998; ASTM, 1999; ASTM, 2003a; ASTM, 2003b; ASTM, 2005; ASTM, 2010.
772 specimens of the bamboo-polypropylene composite were prepared and used according to the treatment and test type (Table 3).
Table 3 Numberof test specimens of bamboo-polypropylene composite by treatment and test type.
Test type | Replication | Number of test specimens | |||||
---|---|---|---|---|---|---|---|
P. sanguineus | R. placenta | Without exposure (Controls) |
Exposure
to moisture |
Subotal | |||
Biological degradationb | 7 | 28 | 28 | c | d | 56 | |
Subtotal | 56 | ||||||
Physical property | Density | 7 | 28 | 28 | 28 | - | 84 |
Absorption | 7 | 28 | 28 | 28 | - | 84 | |
H. | 7 | 28 | 28 | 28 | - | 84 | |
Subtotal | 252 | ||||||
Mechanical property | Bending | 7 | 28 | 28 | 28 | 28 | 112 |
Tension | 7 | 28 | 28 | 28 | 28 | 112 | |
Impact | 20 | 80 | 80 | 80 | - | 240 | |
Subtotal | 464 | ||||||
Total | 772 |
aReplication of 7 test specimens for each of the 4 formulations, with the exception of impact (20 specimens); b7 untreated bamboo control samples were used; cPhysical and mechanical test specimens were used to evaluate the initial values in the different formulations without any exposure (to moisture or fungi); dSpecimens of suitable dimensions for bending and impact tests were used to evaluate the exclusive influence of humidity, without fungi.
The biodegradation test was performed by means of soil-block trials in keeping with the ASTM D 2017-05 norm (ASTM, 2005); the weight loss of the bamboo-polypropylene composite subjected to the action of the fungi Pycnoporus sanguineus and Rhodonia placenta was recorded during 16 weeks for each formulation. In this test, 56 specimens of the bamboo-polypropylene composite material were used. Additionally, 14 samples of bamboo cane without previous treatment were used as control.
In parallel, the composite material samples of each formulation and of the final dimensions were subjected to biodegradation during 16 weeks for physical and mechanical testing (Figure 1). After exposure to the fungi, their physical and mechanical properties were evaluated. To this end, glass jars with the capacity to contain the samples required for these tests were prepared.
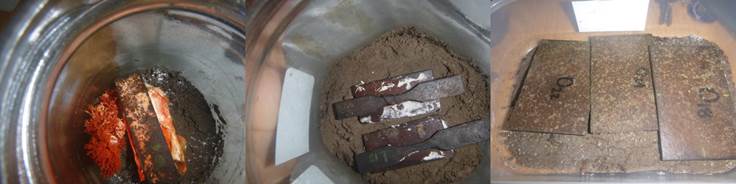
Figure 1 Specimens exposed to Pycnoporus sanguineus (L.) Murrill. (left), Rhodonia placenta (Fr.) Niemelä K.H. Larss & Schigel (center), and moisture (right).
The physical properties of anhydrous density, absorption in weight increase and swelling were tested according to the ASTM D1037-99 (ASTM, 1999) and ASTM D570-98 (ASTM, 1998)norms; out of a total of 252 bamboo-propylene specimens, 168 were exposed to the fungi, while 84 control specimens had no exposure to the rot fungi (Figure 1).
Static bending, tension and impact mechanical properties tests were performed according to ASTM D790-03, ASTM D638-03 and ASTM D5420-04 standards, respectively (ASTM, 2003a; ASTM, 2003b; ASTM, 2010). A total of 136 control specimens without exposure to rot fungi, 272 specimens of a bamboo-polypropylene composite exposed to rot fungi and 56 specimens exposed only to moisture were used, adding up to a total of 464 specimens.
Statistical analysis
The statistical model used was a completely randomized block design (DBCA), with a 2 × 2 factorial arrangement (bamboo particle size and bamboo/polypropylene ratio), with seven repetitions for the weight loss, anhydrous density, absorption, swelling, tension and static bending tests, and 20 for impact testing. The design was as follows:
Where:
µ = General mean
An analysis of variance was applied with the Statistical Analysis System software, version 9.2 (SAS, 2008). When differences between treatments were observed (p<0.05), the Tukey mean comparison test was applied.
Results and Discussion
Biodegradation of bamboo-polypropylene composites
Figure 2 shows the weight loss of bamboo control and bamboo-polypropylene composite exposed to Pycnoporus sanguineus and Rhodonia placenta. The weight loss values of the bamboo-polypropylene composite ranged between 3.3 and 4.9 % for P. sanguineus and from 2.3 to 3.4 % for R. placenta. The highest weight loss was recorded in the composites with the higher proportion of bamboo particles (50/50) and the smaller particle size (-60/+80). Likewise, for the bamboo cane control and the bamboo-polypropylene composite material, a higher weight loss was observed in the presence of P. sanguineus.
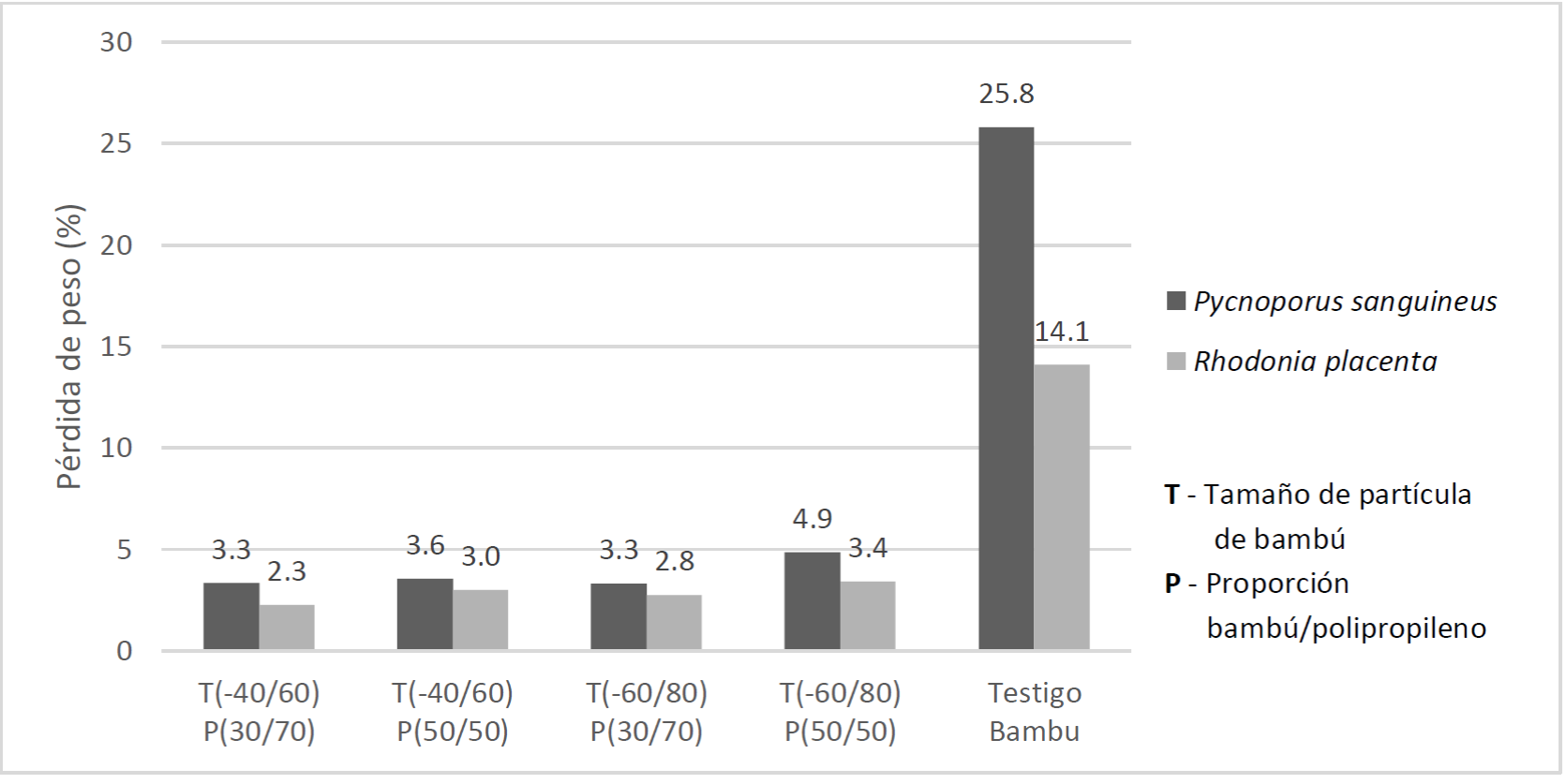
Pérdida de peso = Weight loss; Testigo = Control; Tamaño de partícula = Particle size; Proporción bambú/polipropileno = Bamboo-polypropylene ratio.
Figure 2 Biodegradation of bamboo-polypropylene composite.
Regarding the ratio (R), the weight loss caused by both fungal species was observed to increase with higher proportions of bamboo particles in the composite, because there is a greater availability of the organic material for the colonization by and development of the fungi. These results are widely consistent with what has been cited in several studies (Pendleton et al., 2002; Lomelí et al., 2009; Kartal et al., 2013; Candelier et al., 2018).
In regard to the particle size variable (S), there was a possible greater weight loss caused by P. sanguineus in composites with the smaller bamboo particle size; this can be supported by the results documented by Kartal et al. (2013) and well as by the study by Lomelí et al. (2009), who claim that smaller particles have a better degree of distribution and increase the specific surface area susceptible to infestation by fungi.
These results indicate that the bamboo particles are not fully encapsulated by the polymer matrix, which affects the biodegradation of the material; this agrees with the statement of Klyosov (2007), namely: that when composite materials contain a significant amount of cellulose, there are enough particles to form extended chains in contact, along which water can permeate most of the material, causing its microbial contamination in the pores and conduits of the matrix, as well as degradation of the cellulosic matter.
Regarding the type of fungus (F), white-rot P. sanguineus was proven to produce more biodegradation than brown-rot R. placenta. This comes from the ability of P. sanguineus to degrade the main components of the cell wall, as pointed out by Encinas and Mora (2003). These authors describe the degradation patterns of the white-rot fungi Trametes versicolor (L ex Fr) Pilat. and P. sanguineus in Caribbean pine woods, in whose trees they simultaneously attack the main components of the cell wall: cellulose, hemicellulose and lignin. Likewise, Catto et al. (2016) point out that white-rot fungi contain such enzymes as lignin peroxidase, laccase and manganese peroxidase, which catalyze the deterioration, through the diffusion of oxidizing agents or specific mediators, which for the most part degrade lignin and in some cases degrade both lignin and cellulose.
Brown-rot fungi, as cited by Mankowski and Morrell (2000), Fabiyi et al. (2011), Ibach (2013), and Candelier et al. (2018), contain endoglucanases which tend to degrade cellulose and hemicellulose primarily, so that there is a limited decrease in lignin content, and greater efficiency in the degradation, predominantly of carbohydrates, in conifers (Irbe et al., 2006).
The analysis of statistical variance (ANOVA) showed that the type of fungus (F) and ratio (R) variables were highly significant (with a p-value of 0.0002 and 0.0011, respectively); the particle size variable (S) was also significant (p-value = 0.0176).
Density
Figure 3 shows the density of the bamboo-polypropylene composite; in those exposed to the fungi, the lowest value (0.65 g.cm-3) corresponded to P. sanguineus, with a particle size of 40/60 and a 50/50 bamboo/polypropylene ratio, which is consistent with its higher degradation. Regarding R. placenta, the highest value was 0.89 g.cm-3, with a 60/80 particle size and a 30/70 bamboo/polypropylene ratio. Likewise, the results for bamboo-polypropylene composites with exposure to fungi were lower than those of the control; which shows that biodegradation by fungi in the composite materials affected the density of the composite.
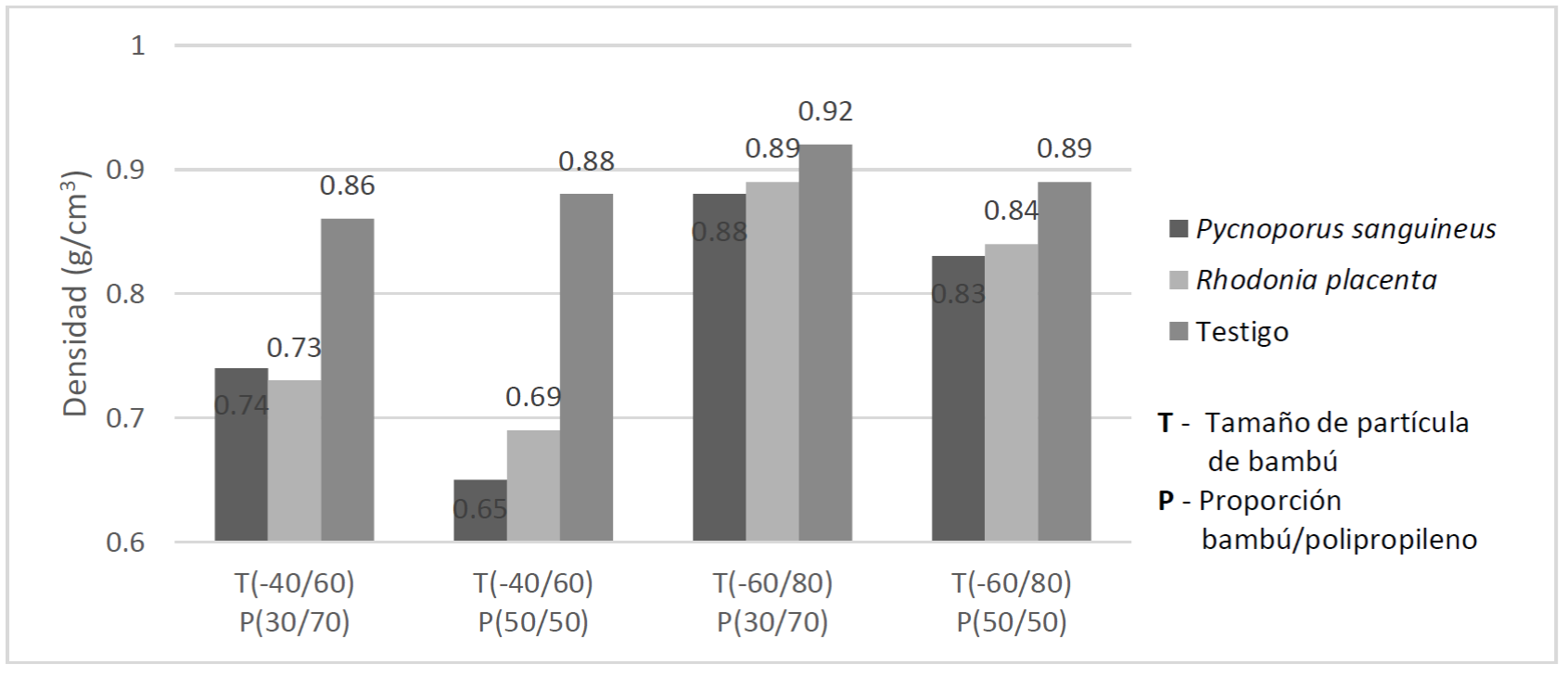
Densidad = Density; Testigo = Control; Tamaño de partícula = Particle size; Proporción bambú/polipropileno = Bamboo-polypropylene ratio.
Figure 3 Density of bamboo-polypropylene composite exposed to rot fungi.
The results showed that bamboo-polypropylene composites with exposure to rot fungi and smaller particle size had the highest density values because smaller particles have a better degree of distribution and encapsulation in the polymeric matrix, which reduce porosity and increase density (Verhey and Laks, 2002; Klyosov, 2007). Also, as the content of bamboo particles in these composites increases, the porosity increases and the density diminishes (Lomelí et al., 2009; Lázaro et al., 2016a, and Córdova et al., 2020).
Based on Figure 3, the densities registered with the 50/50 bamboo/polypropylene ratio (<0.84 g.cm-3) are lower than those documented by Schirp and Wolcott (2005), with 1.05 g.cm-3; by Cárdenas (2012), with 1.06 g.cm-3, and by Kartal et al. (2013), with 1.08 g.cm-3 in specimens unexposed to fungi. This shows that the composites under study had higher porosity levels, which had an impact on the lower density values, possibly because in the present research they were made by extrusion and compression; while in the cited studies the final process was by injection.
The analysis of statistical variance (ANOVA) showed that the variables particle size (S) and ratio (R) were highly significant (p-value < 0.0001).
Absorption
Figure 4 shows the absorption level attributed to the increase in weight of the bamboo-polypropylene composite. Bamboo-polypropylene composites with a smaller particle size (60/80), a 30/70 bamboo/polypropylene ratio, and exposure to R. placenta had the lowest value (7.4 %), while the highest (29.3 %) was recorded in composites with the larger particle size 40/60, a 50/50 bamboo/polypropylene ratio, and exposure to P. sanguineus. These results support the greater influence of the white-rot fungus on the physical property of absorption because the biodegradation was higher (Cárdenas, 2012). Also, the water absorption in the bamboo - polypropylene composites without exposure (control) was evidently lower compared to the composites exposed to the two species of fungi, since penetration by them increased water absorption through the cracks or the greater empty spaces resulting from the separation of the bamboo from the plastic (Steckel et al., 2006; Klyosov, 2007).

Absorción = Absorption; Testigo = Control; Tamaño de partícula = Particle size; Proporción bambú/polipropileno = Bamboo-polypropylene ratio.
Figure 4 Absorption of bamboo - polypropylene composite exposed to rot fungi.
In addition, bamboo-polypropylene composites with larger particle size and exposure to rot fungi exhibited the highest values for water absorption, which was favored by the larger hydrophilic area exposed to moisture (Figure 5) (Verhey and Laks, 2002; Bouafif et al., 2009; Lázaro et al., 2016a). On the other hand, small particles are more effectively encapsulated in the polymer matrix, which reduces the empty spaces for the circulation of water and, therefore, water absorption (Stark, 2001; Wang and Morrel, 2004).
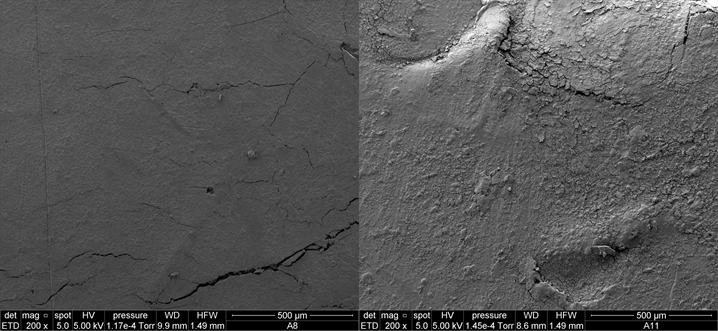
60/80 (left) and 40/60 particle size (right)
Figure 5 Samples subjected to absorption with a 30/70 bamboo/polypropylene ratio after exposure to Rhodonia placenta (Fr.) Niemelä K.H. Larss. & Schigel.
Bamboo - polypropylene composites with exposure to rot fungi with a higher content of bamboo particles showed higher water absorption values, due to the increase of their hydroxyl groups and because of their hydrophilic nature (Westman et al., 2010; Ibach, 2013); in addition, an incompatibility was generated between the particles and the polymeric matrix that originated empty spaces and cracks through which the water penetrated, as pointed out by Klyosov (2007) and Bouafif et al. (2009). That is, the increment of bamboo particles in bamboo-polypropylene composite materials increased water absorption values (Mankowski and Morrell, 2000; Verhey and Laks, 2002; Barton et al., 2017; Candelier et al., 2018).
The absorption values determined for the 50/50 bamboo/polypropylene ratio (>17.9 %) for the two particle sizes exposed to white and brown rot were higher than those reported by Hosseinihashemi et al. (2011), of 9.1 and 10.0 %; by Kartal et al. (2013), of 16.6 %, and by Cárdenas (2012), of 5.1 %; all these authors worked with similar particle proportions and sizes, but with different manufacturing methods.
The analysis of statistical variance (ANOVA) showed that the particle size (S) and ratio (R) variables were highly significant (p-value < 0.0001 for both).
Swelling
In the bamboo-polypropylene composites with exposure, the lowest value, 0.9 %, corresponded to the smaller particle size (60/80), with a 30/70 bamboo/polypropylene ratio and exposure to R. placenta (Figure 6). The highest value, 2.9 %, was for the same particle size and fungal species, but with a bamboo/polypropylene ratio of 50/50 (Figure 6). Also, the swelling of the bamboo-polypropylene composites in the control was lower, compared to the composites with exposure (Figure 6). This can be explained from the penetration of the rot fungi that caused a greater water absorption, due to the cracks or larger empty spaces produced by the separation of the bamboo from the plastic (Steckel et al., 2006; Klyosov, 2007).
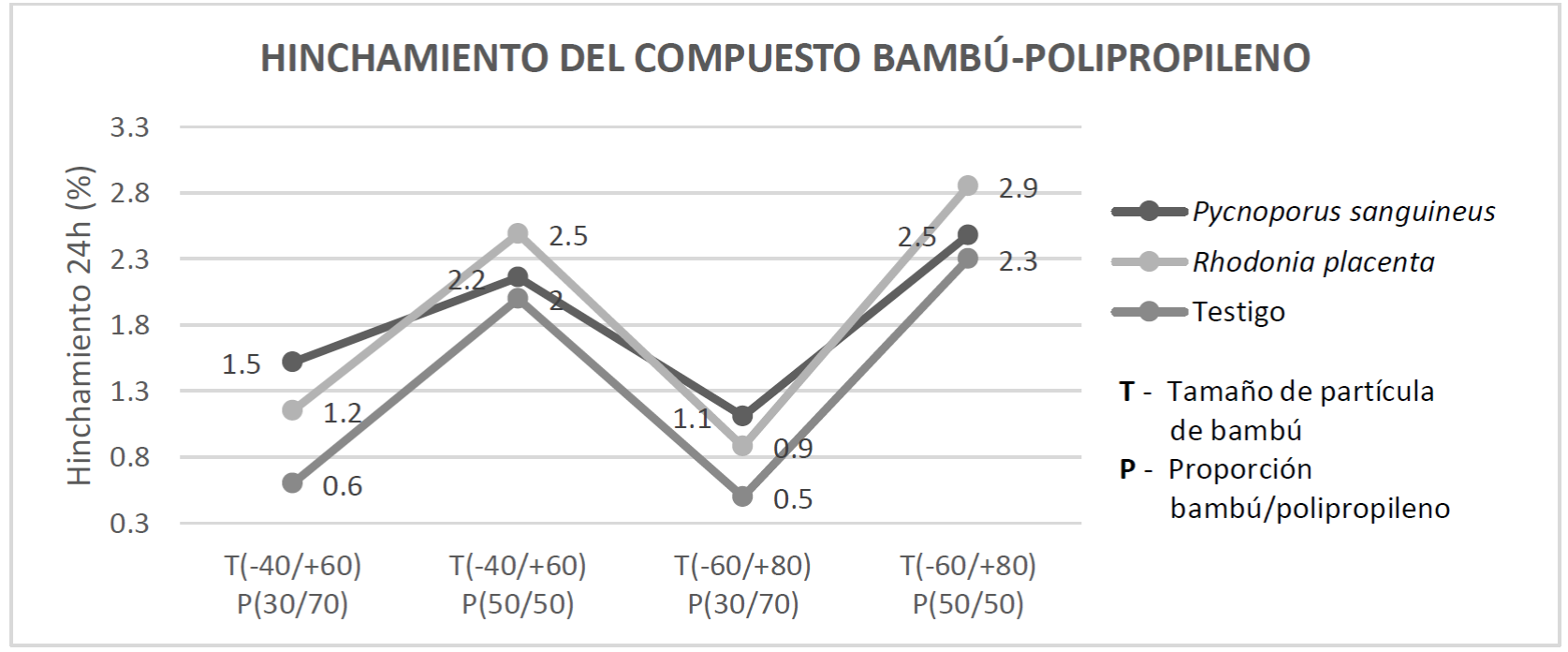
Hinchamiento = Swelling; Testigo = Control; Tamaño de partícula = Particle size; Proporción bambú/polipropileno = Bamboo-polypropylene ratio.
Figure 6 Swelling of bamboo - polypropylene composite exposed to rot fungi.
Results in Figure 6 show that, after exposure to rot fungi, bamboo-polypropylene composites with the higher content of bamboo particles registered the highest values for swelling, in response to the increase of the hydroxyl groups of bamboo and because of its hydrophilic nature; this is consistent with the indications of several authors (Westman et al., 2010; Mankowski and Morrell, 2000; Verhey and Laks, 2002). When the bamboo particles swell, microcracks formed between the particles and the polymer matrix, creating porosity and cracks where the water penetrates (Bouafif et al., 2009).
Both, a larger particle size and a higher ratio were used; this originated a larger hydrophilic surface area exposed to moisture, which increased swelling. The opposite occurred with smaller percentages and particle sizes (Figure 7) (Verhey and Laks, 2002; Wang and Morrel, 2004; Bouafif et al., 2009). Thus, the degree of degradation by fungi depends on the bamboo content in the composites; that is, in the composites with more bamboo particles, there was a greater penetration of fungi, which favored the separation of the bamboo from the plastic, and this, in turn, caused greater porosity or cracks (Steckel et al., 2006; Klyosov, 2007) and the swelling of the composites to increase.
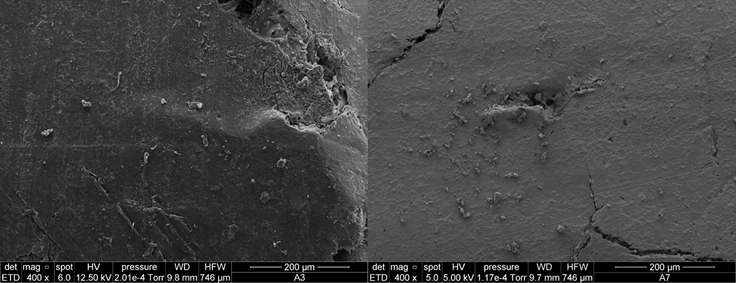
Bamboo/polypropylene ratios of 50/50 (left) and 30/70 (right)
Figure 7 Samples subjected to swelling after exposure to Pycnoporus sanguineus (L.) Murrill., with particle size 60/80.
The swelling values for the 50/50 bamboo/polypropylene ratio (<2.9 %) and the two particle sizes exposed to rot fungi (Figure 6) were lower than those documented by Kartal et al. (2013), of 9.4 % and 9.7 %, and those cited by Cárdenas (2012), of 10.0 %. These authors worked with similar proportions and particle sizes, and the exposure to white and brown rot fungi lasted 16 weeks; this difference in values comes from the fact that the immersion time of the test specimens in the present study was 24 hours, whereas in the aforementioned studies it was longer (30 and 200 days, respectively); however, the results documented herein are close to those obtained by Hosseinihashemi et al. (2011), of 3.6 % and 4.1 %, with an immersion time of the test specimens also of 24 hours.
The statistical analysis of variance (ANOVA) showed that the ratio (R) variable was highly significant (p-value < 0.0001), and the double interactions between type of fungus and the ratio (F*R), as well as between the particle size and the ratio (S*R) were significant (p-value = 0.0411 and 0.034, respectively).
Static bending
Figure 8 shows the maximum static bending strength of bamboo/polypropylene composite without exposure (control), with exposure to moisture, and with exposure to two rot fungi. In the bamboo/polypropylene composites with exposure, 23.7 MPa was the highest value and corresponded to the composite with the smaller particle size (60/80), a bamboo/polypropylene ratio of 30/70, and exposure to R. placenta; the lowest value (10.4 MPa) was registered in the composite with the larger particle size (40/60), a bamboo/polypropylene ratio of 50/50, and exposure to P. sanguineus.

Resistencia máxima en flexión = Maximum bending strength; Testigo = Control; Tamaño de partícula = Particle size; Proporción bambú/polipropileno = Bamboo-polypropylene ratio.
Figure 8 Maximum bending strength of bamboo-polypropylene composite exposed to two rot fungal species.
These results revealed that the particles of the bamboo-polypropylene composite exposed to rot fungi are smaller than the control composites and than the 15 to 22.5 MPa recorded by Lázaro et al. (2016b), who used the same particle sizes and proportions without exposure. This shows that exposure to rot fungi has an inverse relationship to maximum bending strength; this is supported by the evaluation of mechanical properties after exposure to fungi by Stark (2001), Schirp and Wolcott (2005), Ibach (2013), and Candelier et al. (2018). Also, the results of the test specimens exposed to moisture were close to those of the test specimens exposed to the fungi, which indicates that moisture may have a similar influence to that of the presence of such organisms (Figure 9), consistently with the results cited by Cárdenas (2012).

Figure 9 Samples with a 60/80 particle size, subjected to the action of moisture (left) and of Pycnoporus sanguineus (L.) Murrill. (right).
Likewise, the bamboo-polypropylene composites with smaller particle size exhibited the highest values of maximum resistance to bending because, depending on the size of the particles, there is a degree of encapsulation in the polymeric matrix; i.e. the small particles are more effectively encapsulated (Verhey and Laks, 2002).
Figure 8 shows that, in bamboo-polypropylene composites, the increase in bamboo particle content decreased the maximum static bending strength, which is in line with the findings of Schirp and Wolcott (2005), Kartal et al. (2013) and Córdova et al. (2020).
The analysis of variance (ANOVA) showed that the particle size (S) and bamboo/polypropylene ratio (R) variables were highly significant (p-value = 0.0001 and < 0.0001, respectively), and that the fungus type variable (F) was significant (p-value of 0.0137).
Tension
In bamboo-polypropylene composites with exposure, 15.0 MPa was observed to be the highest value for composites containing the smaller particles (60/80), with a 30/70 bamboo/polypropylene ratio and exposure to R. placenta (Figure 10); the lowest value (7.7 MPa) was obtained for composites with the larger particle size (40/60), a 50/50 bamboo/polypropylene ratio, and the presence of R. placenta (Figure 10).

Resistencia máxima en tensión = Maximum tensile strength; Testigo = Control; Tamaño de partícula = Particle size; Proporción bambú/polipropileno = Bamboo-polypropylene ratio.
Figure 10 Maximum tensile strength of bamboo- polypropylene composite exposed to rot fungi.
Bamboo-polypropylene composites with exposure to rot fungi had lower values than the unexposed composites (control); the same happens when compared with the results obtained by Lázaro et al. (2016b) , who reported values between 16 and 17.8 MPa for the same particle sizes and ratios without exposure. This would prove that exposure to rot fungi inversely influences the maximum resistance to tension, which is supported by several studies in which losses of mechanical properties are registered after exposure to biodegrading fungi (Stark, 2001; Cárdenas, 2012; Ibach, 2013; Candelier et al., 2018; Teixeira et al., 2018). However, the recorded values are close to those of the samples exposed to moisture only, demonstrating the inverse influence of moisture on the tension property.
The bamboo-polypropylene composites with the smaller particle size exhibited a higher maximum resistance to tension for both rot fungi, since the small particles are more effectively encapsulated by the polymeric matrix (Verhey and Laks, 2002), increasing this mechanical property. The decrease of bamboo particle content augments the maximum tensile strength values (Lu et al., 2005). Therefore, the bamboo - polypropylene composites elaborated with lower proportions of bamboo (30 %) had higher values for maximum resistance to traction.
The analysis of variance (ANOVA) showed that the particle size (S) and ratio (R) variables were highly significant (p-value < 0.0001 for both).
Impact
In the bamboo-polypropylene composites exposed to the fungal species, the highest value (0.53 J) was observed to correspond to the 30/70 bamboo/polypropylene ratio for both particle sizes and both rot fungi (Figure 11). The lowest values (0.33 J) were recorded for the 50/50 bamboo/polypropylene ratio, with the largest particle size (40/60) and exposure to P. sanguineus. This is consistent with the highest degradation of the bamboo/polypropylene composite material. Likewise, these results were superior to those of the control composites, which proves that this mechanical property was not affected by the biodegradation of the rot fungi in the composite materials.

Energía = Energy; Testigo = Control; Tamaño de partícula = Particle size; Proporción bambú/polipropileno = Bamboo-polypropylene ratio.
Figure 11 Impact of bamboo-polypropylene composite exposed to rot fungi
The values of bamboo-polypropylene composites exposed to rot fungi were lower than those quoted by Cárdenas (2012), who estimated an average value of 0.6 J for samples made by extrusion and injection and exposed to rot fungi. Likewise, Lázaro et al. (2016b) documented values between 0.39 and 0.44 J with similar particle sizes and ratios, but without exposure to fungi and using the same manufacturing method. This provides evidence of a greater influence of the manufacturing method of the composite material on the impact mechanical property. However, the mechanical property of impact is influenced by prolonged exposure such as weathering (Fuentes et al. 2014).
In the bamboo-polypropylene composites, higher bamboo particle contents reduced the values for the impact property, indicating that polypropylene is the main responsible for resistance to impact. Particle size did not have a significant influence.
The analysis of variance (ANOVA) showed that the proportion variable (P) was highly significant (p-value < 0.0001).
Statistical analysis
Table 4 shows the values obtained and the standard deviation of the weight loss variables, as well as the physical properties of anhydrous density, absorption and swelling.
Table 4 Estimated values and standard deviation of physical properties.
Fungus | Particle size (Mesh) |
Bamboo/ plastic ratio |
Weight loss % [s.d.] |
Density (g cm-3) |
A (%) | S (%) |
---|---|---|---|---|---|---|
P. sanguineus | -40/+60 | 50/50 | 3.56 [1.11] | 0.65 [0.07] | 29.36 [5.91] | 2.16 [0.46] |
P. sanguineus | -40/+60 | 30/70 | 3.32 [0.59] | 0.74 [0.05] | 14.28 [4.92] | 1.52 [0.54] |
P. sanguineus | -60/+80 | 50/50 | 4.85 [0.67] | 0.83 [0.05] | 23.26 [4.25] | 2.48 [0.25] |
P. sanguineus | -60/+80 | 30/70 | 3.32 [0.92] | 0,88 [0.04] | 7.67 [1.91] | 1.11 [0.34] |
R. placenta | -40/+60 | 50/50 | 2.98 [1.01] | 0.69 [ 0.05] | 27.07 [4.42] | 2.49 [0.67] |
R. placenta | -40/+60 | 30/70 | 2.26 [0.26] | 0.73 [0.06] | 14.01 [5.75] | 1.15 [0.37] |
R. placenta | -60/+80 | 50/50 | 3.41 [0.90] | 0.84 [0.05] | 17.90 [4.30] | 2.85 [0.14] |
R. placenta | -60/+80 | 30/70 | 2.76 [0.61] | 0.89 [0.02] | 7.36 [1.27] | 0.88 [0.59] |
None | -40/+60 | 50/50 | 0.88 [0.06] | 13.2 [1.18] | 2.0 [0.36] | |
None | -40/+60 | 30/70 | 0.86 [0.03] | 4.8 [0.64] | 0.6 [0.39] | |
None | -60/+80 | 50/50 | 0.89 [0.04] | 12.12 [1.64] | 2.3 [0.57] | |
None | -60/+80 | 30/70 | 0.92 [0.04] | 4.2 [0.41] | 0.5 [0.31] |
A = Absorption; S = Swelling.
Table 5 shows the values and standard deviation of the variables corresponding to the mechanical properties.
Table 5 Estimated values and standard deviation of mechanical properties.
Exposure Factor | Particle size (Mesh) |
Bamboo /plastic ratio |
Static bending |
Tension | Impact |
---|---|---|---|---|---|
Maximum resistance (Mpa) |
Maximum
R. (Mpa) |
Energy (J) |
|||
P. sanguineus | -40/+60 | 50/50 | 10.41 [2.92] | 8.03 [1.18] | 0.33 [0.04] |
P. sanguineus | -40/+60 | 30/70 | 17.62 [3.34] | 12.64 [1.98] | 0.53 [0.13] |
P. sanguineus | -60/+80 | 50/50 | 13.58 [3.31] | 10.36 [0.73] | 0.34 [0.11] |
P. sanguineus | -60/+80 | 30/70 | 21.88 [3.08] | 14.99 [2.65] | 0.48 [0.08] |
R. placenta | -40/+60 | 50/50 | 11.87 [3.02] | 7.70 [0.63] | 0.38 [0.09] |
R. placenta | -40/+60 | 30/70 | 20.89 [3.95] | 12.55 [1.47] | 0.50 [0.08] |
R. placenta | -60/+80 | 50/50 | 13.91 [3.52] | 12.09 [1.21] | 0.34 [0.05] |
R. placenta | -60/+80 | 30/70 | 25.29 [1.38] | 15.38 [1.59] | 0.53 [0.07] |
Moisture | -40/+60 | 50/50 | 12.10 [4.91] | 8.20 [0.68] | |
Moisture | -40/+60 | 30/70 | 21.81 [2.12] | 13.80 [1.87] | |
Moisture | -60/+80 | 50/50 | 17.05 [3.69] | 9.30 [0.90] | |
Moisture | -60/+80 | 30/70 | 23.68 [1.74] | 13.04 [1.51] | |
Control | -40/+60 | 50/50 | 13.78 [3.10] | 10.62 [3.13] | 0.3 [0.09] |
Control | -40/+60 | 30/70 | 25.40 [2.27] | 14.07 [2.35] | 0.4 [0.05] |
Control | -60/+80 | 50/50 | 22.00 [4.55] | 13.62 [1.41] | 0.4 [0.05] |
Control | -60/+80 | 30/70 | 27.42 [4.77] | 16.47 [3.66] | 0.4 [0.07] |
Table 6 summarizes the analysis of variance (ANOVA) values for durability, weight loss, density, absorption, and swelling; and Table 7 shows the values for the mechanical properties of maximum strength in static bending, modulus of elasticity in static bending, maximum stress and impact load of bamboo-plastic composites exposed to biodegradation. Finally, Table 8 shows the values of the multiple mean comparison for the same variables using Tukey’s test.
Table 6 Analysis of Variance (ANOVA) values for durability and physical testing.
Source of variability |
Degrees of freedom (D.F.) |
Durability | Density | Absorption (24 hours) |
Swelling | ||||
---|---|---|---|---|---|---|---|---|---|
p-value | Sig. | p-value | Sig. | p-value | Sig. | p-value | Sig. | ||
F | 1 | 0.0002 | ** | 0.2316 | n.s. | 0.0756 | n.s. | 0.8684 | n.s. |
S | 1 | 0.0176 | * | <0.0001 | ** | <0.0001 | ** | 0.9957 | n.s. |
R | 1 | 0.0011 | ** | <0.0001 | ** | <0.0001 | ** | <0.0001 | ** |
F*S | 1 | 0.683 | n.s. | 0.9542 | n.s. | 0.4961 | n.s. | 0.7689 | n.s. |
F*R | 1 | 0.6554 | n.s. | 0.3603 | n.s. | 0.1255 | n.s. | 0.0411 | * |
S*R | 1 | 0.1748 | n.s. | 0.3909 | n.s. | 0.6604 | n.s. | 0.034 | * |
F*S*R | 1 | 0.1343 | n.s. | 0.4917 | n.s. | 0.5079 | n.s. | 0.8475 | n.s. |
Error | 42 | 0.0022 | <0.0001 | <0.0001 | <0.0001 | ||||
Corrected total | 55 | ||||||||
C.V. | 25.34 | 5.92 | 24.04 | 29.3 |
F = Main effect of the fungus type factor; S: main effect of particle size factor; R = Main effect of bamboo/plastic ratio factor; F*S = Effect of the double interaction between fungus type and particle size; F*R = Effect of the double interaction between fungus type and bamboo/plastic ratio; S*R = Effect of the double interaction between particle size and bamboo/plastic ratio; F*S*R = Effect of the triple interaction between fungus type, particle size and bamboo/plastic ratio; C.V. = Coefficient of variability; n.s. = Not significant; * = Significant; ** = Very significant.
Table 7 Analysis of Variance (ANOVA) values for mechanical tests.
Source of vari ability |
Degrees of freedom (D.F.) |
Bending (Rmax) |
Tension | Impact | |||
---|---|---|---|---|---|---|---|
p-value | Sig. | p-value | Sig. | p-value | Sig. | ||
F | 1 | 0.0137 | * | 0.4308 | n.s. | 0.4109 | n.s. |
S | 1 | 0.0001 | ** | <0.0001 | ** | 0.3668 | n.s. |
R | 1 | <0.0001 | ** | <0.0001 | ** | <0.0001 | ** |
F*S | 1 | 0.7646 | n.s. | 0.2315 | n.s. | 0.3688 | n.s. |
F*R | 1 | 0.1447 | n.s. | 0.6561 | n.s. | 0.437 | n.s. |
S*R | 1 | 0.3012 | n.s. | 0.3877 | n.s. | 0.7577 | n.s. |
F*S*R | 1 | 0.6997 | n.s. | 0.3182 | n.s. | 0.1245 | n.s. |
Error | 42 | <.0001 | Gl = 37 | <.0001 | Gl = 37 | 0.0011 | |
Corrected total | 55 | Corrected total = 50 | Corrected total = 50 | ||||
C.V. | 18.19 | 13.51 | 21.61 |
F = Main effect of the fungus type factor; S = Main effect of particle size factor; R = Main effect of bamboo/plastic ratio factor; F*S = Effect of the double interaction between fungus type and particle size; F*R = Effect of the double interaction between fungus type and bamboo/plastic ratio; S*R = Effect of the double interaction between particle size and bamboo/plastic ratio; F*S*R = Effect of the triple interaction between fungus type, particle size and bamboo/plastic ratio; C.V. = Coefficient of variability; n.s. = Not significant; * = Significant; ** = Very significant.
Table 8 Values of the multiple mean comparison estimated using Tukey’s test.
Variable | Fungus type | Particle size | Bamboo/plastic ratio | ||||||
---|---|---|---|---|---|---|---|---|---|
Level | Mean | Tukey | Level | Mean | Tukey | Level | Mean | Tukey | |
Weight loss | Ps | 3.76 | A | -40/+60 | 3.03 | B | 50/50 | 3.70 | A |
Rp | 2.85 | B | -60/+80 | 3.59 | A | 30/70 | 2.92 | B | |
Density | Ps | 0.77 | A | -40/+60 | 0.70 | B | 50/50 | 0.75 | B |
Rp | 0.79 | A | -60/+80 | 0.86 | A | 50/50 | 0.81 | A | |
Absorption | Ps | 18.65 | A | -40/+60 | 21.18 | A | 50/50 | 24.40 | A |
Rp | 16.58 | A | -60/+80 | 14.05 | B | 30/70 | 10.83 | B | |
Swelling | Ps | 1. 82 | A | -40/+60 | 1.83 | A | 50/50 | 2.50 | A |
Rp | 1.84 | A | -60/+80 | 1.83 | A | 30/70 | 1.17 | B | |
Bending (Rmax) | Ps | 15.87 | B | -40/+60 | 15.20 | B | 50/50 | 12.44 | B |
Rp | 17.98 | A | -60/+80 | 18.66 | A | 30/70 | 21.42 | A | |
Tension | Ps | 5.85 | A | -40/+60 | 5.16 | B | 50/50 | 4.82 | B |
Rp | 5.95 | A | -60/+80 | 6.62 | B | 50/50 | 6.95 | A | |
Impact | Ps | 0.42 | A | -40/+60 | 0.44 | A | 50/50 | 0.34 | B |
Rp | 0.42 | -60/+80 | 0.41 | A | 30/70 | 0.50 | A |
Conclusions
Bamboo-polypropylene composites are affected by Pycnoporus sanguineus (3.3 %) and Rhodonia placenta (2.2 %). The highest weight loss in the composites occurs with a 50/50 ratio of bamboo/polypropylene particles.
Biodegradation in bamboo-polypropylene composite materials reduces the values for anhydrous density, maximum resistance to static bending and maximum resistance to tension. However, it increases the values for absorption, swelling and impact.
In general, the 60/80 particle size registers higher values than the 40/60 size for anhydrous density, maximum resistance to static bending and tension. On the other hand, the smaller particle size (60/80) exhibits lower water absorption values.
After the exposure to fungi, a higher ratio (50/50) of bamboo to plastic particles in the composite results in lower values for impact and maximum resistance to bending, while a larger amount of plastic (30/70) increases the values for maximum resistance to tension, swelling, and water absorption.
Acknowledgements
The authors wish to thank the National Innovation Program for Competitiveness and Productivity, INNOVATE PERU, for supporting and financing this research through project 414-PNICP-PIAP-2014. They are grateful to Javier Gago Campusano, of the Peruvian Institute of Nuclear Energy (Instituto Peruano de Energía Nuclear, IPEN), for his support in the extrusion of the materials.
REFERENCES
American Society for Testing and Materials (ASTM). 1998. Standard Test Method for Water Absorption of Plastic. ASTM D 570 - 98. West Conshohocken, PA, USA. 4 p. [ Links ]
American Society for Testing and Materials (ASTM). 1999. Standard Test Methods for Evaluating Properties of Wood Base Fiber and Particle Panels Materials ASTM D 1037 - 99. West Conshohocken, PA, USA. 30 p. [ Links ]
American Society for Testing and Materials (ASTM). 2003a. Standard Test Methods for Flexural Properties of Unreinforced and Reinforced Plastics and Electrical Insulating Materials ASTM D790-03. West Conshohocken, PA, USA 11 p. [ Links ]
American Society for Testing and Materials (ASTM). 2003b. Standard Test Method for Tensile Properties for Plastics. ASTM D 638 - 03. West Conshohocken, PA, USA.15 p. [ Links ]
American Society for Testing and Materials (ASTM). 2005. Standard Method of Accelerated Laboratory Test of Natural Decay Resistance of Woods (Withdrawn 2014). ASTM D2017-05. West Conshohocken, PA, USA 5 p. [ Links ]
American Society for Testing and Materials (ASTM). 2010. Standard Test Method for Impact Resistance of Flat, Rigid Plastic Specimen by Means of a Striker Impacted by a Falling Weight (Gardner Impact). ASTM D5420-04. West Conshohocken, PA, USA 8 p. [ Links ]
Barton, J., K. Czaja, M. Grzymek and J. Lipok. 2017. Evaluation of wood-polyethylene composites biodegradability caused by filamentous fungi. International Biodeterioration & Biodegradation 118 (1) 10-18. Doi: 10.1016/j.ibiod.2017.01.014. [ Links ]
Bouafif, H., A. Koubaa, P. Perré and A. Cloutier. 2009. Effects of fiber characteristics on the physical and mechanical properties of wood plastic composites. Composites: Part A Applied Science and Manufacturing 40: 1975-1981. Doi: org/10.1016/j.compositesa.2009.06.003. [ Links ]
Candelier, K., A. Atli and J. Alteyrac. 2018. Termite and decay resistance of bioplast-spruce green wood-plastic composites. European Journal of Wood and Wood Products. 77(2019) 157-169. Doi: 10.1007/s00107-018-1368-y. [ Links ]
Cárdenas, A. 2012. Evaluación de la eficiencia como preservante antifúngico del borato de zinc y su efecto en las propiedades físicas y mecánicas de materiales compuestos de plástico y madera de pino. Tesis de Maestría en Ciencia de Productos Forestales. Departamento de Madera, Celulosa y Papel. Universidad de Guadalajara. Guadalajara, Jal., México. 145 p. [ Links ]
Catto, A., L. Montagna, S. Almeida, R. Silveira and R. Santana. 2016. Wood plastic composites weathering: Effects of compatibilization on biodegradation in soil and fungal decay. International Biodeterioration & Biodegradation 109(1): 11-22. Doi:org/10.1016/j.ibiod.2015.12.026. [ Links ]
Córdova, A., A. Cárdenas y H. Gonzáles. 2020. Caracterización física y mecánica de compuestos de Guazuma crinita Mart. a base de polipropileno virgen. Revista Mexicana de Ciencias Forestales Vol. 11 (57). Doi: 10.29298/rmcf.v11i57.621. [ Links ]
Encinas, O. y N. Mora. 2003. Patrones de degradación de las maderas de Pino caribe, Curarire y Drago por Gleophyllum trabeum, Trametes versicolor y Pycnoporus sanguineus. Revista forestal venezolana 1(47):2-14 Revista forestal venezolana 1(47):2-14 http://www.saber.ula.ve/handle/123456789/24336 (12 de marzo de 2020). [ Links ]
Fabiyi, J., A. McDonald, J. Morrell and C. Freitag. 2011. Effects of wood species on durability and chemical changes of fungal decayed wood plastic composites. Composites Part A: Applied Science and Manufacturing 42(5) 501-510. Doi:10.1016/j.compositesa.2011.01.009. [ Links ]
Fuentes, F., J. Silva, F. Quintana, J. Turrado, A. Cárdenas, R. Rodríguez y J. Robledo. 2014. Comportamiento al intemperismo natural de compositos polipropileno madera. Revista Mexicana de Ciencias Forestales . 6 (27): 102-113. Doi: 10.29298/rmcf.v6i27.284. [ Links ]
Ghavami, K. 2005. Bamboo as reinforcement in structural concrete elements. 2005. Cement & Concrete Composites 27:637-49. Doi:10.1016/j.cemconcomp.2004.06.002. [ Links ]
Hosseinihashemi, S., M. Modirzare, V. Safdari and K. Behzad. 2011. Decay resistance, hardness, water absorption, and thickness swelling of a bagasse fiber/plastic composites. BioResources 6 (3): 3289-3299. Doi:10.15376/biores.6.3.3289-3299. [ Links ]
Ibach, R. 2013. Biological properties of wood. In: Rowell, R. M. (Ed.). Handbook of Wood Chemistry and Wood Composites. 2nd edition. CRC Press. Boca Raton, FL, USA. pp. 99-126. [ Links ]
Irbe, I., B. Andersons, J. Chirkova, U. Kallavus, I. Andersone and O. Faix, O. 2006. On the changes of pinewood (Pinus sylvestris L.) Chemical composition and ultrastructure during the attack by brown-rot fungi Postia placenta and Coniophora puteana. International Biodeterioration & Biodegradation 57(2): 99-106. Doi:10.1016/j.ibiod.2005.12.002. [ Links ]
Kalia, S., B. Kaith and I. Kaur. 2009. Pretreatments of natural fibers and their application as reinforcing material in polymer composites-a review. Polymer Engineering and Science 49(7):1253-1272. DOI 10.1002/pen.21328. [ Links ]
Kartal, N., A. Sema, T. Evren, N. Yilgör, T. Yoshimura and K. Tsunoda. 2013. Wood and Bamboo-PP Composites: Fungal and Termite Resistance, Water Absortion, and FT-IR Analyses. BioResources 8(1): 1222-1224. Doi: 10.15376/biores.8.1.1222-1244. [ Links ]
Klyosov, A. 2007. Wood-Plastic Composites. John Wiley & Sons, Inc. Hoboken, NJ, USA. pp. 726. [ Links ]
Lázaro, K., H. Gonzáles, A. Cárdenas, y J. Gago. 2016a. Evaluación de las propiedades físicas de material compuesto elaborado con bambú (Guadua angustifolia) y polipropileno. Revista Mexicana de Ciencias Forestales . 7 (38): 79-94. Doi: 10.29298/rmcf.v7i38.6. [ Links ]
Lázaro, K., H. Gonzáles y A. Cárdenas. 2016b. Propiedades mecánicas del material compuesto elaborado con bambú (Guadua angustifolia) y polipropileno. Revista Mexicana de Ciencias Forestales 7 (38): 95-110. Doi: 10.29298/rmcf.v7i38.7. [ Links ]
Lomelí, M., H. Ochoa, F. Fuentes, S. García, M. Cerpa and J. Silva. 2009. Evaluation of accelerated decay of wood plastic composites by Xylophagus fungi. International Biodet & Biodegradation 63: 1030-1035. Doi:10.1016/j.ibiod.2009.08.002. [ Links ]
Lu, J., Q. Wu and I. Negulescu. 2005. Wood-Fiber/High-Density-Polyethylene Composites: Coupling Agent Performance. Journal of Applied Polymer Science no 96: 93-102. Doi: 10.1002/app.21410. [ Links ]
Mankowski, M. and J. Morrell. 2000. Patterns of fungal attack in composites wood. Wood Fiber Science 32(3): 345-367. [ Links ]
Morris, P. and P. Cooper. 1998. Recycled Plastic/Wood Composite Lumber Attacked by Fungi. Forest Products Journal 48(1): 86-88. https://search.proquest.com/openview/ab064d1873aecd47f96c3dbbb5b4da7d/ 1?pq-origsite=gscholar&cbl=25222 (12 de marzo de 2020). [ Links ]
Pendleton, D., T. Hoffard, B. Woodward and M. Wolcott. 2002. Durability of an extruded HDPE/wood composite. Composites and Manufactured products 52(6): 21-27. [ Links ]
Statistical Analysis System (SAS). 2008. SAS Ver. 9.2. SAS, Inc. Cary, NC, USA. n/p. [ Links ]
Schirp, A. and M. Wolcott. 2005. Influence of fungal decay and moisture absorption on mechanical properties of extruded wood-plastic composites. Wood and Fiber Science 37(4): 643-652. https://wfs.swst.org/index.php/wfs/article/view/1541/1541 (9 de marzo de 2020). [ Links ]
Stark, N. 2001. Influence of Moisture Absorption on Mechanical Properties of Wood Flour-Polypropylene Composites. Journal of Thermoplastic Composite Materials 14(5): 643-652. Doi: 10.1106/UDKY-0403-626E-1H4P. [ Links ]
Steckel, V., C. Clemons and H. Thoemen. 2006. Effects of material parameters on the diffusion and sorption properties of wood-flour/polypropylene composites. Applied Polymer Science 103(2): 752-763. Doi: 10.1002/app.25037. [ Links ]
Teixeira, J., M. Matos, B. Nascimento, S. Griza, F. Rodrigues and R. Marino. 2018. Production and mechanical evaluation of biodegradable composites by white rot fungi. Ciência e Agrotecnologia 42(6):676-684. Doi:10.1590/1413-70542018426022318. [ Links ]
Verhey, S. and P. Laks. 2002. Wood particles size affects the decay resistance of wood fiber/thermoplastic composites. Forest Products Journal 52 (11): 78-81. https://search.proquest.com/openview/fd3974e418bbaeab8c3f814092c4644c/1?pq-origsite=gscholar&cbl=25222 (12 de marzo de 2020). [ Links ]
Wang, W. and J. Morrel. 2004. Water sorption characteristics of two wood-plastic composites. Forest Products Journal 54(12): 209-212. https://ir.library.oregonstate.edu/concern/articles/sf2685560 (14 de marzo de 2020). [ Links ]
Westman, M., L. Fifield, K. Simmons, S. Laddha, T. Kafentzis. 2010. Natural Fiber Composites: A Review. Department of Energy of US. Richland, WA, USA. Doi: 10.2172/989448. [ Links ]
Received: April 16, 2020; Accepted: August 24, 2020