Services on Demand
Journal
Article
Indicators
-
Cited by SciELO
-
Access statistics
Related links
-
Similars in SciELO
Share
Revista mexicana de ciencias forestales
Print version ISSN 2007-1132
Rev. mex. de cienc. forestales vol.11 n.57 México Jan./Feb. 2020 Epub June 20, 2020
https://doi.org/10.29298/rmcf.v11i57.556
Scientific article
Intraspecific variation of functional traits in Cedrela odorata L. in the Nicoya Peninsula, Costa Rica
1Centro Agronómico Tropical de Enseñanza e Investigación. CATIE. Costa Rica.
Climate change will modify the global hydrological regime due to changes in the magnitude of precipitation and temperature and their interaction with the physical and vegetation conditions of each place. This entails a great risk for the conservation of species because it involves variations in the conditions that are crucial to their performance in the ecosystem. In response to abiotic and biotic habitat factors, plants can show great intraspecific trait variability; this ability allows them to survive, grow and reproduce in different scenarios. The study of the intraspecific variability of morphological characters allows us to understand the potential response of species to engines of change, such as climate change. The aim of this study was to evaluate the intraspecific variability of leaf area (mm2), specific leaf area (mm-2 mg), leaf dry matter content (mg-1 g), wood density (g cm-3) and bark thickness (cm) of 90 individuals of Cedrela odorata in three natural forests with different annual rainfall regimes. It was hypothesized that C. odorata can modulate the magnitude of expression of the five morphological characters according to different precipitation conditions. Results showed that functional attribute values varied significantly between populations with different rainfall regimes; furthermore, the species was observed to express its traits within an axis of acquisitive-conservative specialization in the use of resources. The intraspecific variability of traits of C. odorata may be a mechanism of resilience to climate change.
Key words Functional ecology; community assembly; habitat filtering; phenotypic plasticity; functional traits; intraspecific variability
El cambio climático modificará el régimen hidrológico global a causa de alteraciones en la magnitud de la precipitación y temperatura, y su interacción con las condiciones físicas y de vegetación de cada lugar; esto representa un gran riesgo para la conservación de las especies, ya que implica variaciones en las condiciones que les son cruciales para su desarrollo. En respuesta a los factores abióticos y bióticos del hábitat, las plantas muestran gran variabilidad intraespecífica de rasgos, esta habilidad les permite sobrevivir, crecer y reproducirse en diversos escenarios. Estudiar la variabilidad intraespecífica de caracteres morfológicos conlleva a entender el potencial de respuesta de las especies a factores de alteración, como el cambio climático. El objetivo del presente estudio fue evaluar la variabilidad intraespecífica del área foliar (mm2), el área foliar específica (mm-2 mg), contenido de materia seca foliar (mg-1 g), densidad de madera (g cm-3) y grosor de corteza (cm) de 90 individuos de Cedrela odorata en tres bosques naturales con distintos regímenes de precipitación anual. A partir de la hipótesis de que C. odorata podría modular la magnitud de expresión de los caracteres morfológicos antes mencionados, de acuerdo con las diferentes condiciones de precipitación. Los resultados demostraron que los valores de los atributos funcionales variaron significativamente entre las poblaciones estudiadas; además, se evidenció que el taxón expresó sus rasgos en un eje de especialización adquisitivo-conservativo en el uso de los recursos. La variabilidad intraespecífica de rasgos en C. odorata podría ser un mecanismo de resiliencia ante el cambio climático.
Palabras clave Ecología funcional; estructura de comunidades; filtro ambiental; plasticidad fenotípica; rasgo funcional; variabilidad intraespecífica
Introduction
Functional traits are all those morpho-physio-phenological characteristics that exert a direct or indirect influence on the biological success of the plant and which are manifest in its growth, reproduction and survival (Lavorel and Garnier, 2002; Violle et al., 2007; Pérez-Harguindeguy et al., 2016). Within an ecosystem, the climatic conditions, the availability of resources and the natural and anthropic disturbances act as a natural filter for the species; only those with a given set of attributes overcome the environmental barrier and attain biological success by coexisting with other similar functional characteristics (Keddy, 1992; Zobel, 1997; Díaz et al., 1998; Lortie et al., 2004; Cornwell and Ackerly, 2009).
Climate change will modify the global hydrological regime as a consequence of changes in the magnitude of both the precipitation and the temperature and their interaction with the physical and vegetation conditions of each place (IPCC, 2007; Imbach et al., 2010). This entails a great risk for the conservation of the species, as it involves changes in the natural conditions that are crucial to their development within the ecosystem (Sala et al., 2000; Nicotra et al., 2010).
Based on the climatic variations predicted for Costa Rica, dry and humid ecosystems will be the most exposed and the most sensitive (Locatelli and Imbach, 2010); these are the natural habitats of Cedrela odorata L., and therefore the permanence of this species in space and time is at risk.
Cedrela odorata is a tropical tree species of enormous value for the forestry industry; for this reason, its conservation is relevant for Costa Rica and for the world at large (Gillie et al., 1997). Its natural distribution stretches from Mexico to northern Argentina; it inhabits a broad range of ecosystems with various environmental conditions (Pennington and Sarukhán, 2005; Rojas-Rodríguez and Torres-Córdoba, 2013). In Costa Rica, it grows in both seasonally dry and very humid environments, exhibiting different phenotypic characteristics in each (Navarroet al., 2002; Cavers et al., 2003).
The taxa adjust the magnitude of the expression of their functional features according to the conditions and restrictions of the habitat, resulting in suitable phenotypes that are able to attain biological success (Bolnick et al., 2003; Jung et al., 2010; Bolnick et al., 2011). This divergence between individuals of the same species is known as intraspecific variation and is regarded as a microevolutionary process (Masuelli and Marfil, 2011). The intraspecific variability of morphological features/traits may be the source of the resilience of the taxa and, therefore, of the ecosystems in the face of climate modification (Garzón et al., 2011; Jung et al., 2014; Moran et al., 2016).
Studying intraspecific variability makes it possible to understand the response potential of the species to alteration factors, such as climate change (Cianciaruso et al., 2009; Salgado, 2016; Des Roches et al., 2018). However, so far there is no clear method to measure it correctly, given that the protocols for its evaluation have focused mainly on the variation between species, i.e. interspecific variability, while neglecting intraspecific variation (Albert et al., 2010a; Albert et al., 2011).
The objective of the present study was to assess the intraspecific variability of the functional traits exhibited by Cedrela odorata populations in three types of natural forests with different rainfall regimes in the Nicoya Peninsula, in Costa Rica. The selection of the features was based on their acknowledged relationship with vital processes of the plant, such as the acquisition and allotment of resources, adaptation to change factors and survival from disturbances (Cornelissen, 1999; Poorter and Jong, 1999; Poorter and Garnier, 2001; Hacke et al., 2001; Mediavilla et al., 2001; Wright and Cannon, 2001; Westoby et al., 2002; Wright and Westoby, 2002; King et al., 2006; Poorter and Bongers, 2006; Sterkt et al., 2006; Álvarez‐Clare and Kitajama, 2007; Chao et al., 2008; Curran et al., 2008; Wright et al., 2017) (Table 1). C. odorata individuals are expected not to exhibit constant values for these features throughout the environmental gradient, as the manner in which an individual expresses its functional attributes is closely related to the specific environmental conditions of the habitat where it pursues biological success (Poorter, 2008; Albert et al., 2010b).
Table 1 List of measured functional traits, abbreviation and the units in which they are evaluated.
Functional trait | Abbreviation | Unit |
---|---|---|
Foliar | ||
Leaf area | LA | mm2 |
Specific leaf area | SLA | mm-2 mg |
Content of leaf dry matter | CLDM | mg-1 g |
Stem | ||
Wood density | WD | g cm-3 |
Bark thickness | BT | cm |
The results contribute to the knowledge of this microevolutionary process and make it possible to glimpse the response potential expressed by vegetal species in the face of environmental variations, as well as its implications for their conservation.
Materials and Methods
The intraspecific variability of five morphological features was assessed: leaf area (mm2), specific leaf area (mm-2 mg), content of leaf dry matter (mg-1 g), wood density (g cm-3), and bark thickness (cm) of 90 C. odorata individuals in three natural forests under different annual precipitation regimes.
The climate change scenarios suggest that the hydrological regimes will be potentially affected (Arnell, 1999; Arnell 2003); however, the knowledge of how the plants will respond to this situation is scarce. For this reason, the present paper considered only the mean annual precipitation as the environmental filter with an effect on the adjustment process of the values that correspond to the traits cited above.
Study area
The study was carried out in the region of the Nicoya Peninsula in Costa Rica (10°51´5´´ - 9°34´10´´ LN; 85° 42´ 27.3´´ - 85° 06´41.6´´ W). The topography is generally flat, with a single elevation at the center reaching 900 masl. According to the climate data of Worldclim (http://www.worldclim.org/), the area exhibits a north-south precipitation gradient; the conditions of low precipitation and seasonality of the rains occur toward the northern part of the peninsula, on the border with Nicaragua, while toward the south the conditions are more humid (Figure 1).
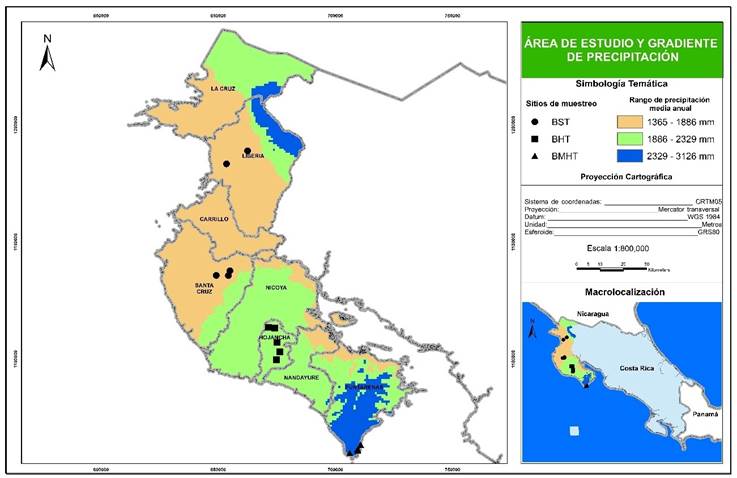
Área de studio = Study area; Gradiente de precipitación = Precipitation gradient; Sitios de muestreo = Sampling sites; Proyección cartográfica = Cartographic projection; Sistema de coordenadas = Coordinates system; Esferoide = Spheroid.
Figure 1 Study area, precipitation gradient, and location of the sampling sites per stratum.
The Arc Gis software, version 10.2, and the raster layer of the bioclimatic variable “annual precipitation” from the online database of Wordclim were utilized to spatially define the precipitation gradient in the peninsula. The precipitation gradient was divided into three strata (Table 2), each one associated to a Holdridge life zone (Holdridge, 1967).
Table 2 Stratification of the precipitation gradient in the Nicoya Peninsula, Costa Rica.
Stratification of the gradient | Mean precipitation interval (mm) | Holdridge life zone |
---|---|---|
Stratum 1 | 1 365-1 886 | Tropical dry forest (TDF) |
Stratum 2 | 1 886-2 329 | Tropical wet forest (TWF) |
Stratum 3 | 2 329-3 126 | Tropical very wet forest (TVWF) |
Sampling and evaluation of morphological features/traits
The functional traits of 30 Cedrela odorata were measured for each stratum of the gradient, using 14 sampling sites (Figure 1) within the patches of secondary forest, according to Finegan’s definition (1996). The sampled sites were relatively flat, with a slope of 0 to 15 %, and the minimum separation between individuals was 50 m. Only C. odorata trees with a healthy appearance and a normal diameter (ND) of 30 to 100 cm were measured.
Assessed variables
The five attributes mentioned above was measured. The LA, SLA, CLDM and BT were measured and estimated according to the standardized protocol of functional traits of the plants (Pérez-Harguindeguy et al. 2013). For the WD, the specific gravity method was used (Williamson and Wieman 2010). The value of this risk per individual corresponded directly to the estimate made with the proposed method. The traits were measured during the sampling period, which coincided with the time when the trees had their full foliage in the year 2017. Only mature leaves of the part exposed to the sunlight and without evidence of herbivory or damage by pathogens were considered.
For the foliar area estimation, a conventional HP Scanjet 300 Flatbed scanner and the Leaf Area Measurement software version 1.3. were used. Also, a H-5851 OHAUS SCOUT analytical balance to weigh the samples, in addition to conventional laboratory equipment such as specimens and Kimax beakers. To measure bark thickness, a Suunto bark caliper was utilized.
The protocol for the record of functional traits indicates that 10 leaves per taxon -or, preferably, 20 leaves from 10 individuals, i.e. two leaves from 10 specimens at random- are required for woody species. However, the procedure is designed for the performance of studies at the interspecific level, which consider only the average value of the traits of the community and disregard the wide variability that may be exhibited by members of the same species.
To the present day, the choice of the best sampling strategy for capturing mean values of morphological traits of a species or population while preserving the information on the variability of the traits and minimizing the sampling size and effort is a topic open for discussion in the ecology of functional traits (Pretuzzelis et al., 2017).
Therefore, in order to include the greatest possible intraspecific variability of morphological features per specimen, in the present study, 20 leaves per tree were collected and measured, without taking into account the petiole of the leaflets, which was withdrawn before processing the samples. Thus, the individual value of the leaf characteristics (LA, SLA, CLDM) was the mean of the values of the 20 leaves considered per tree.
Data analysis
A linear model of mixed effects was executed in order to know the effect of the precipitation gradient on the variation of the values of the functional traits. The attributes were regarded as the response variable of the model; the sampling site, as a random factor, and the life zone of the gradient as the fixed factor. Subsequently, a multiple comparison test was performed using the LSD method (Fisher) with a level of significance of 0.05 (Williams y Abdi, 2010), in order to know what populations differ statistically from one another. Furthermore, a principal component analysis (PCA) of the five assessed traits was carried out in order to obtain the grouping of the 90 assessed specimens according to the magnitude of expression of its morphological traits. All the analyses were performed using the InfoStat statistical platform, version 2008 (Di Rienzo et al., 2018).
Results and Discussion
Intraspecific variation of traits between strata
The variability of a set of traits in a given population may be due to local adaptation or to phenotypical plasticity; i.e. the range of phenotypes that the same genotype expresses in response to environmental heterogeneity, in space and time (epigenetics, microevolution) (Geber and Griffen, 2003; Richards et al., 2010;Violle et al., 2012).
The plants exhibit a great intraspecific variability of traits in response to the abiotic or biotic restriction of the ecosystem (Violle et al., 2007; Violle et al., 2012). This ability allows them to survive, grow and reproduce under new environmental conditions (Joshi et al., 2001). Many vegetal populations have been proven, even in relatively small areas, to exhibit a great phenotypical variation in a set of features that include characteristics of life forms, resistance to pathogens and herbivory, as well as the differentiation of strategies of acquisition and distribution of nutrients (Wellstein et al., 2013).
In this study, C. odorata exhibited intraspecific variation in all the assessed functional traits. The interval of variation of the morphological traits was the following: WD: 0.27-0.48 g cm-3, BT: 0.7-4.34 cm, CLDM: 115-372 mg-1 g, LA: 22 048-68 566 mm2, and SLA: 15.4-53.6 mm-2 mg. The bark thickness and the specific leaf area were the functional attributes with the largest variation along the gradient of precipitation (Table 3). The analysis of the linear model of mixed effects and their mean comparison test proved the existence of a significant effect of the stratum on the variation in the values of the functional features of each assessed individual (Table 4).
Table 3 Descriptive statistics of the functional attributes assessed in Cedrela odorata L. in the Nicoya Peninsula, Costa Rica.
Trait | Unit | n | Mean | S.E | C.V | Min. | Max. |
---|---|---|---|---|---|---|---|
LA | mm2 | 90 | 40 287 | 872 | 20.53 | 22 048 | 685 666 |
SLA | mm-2 mg | 90 | 26.2 | 1 | 36.25 | 15.4 | 53.6 |
CLDM | mg-1 g | 90 | 242.2 | 6.04 | 23.65 | 115 | 372.2 |
WD | g cm-3 | 90 | 0.4 | 0 | 11.748 | 0.27 | 0.48 |
BT | cm | 90 | 2.2 | 0.08 | 34.37 | 0.74 | 4.34 |
LAF = Leaf area; SLA = Specific leaf area; CLDM = Content of leaf dry matter; WD = Wood density; BT = Bark thickness; S.E = Standard error; CV = Coefficient of variation.
Table 4 List of analyzed functional traits, mean values, and effect of the gradient for each stratum.
Trait | Unit | TDF | TWF | TVWF | F | P |
---|---|---|---|---|---|---|
LA | mm2 | 35 869±1 712.5 b | 41 936±1 681.1 a | 43 059±17 36.7 a | 4.95 | 0.029* |
SLA | mm-2 mg | 20.1 ± 2.8 b | 35 ± 2.82 a | 23 ± 3.13 b | 7.59 | 0.009** |
CLDM | mg-1 g | 274 ±16.87 a | 190 ±16.8 b | 269 ± 18.6 a | 7.63 | 0.008** |
WD | g cm-3 | 0.41 ±0.1 a | 0.4 ± 0.1 b | 0.41 ±0.1 a | 4 | 0.05* |
BT | cm | 2.7 ± 0.14 a | 1.7 ± 0.14 b | 2.3 ± 0.15 a | 13.7 | 0.001** |
LA = Leaf area; SLA = Specific leaf area; CLDM = Content of leaf dry matter; WD = Wood density; BT = Bark thickness; TDF = Tropical dry forest; TWF = Tropical wet forest; TVWF = Tropical very wet forest; Mean values with different letters represent statistically significant differences. The level of significance is expressed as: *P<0.05, **P <0.01.
The TDF and the TWF exhibited significant differences in all the assessed functional traits (p≤ 0.05); i.e. the populations of both ecosystems are significantly different, since each expressed the five considered traits to a different extent. The population of the TDF had a thinner foliar lamina, a smaller specific leaf area, a larger content of dry matter per leaflet and of specific wood density and a greater bark thickness, in relation to the TWF (Figure 2).
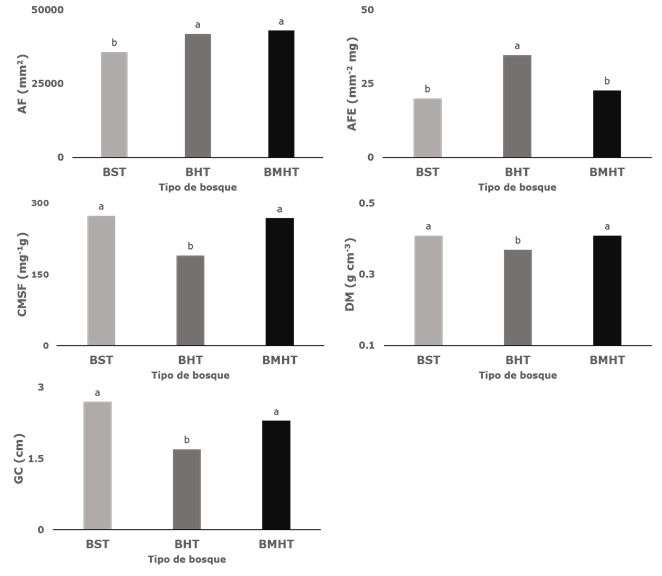
Different letters represent statistically significant differences (p ≤ 0.05).
Figure 2 Intraspecific variability of functional traits of Cedrela odorata L. between forest types.
The TDF and the TVWF registered no significant differences (p≤ 0.05) with regard to the WD, BT, CLDM, and SLA, but the LA did. For this trait, the TWF and the TVWF were significantly different from the TDF, which exhibited smallest the foliar lamina of all three forests (Figure 2).
The variation of the LA was gradual, according to the conditions of humidity of the precipitation gradient (Figure 2). The mean value was higher in the TWF and the TVWF, with 41 936 ± 1 681.1 mm2 and 43 059 ± 1 736.7 mm2, respectively; these ecosystems exhibited the highest precipitation within the study area, while in the TDF the LA was 35 869 mm2, with a significant difference (p≤0.05).
The reduction in the size of the foliar lamina is generally interpreted as an adaptation to drought and to the high degree of radiation (Givnish, 1987; Cornelissen et al., 2003). This trait has been observed to have a positive relationship with the precipitation (Hamann, 1979; Mencuccini and Grace, 1995; Yates et al., 2010; Wright et al., 2017). Xu et al. (2009) argue that the variation in the size of the leaf sheet along a climate gradient may be due to the various demands of evapotranspiration.
It is barely appropriate to interpret the variation of each trait separately, as the organs of the plants perform more than one function, as in the case of the bark thickness, which not only has protective functions against biotic and abiotic factors, but is also related to the allotment and distribution of the nutrients and the water within the plant, and to the respiration and photosynthesis at the level of the stem (Roth, 1981; Pfanz et al., 2002; Paine et al., 2010, Cernusak and Hutley, 2011; Lawes et al., 2011; Poorter et al., 2014; Pausas, 2015; Rosell et al., 2015; Rosell, 2016). The compensation may be in the entire plant, not just in a particular organ. The adjustment of the values of the functional traits may be the result of the coordinated compensation within each individual (Rosell, 2014).
Functional strategies in the use of the resources
In response to the heterogeneous availability of resources in the environment and for purposes of their efficient exploitation, functional ecology suggests that the vegetal species may migrate within a range of functional variation along specific specialization axes (Wright et al., 2004; Freschet et al., 2010; Freschet et al., 2012; Reich, 2014), and alternate between conservative strategies in environments where the natural conditions accentuate the environmental barrier (conservative species) and acquisitive strategies, in which a rapid acquisition and renewal of resources (acquisitive species) is pursued. This compensation is based on the investment of resources in the various organs and tissues of the plant to enhance its durability and degree of tolerance to different types of environmental conditions.
The conservative species are characterized by investing in more dense, lasting and protective tissues, while acquisitive species choose soft, rapid-growing tissues (Díaz et al., 2004; Chave et al., 2009; Baraloto et al., 2010; de la Riva et al., 2014; Díaz et al., 2016).
The principal component analysis (Figure 3) shows a main axis that explains the largest part of the variability (51.7 %), in which the functional traits and the individuals are distributed along this first specialization axis of acquisitive and conservative strategies in regard to the use of resources. Thus, the individuals associated with conservative strategies (high contents of leaf dry matter, higher wood density and greater bark thickness) were located at the negative extreme, while the individuals associated with more acquisitive strategies (with high values for specific areas of their leaves) were placed at the opposite end.
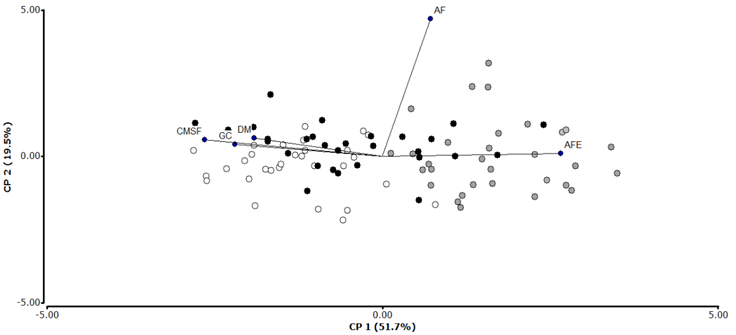
White circles = TDF individuals; Gray circles = TWF individuals; Black circles = TVWF individuals (see abbreviations in Tables 1 and 2).
Figure 3 Principal component analysis for the 5 functional traits and the 90 Cedrela odorata L. individuals considered in this study.
The trees of the TDF and 66 % of the population of the TVWF have been observed to grow in the conservative region of the axis. Based on the values of the SLA, CLDM, WD and BT, we infer that this population of C. odorata has a low photosynthesis rate and a low relative growth, as it invests its resources in leaf tissues and has a denser, stronger stem, which affords it greater resistance to pathogenic agents, to herbivority, and to physical damage due to abiotic factors; therefore, these individuals have a higher life expectancy. All specimens of the THG and the rest of the population of the TVWF are at the opposite extreme. According to the values of the traits of this population, these trees have a higher photosynthesis rate than the conservative population, and therefore, they have a greater relative growth. However, the tissues of their leaves and stem are less resistant to biotic and abiotic factors, so they have a lower life expectancy.
The LA had no relationship with these traits because its variance is associated to axis two (19.5 %) and is independent from other features; the variation of this morphological feature may be associated to a different specialization axis.
The functional attributes of the populations of the extremes of the gradient (TDF and TVWF) showed similar values, which suggests that precipitation is not the filter with the greatest effect on the process of adjustment of these attributes. There may be another factor or a synergy of various factors that influence the intraspecific variation in C. odorata in the study area (Bergholz et al., 2017). Lohbeck et al. (2013) suggest that, when the effect of the availability of water diminishes, other environmental elements, like the availability of light due to inter- and intraspecific competition, acquire greater strength and therefore the individuals focus their functional strategies on tolerating the low availability of light due to the presence of a tighter canopy.
Based on various climate change scenarios for the study area, Fung et al. (2017) estimate that, by the year 2060, the dry forest of the Nicoya Peninsula will displace the wet forest. Apparently, this change will be rapid, as the flat terrain that is characteristic of this area will favor the process. In this sense, the results of this research suggest that the populations of C. odorata existing in the Nicoya Peninsula have the potential to modulate the expression of their functional traits and adapt to the new environmental conditions that climate change will bring about in this area of the planet. However, the rapidity with which the species may adjust its traits and, therefore, whether these adjustments will allow the survival of the species, is unknown.
The results indicate that the plants have a great variability between phenotypes and highlight the importance of considering the intraspecific variability of traits when seeking to understand the adaptive responses of the species to environmental alterations. The study of the variation of the functional traits is necessary to know how the living organisms may respond to global changes (McGill et al. 2006). These responses will depend on the type of character and its inheritability (Lloyd-Smith et al., 2005).
Conclusions
The study of the variation of functional risks of Cedrela odorata proves that there is a degree of divergence between phenotypes along a precipitation gradient in the Nicoya Peninsula, in Costa Rica. Furthermore, the leaf area was observed to be larger in individuals belonging to humid ecosystems.
In his geographical area, Cedrela odorata has been shown to exhibit its morphological traits within an acquisitive-conservative specialization axis of resource use. In this regard, the results suggest that this species has the potential to adapt to the new environmental conditions that are predicted for the Nicoya area; however, climate change is a complex scenario where the response of the species can be strongly conditioned by the geography of the study area, the time-space scale considered, the nature and width of the sampled environmental gradient, or even the genotype of the individuals. Therefore, further research on the intraspecific variability of the morphological traits is required in order to determine how climate change will affect the performance and distribution of the taxa.
Acknowledgements
The authors are grateful to the chair of Forests and Climate Change of the Tropical Agronomic Center for Research and Teaching (CATIE), the Cantonal Agricultural Center of Hojancha (CACH), and the regional Office of Hojancha of the Ministry of the Environment and Energy of Costa Rica (Minae) for their logistical and material support; to Higinio Juárez and Angélica Osorio, for their important collaboration in the field stage; to the Engineer Óscar Cantú, for drawing the maps, and, finally, to the National Council for Science and Technology (Consejo Nacional de Ciencia y Tecnología, Conacyt), for the economic support it provided through an excellence scholarship.
REFERENCES
Albert C. H., W. Thuiller, N. G. Yoccoz, R. Douzet, S. Aubert and S. Lavorel. 2010a. A multi‐trait approach reveals the structure and the relative importance of intra‐vs. interspecific variability in plant traits. Functional Ecology 24(6): 1192-1201. Doi:10.1111/j.1365-2435.2010.01727.x. [ Links ]
Albert, C. H., W. Thuiller, N. G. Yoccoz, A. Soudant, F. Boucher, P. Saccone and S. Lavorel. 2010b. Intraspecific functional variability: extent, structure and sources of variation. Journal of Ecology 98(3): 604-613. Doi: 10.1111/j.1365-2745.2010.01651.x. [ Links ]
Albert, C. H. , F. Grassein, F. M. Schurr, G. Vieilledent and C. Violle. 2011. When and how should intraspecific variability be considered in trait-based plant ecology? Perspectives in Plant Ecology, Evolution and Systematics 13(3): 217-225. Doi:10.1016/j.ppees.2011.04.003. [ Links ]
Álvarez‐Clare, S. and K. Kitajima. 2007. Physical defense traits enhance seedling survival of neotropical tree species. Functional Ecology 216: 1044-1054. Doi:10.1111/j.1365-2435.2007.01320.x. [ Links ]
Arnell, N. W. 1999. Climate change and global water resources. Global environmental change 9: S31-S49. Doi: 10.1016/s0959-3780(99)00017-5. [ Links ]
Arnell, N. W. 2003. Effects of IPCC SRES* emissions scenarios on river runoff: a global perspective. Hydrology and Earth System Sciences Discussions 7(5): 619-641. Doi:10.5194/hess-7-619-2003. [ Links ]
Baraloto, C., C. E. T. Paine, L. Poorter, J. Beauchene, D. Bonal, A.-M. Domenach, B. Hérault, S. Patiño, J.-C. Roggy and J. C. Chave. 2010. Decoupled leaf and stem economics in rain forest trees. Ecology Letters 13(11):1338-1347. Doi:10.1111/j.1461-0248.2010.01517.x. [ Links ]
Bergholz, K., F. May, M. Ristow, I. Giladi, Y. Ziv and F. Jeltsch. 2017. Two Mediterranean annuals feature high within-population trait variability and respond differently to a precipitation gradient. Basic and Applied Ecology 25: 48-58. Doi:10.1016/j.baae.2017.11.001. [ Links ]
Bolnick, D. I., R. Svanbäck, J. A. Fordyce, L. H. Yang, J. M. Davis, C. D. Hulsey and M. L. Forister. 2003. The ecology of individuals: incidence and implications of individual specialization. The American Naturalist 161(1): 1-28. Doi:10.1086/343878. [ Links ]
Bolnick, D. I. , P. Amarasekare, M. S. Araújo, R. Bürger, J. M. Levine, M. Novak, V. H. W. Rudolf, S. J. Schreiber, M. C. Urban and D. A. Vasseur. 2011. Why intraspecific trait variation matters in community ecology. Trends in Ecology & Evolution 26(4): 183-192. Doi:10.1016/j.tree.2011.01.009. [ Links ]
Cavers, S., C. Navarro and A. Lowe. 2003. A combination of molecular markers identifies evolutionarily significant units in Cedrela odorata L. (Meliaceae) in Costa Rica. Conservation Genetics 45: 571-580. Doi: 10.1023/A:1025692321860. [ Links ]
Cernusak, L. A. and L. B. Hutley. 2011. Stable isotopes reveal the contribution of corticular photosynthesis to growth in branches of Eucalyptus miniata. Plant physiology 155(1): 515-523. Doi: 10.1104/pp.110.163337. [ Links ]
Chao, K.-J., O. L. Phillips, E. Gloor, A. Monteagudo, A. Torres‐Lezama y R. Vásquez M. 2008. Growth and wood density predict tree mortality in Amazon forests. Journal of Ecology 96(2): 281-292. Doi:10.1111/j.1365-2745.2007.01343.x. [ Links ]
Chave, J., D. Coomes, S. Jansen, S. L. Lewis, N. G. Swenson and A. E. Zanne. 2009. Towards a worldwide wood economics spectrum. Ecology Letters 12(4): 351-366. Doi: 10.1111/j.1461-0248.2009.01285.x. [ Links ]
Cianciaruso, M. V., M. A. Batalha, K. J. Gaston and O. L. Petchey. 2009. Including intraspecific variability in functional diversity.Ecology 90(1): 81-89. Doi: 10.1890/07-1864.1. [ Links ]
Cornelissen J., H. C. 1999. A triangular relationship between leaf size and seed size among woody species: allometry, ontogeny, ecology and taxonomy. Oecologia 118(2): 248-255. Doi: 10.1007/s004420050725. [ Links ]
Cornelissen, J., S. Lavorel, E. Garnier, S. Díaz, N. Buchmann, D. Gurvich, P. Reich, H. Ter Steege, H. Morgan and M. Van Der Heijden. 2003. A handbook of protocols for standardised and easy measurement of plant functional traits worldwide. Australian Journal of Botany 514: 335-380. Doi: 10.1071/BT02124. [ Links ]
Cornwell, W. K. and D. D. Ackerly. 2009. Community assembly and shifts in plant trait distributions across an environmental gradient in coastal California. Ecological Monographs 791: 109-126. Doi: 10.1890/07-1134.1. [ Links ]
Curran, T. J., L. N. Gersbach, W. Edwards and A. K. Krockenberger. 2008. Wood density predicts plant damage and vegetative recovery rates caused by cyclone disturbance in tropical rainforest tree species of North Queensland, Australia. Austral Ecology 33(4): 442-450. Doi: 10.1111/j.1442-9993.2008.01899.x. [ Links ]
de la Riva E. G., I. M.Pérez-Ramos, C. M. Navarro-Fernández, M. Olmo T. Marañón y R. Villar. 2014. Rasgos funcionales en el género Quercus: estrategias adquisitivas frente a conservativas en el uso de recursos. Revista Ecosistemas 23(2): 82-89. Doi:10.7818/ECOS.2014.23-2.11. [ Links ]
Des Roches, S., D. M. Post, N. E. Turley, J. K. Bailey, A. P. Hendry, M. T. Kinnison, J. A. Schweitzer and E. P. Palkovacs. 2018. The ecological importance of intraspecific variation. Nature Ecology & Evolution 2(1): 57-64. Doi: 10.1038/s41559-017-0402-5. [ Links ]
Di Rienzo, J. A., F. Casanoves, M. G. Balzarini, L. Gonzalez, M. Tablada y C. W. Robledo. 2018. InfoStat versión 2018. Centro de Transferencia InfoStat, FCA, Universidad Nacional de Córdoba, Argentina. URL URL http://www.infostat.com.ar (17 de octubre de 2017). [ Links ]
Díaz, S., M. Cabido and F. Casanoves. 1998. Plant functional traits and environmental filters at a regional scale. Journal of Vegetation Science 91:113-122. Doi:10.2307/3237229. [ Links ]
Díaz, S. , J. G. Hodgson, K. Thompson, M. Cabido, J. H. C. Cornelissen, A. Jalili, G. Montserrat-Martí, J. P. Grime, F. Zarrinkamar, Y. Asri, S. R. Band, S. Basconcelo, P. Castro-Díez, G. Funes, B. Hamzehee, M. Khoshnevi, N. Pérez-Harguindeguy, M. C. Pérez-Rontomé, F. A. Shirvany, F. Vendramini, S. Yazdani, R. Abbas-Azimi, A. Bogaard, S. Boustani, M. Charles, M. Dehghanm, L. de Torres-Espuny, V. Falczuk, J. Guerrero-Campo, A. Hynd, A., G. Jones, E. Kowsary, F. Kazemi-Saeed, M. Maestro-Martínez, A. Romo-Díez, S. Shaw, B. Siavash, P. Villar-Salvador and M. R. Zak. 2004. The plant traits that drive ecosystems: evidence from three continents. Journal of Vegetation Science 15(3):295-304. Doi: 10.1111/j.1654-1103.2004.tb02266.x. [ Links ]
Díaz, S. , J. Kattge, J. H. C. Cornelissen, I. J. Wright, S. Lavorel, S. Dray, b. Reu, M. Kleyer, C. Wirth, I. Colin P., E. Garnier, G. Bönisch, M. Westoby, H. Poorter, P. B. Reich, A. T. Moles, J. Dickie, A. N. Gillison, A. E. Zanne, J. Chave, S. J. Wright, S. N. Sheremet'ev, H. Jactel, C. Baraloto, B. Cerabolini, S. Pierce, B. Shipley, D. Kirkup, F. Casanoves, J. S. Joswig, A. Günther, V. Falczuk, N. Rüger, M. D. Mahecha and L. D. Gorné. 2016. The global spectrum of plant form and function. Nature 529(7585): 167-171. Doi: 10.1038/nature16489. [ Links ]
Finegan, B. 1996. Pattern and process in neotropical secondary rain forests: the first 100 years of succession. Trends in ecology & evolution 11(3): 119-12. Doi:10.1016/0169-5347(96)81090-1. [ Links ]
Freschet, G. T., J. H. Cornelissen, R. S. Van Logtestijn and R. Aerts. 2010. Evidence of the ‘plant economics spectrum’ in a subarctic flora. Journal of Ecology 98(2): 362-373. Doi: 10.1111/j.1365-2745.2009.01615.x. [ Links ]
Freschet, G. T. , R. Aerts and J. H. C. Cornelissen. 2012. A plant economics spectrum of litter decomposability. Functional Ecology 26(1): 56-65. Doi: 10.1111/j.1365-2435.2011.01913.x. [ Links ]
Fung, E., P. Imbach, L. Corrales, S. Vilchez, N. Zamora, F. Argotty, L. Hannah and Z. Ramos. 2017. Mapping conservation priorities and connectivity pathways under climate change for tropical ecosystems. Climatic Change 141(1):77-92. Doi:10.1007/s10584-016-1789-8. [ Links ]
Garzón, M. B., R. Alía, T. M. Robson y M. A. Zavala. 2011. Intra‐specific variability and plasticity influence potential tree species distributions under climate change. Global Ecology and Biogeography 20(5):766-7. Doi: 10.1111/j.1466-8238.2010.00646.x. [ Links ]
Geber, M. A. and L. R. Griffen. 2003. Inheritance and natural selection on functional traits. International Journal of Plant Sciences 164(S3): 21-42. Doi: 10.1086/368233. [ Links ]
Gillies, A. C. M., J. P. Cornelius, A. C. Newton, C. Navarro, M. Hernández and J. Wilson. 1997. Genetic variation in Costa Rican populations of the tropical timber species Cedrela odorata L., assessed using RAPDs. Molecular Ecology 6(12): 1133-1145. Doi:10.1046/j.1365-294X.1997.00287.x. [ Links ]
Givnish, T. J. 1987. Comparative studies of leaf form: assessing the relative roles of selective pressures and phylogenetic constraints. New phytologist 106: 131-160. Doi:10.1111/j.1469-8137.1987.tb04687.x. [ Links ]
Hacke, U. G., J. S. Sperry, W. T. Pockman, S. D. Davis and K. A. McCulloh. 2001. Trends in wood density and structure are linked to prevention of xylem implosion by negative pressure. Oecologia 126(4):457-461. Doi: 10.1007/s00442010. [ Links ]
Hamann, O. 1979. On Climatic Conditions, Vegetation Types, and Leaf Size in the Galápagos Islands. Biotropica 11(2):101-122. Doi:10.2307/2387785. [ Links ]
Holdridge, L. R. 1967. Life zone ecology. Life zone ecology. Tropical Science Center. The University of Texas. Austin, TX, USA. 207 p. [ Links ]
Imbach, P., L. Molina, B. Locatelli y L. Corrales. 2010. Vulnerabilidad de los servicios ecosistémicos hidrológicos al cambio climático en Mesoamérica. Adaptación al cambio climático y servicios ecosistémicos en América Latina. CATIE. Turrialba, Costa Rica. pp. 32-43. [ Links ]
Intergovernmental Panel on Climate Change (IPCC). 2007. Climate Change 2007: Impacts, Adaptation and Vulnerability. In: Parry, M. L., O. F. Canziani, J. P. Palutikof, P. J. van der Linden and C. E. Hanson (eds.). Contribution of Working Group II to the Fourth Assessment Report of the Intergovernmental Panel on Climate Change. Cambridge University Press. Cambridge, UK. 976 p. [ Links ]
Joshi, J., B. Schmid, M.C. Caldeira, P. G. Dimitrakopoulos, J. Good, R. Harris, A. Hector, K. Huss‐Danell, A. Jumpponen, A. Minns, C. P. H. Mulder, J. S. Pereira, A. Prinz, M. Scherer-Lorenzen, A.-S.D. Siamantziouras, A.C. Terry, A. Y. Troumbis and J. H. Lawton. 2001. Local adaptation enhances performance of common plant species. Ecology Letters 46:536-544. Doi: 10.1046/j.1461-0248.2001.00262.x. [ Links ]
Jung, V., C. Violle, C. Mondy, L. Hoffmann and S. Muller .2010. Intraspecific variability and trait‐based community assembly. Journal of Ecology 98(5):1134-1140. Doi:10.1111/j.1365-2745.2010.001687.x. [ Links ]
Jung, V., C. H. Albert, C. Violle, G. Kunstler, G. Loucougaray and T. Spiegelberger. 2014. Intraspecific trait variability mediates the response of subalpine grassland communities to extreme drought events. Journal of Ecology 102(1):45-53. Doi:10.1111/1365-2745.12177. [ Links ]
Keddy, P. A. 1992. Assembly and response rules: two goals for predictive community ecology. Journal of Vegetation Science 3(2):157-164. Doi: 10.2307/3235676. [ Links ]
King, D. A., S. T. Davies, S. Tan and M. D. Noor. 2006. The role of wood density and stem support costs in the growth and mortality of tropical trees. Journal of Ecology 94:670-680. Doi: 10.1111/j.1365-2745.2006.01112.x. [ Links ]
Lavorel, S. and E. Garnier. 2002. Predicting changes in community composition and ecosystem functioning from plant traits: revisiting the Holy Grail. Functional Ecology 16(5):545-556. Doi: 10.1046/j.1365-2435.2002.00664.x. [ Links ]
Lawes, M. J., A. Richards, J. Dathe and J. J. Midgley. 2011. Bark thickness determines fire resistance of selected tree species from fire-prone tropical savanna in north Australia. Plant Ecology 212(12):2057-2069. Doi: 10.1007/s11258-011-9954-7. [ Links ]
Lloyd-Smith, J. O., S. J. Schreiber, P. E. Kopp and W. M. Getz. 2005. Superspreading and the effect of individual variation on disease emergence. Nature 438:355-359. Doi: 10.1038/nature04153. [ Links ]
Locatelli, B. y P. Imbach. 2010. Migración de ecosistemas bajo escenarios de cambio climático: el rol de los corredores biológicos en Costa Rica. Adaptación al cambio climático y servicios ecosistémicos en América Latina. CATIE. Turrialba, Costa Rica. pp. 44-53. [ Links ]
Lohbeck, M., L. Poorter, E. Lebrija-Trejos, M. Martínez-Ramos, J. A. Meave, H. Paz, E. A. Pérez-García, I. E. Romero-Pérez, A. Tauro and F. Bongers. 2013. Successional changes in functional composition contrast for dry and wet tropical forest. Ecology 94(6): 1211-1216. Doi: 10.1890/12-1850.1. [ Links ]
Lortie, C. J., R. W. Brooker, P. Choler, Z. Kikvidze, R. Michalet, F. I. Pugnaire and R. M. Callaway. 2004. Rethinking plant community theory. Oikos 107(2): 433-438. Doi:10.1111/j.0030-1299.2004.13250.x. [ Links ]
Masuelli, R. W. y C. F. Marfil. 2011. Variabilidad epigenética en plantas y evolución. BAG. Journal of Basic and Applied Genetics 22(1):1-8. [ Links ]
McGill, B. J., B. J. Enquist, E. Weiher and M. Westoby. 2006. Rebuilding community ecology from functional traits. in Trends Ecology& Evolution 21(4):178-185. Doi:10.1016/j.tree.2006.02.002. [ Links ]
Mediavilla, S., A. Escudero and H. Heilmeier. 2001. Internal leaf anatomy and photosynthetic resource-use efficiency: interspecific and intraspecific comparisons. Tree Physiology 21(4):251-259. Doi: 10.1093/treephys/21.4.251. [ Links ]
Mencuccini, M. and J. Grace. 1995. Climate influences the leaf area/sapwood area ratio in Scots pine. Tree Physiology 15(1):1-10. Doi: 10.1093/treephys/15.1.1. [ Links ]
Moran, E. V., F. Hartig and D. M. Bell. 2016. Intraspecific trait variation across scales: implications for understanding global change responses. Global Change Biology 22(1): 137-150. Doi: 10.1111/gcb.13000. [ Links ]
Navarro, C., S. Ward and M. Hernández. 2002. The tree Cedrela odorata (Meliaceae): a morphologically subdivided species in Costa Rica. Revista de Biología Tropical 50(1): 21-29. [ Links ]
Nicotra, A. B., O. K. Atkin, S. P. Bonser, A. M. Davidson, E. J. Finnegan, U. Mathesius and M. van Kleunen. 2010. Plant phenotypic plasticity in a changing climate. Trends in plant science 15(12): 684-692. Doi: 10.1016/j.tplants.2010.09.008. [ Links ]
Paine, C. T., C. Baraloto, J. Chave and B. Hérault. 2011. Functional traits of individual trees reveal ecological constraints on community assembly in tropical rain forests. Oikos 120(5): 720-727. Doi: 10.1111/j.1600-0706.2010.19110.x. [ Links ]
Pausas, J. G. 2015. Bark thickness and fire regime. Functional Ecology 29(3):315-327. Doi: 10.1111/1365-2435.12372. [ Links ]
Pennington, T. D. y J. Sarukhán. 2005. Árboles tropicales de México. Manual para la identificación de las principales especies. Universidad Nacional Autónoma de México. 3a ed. México, D.F., México. 523 p. [ Links ]
Pérez-Harguindeguy, N., S. Díaz, E. Garnier, S. Lavorel, H. Poorter, P. Jaureguiberry, M. S. Bret-Harte, W. K. Cornwell, J. M. Craine, D. E. Gurvich, C. Urcelay, E. J. Veneklaas, P. B. Reich, L. Poorter, I. J. Wright, P. Ray, L. Enrico, J. G. Pausas, A. C. de Vos, N. Buchmann, G. Funes, F. Quétier, J. G. Hodgson, K. Thompson, H. D. Morgan, H. ter Steege, L. Sack, B. Blonder, P. Poschlod, M. V. Vaieretti, G. Conti, A. C. Staver, S. Aquino and J. H. C. Cornelissen. 2013. New handbook for standardised measurement of plant functional traits worldwide. Australian Journal of Botany 61(3):167-234. Doi: 10.1071/BT1225_CO. [ Links ]
Pérez-Harguindeguy, N. , S. Díaz, E. Garnier, S. Lavorel, H. Poorter, P. Jaureguiberry, M. S. Bret-Harte, W. K. Cornwell, J. M. Craine, D. E. Gurvich, C. Urcelay, E. J. Veneklaas, P. B. Reich, L. Poorter, I. J. Wright, P. Ray, L. Enrico, J. G. Pausas, A. C. de Vos, N. Buchmann, G. Funes, F. Quétier, J. G. Hodgson, K. Thompson, H. D. Morgan, H. ter Steege, L. Sack, B. Blonder, P. Poschlod, M. V. Vaieretti, G. Conti, A. C. Staver, S. Aquino and J. H. C. Cornelissen. 2016. Corrigendum to: new handbook for standardised measurement of plant functional traits worldwide. Australian Journal of Botany 64(8): 715-716. Doi: 10.1071/BT12225_CO. [ Links ]
Petruzzellis, F., C. Palandrani, T. Savi, R. Alberti, A. Nardini and G. Bacaro. 2017. Sampling intraspecific variability in leaf functional traits: Practical suggestions to maximize collected information. Ecology and evolution 7(24): 11236-11245. Doi:10.1002/ece3.3617. [ Links ]
Pfanz, H., G. Aschan, R. Langenfeld-Heyser, C. Wittmann and M. Loose. 2002. Ecology and ecophysiology of tree stems: corticular and wood photosynthesis. Naturwissenschaften 89(4): 147-162. Doi: 10.1007/s00114-002-0309-z. [ Links ]
Poorter, H. and R. de Jong. 1999. A comparison of specific leaf area, chemical composition and leaf construction costs of field plants from 15 habitats differing in productivity. The New Phytologist 143(1):163-176. Doi: 10.1046/j.1469-8137.1999.00428.x. [ Links ]
Poorter, H. and E. Garnier. 1999. Ecological significance of inherent variation in relative growth rate and its components. Handbook of functional plant ecology 20: 81-120. Doi: 10.1201/9781420007626.ch3. [ Links ]
Poorter, L. and F. Bongers. 2006. Leaf traits are good predictors of plant performance across 53 rain forest species. Ecology 87(7):1733-1743. Doi: 10.1890/0012-9658(2006)87[1733:LTAGPO]2.0.CO;2. [ Links ]
Poorter, L., S. J. Wright, H. Paz, D. D. Ackerly, R. Condit, G. Ibarra-Manríquez, K. E. Harms, J. Licona, M. Martinez-Ramos and S. Mazer. 2008. Are functional traits good predictors of demographic rates? Evidence from five neotropical forests. Ecology 897:1908-1920. Doi: 101890/07-0207.1. [ Links ]
Poorter, L. , A. McNeil, V.-H. Hurtado, H. H. Prins and F. E. Putz. 2014. Bark traits and life‐history strategies of tropical dry‐and moist forest trees. Functional Ecology 28(1):232-242. Doi: 10.1111/1365-2435.12158. [ Links ]
Reich, P. B. 2014. The world‐wide ‘fast-slow’plant economics spectrum: a traits manifesto. Journal of Ecology 102(2): 275-301. Doi: 10.1111/1365-2745.12211. [ Links ]
Richards, C. L., O. Bossdorf and M. Pigliucci. 2010. What role does heritable epigenetic variation play in phenotypic evolution? BioScience 60(3):232-237. Doi: 10.1525/bio.2010.60.3.9. [ Links ]
Rojas-Rodríguez, F. y G. Torres-Córdoba. 2013. Árboles del Valle Central de Costa Rica: reproducción Cedro amargo (Cedrela odorata L.). Revista Forestal Mesoamericana Kurú 11(26):25-27. Doi: 10.18845/rdmk.v11i26.1593. [ Links ]
Rosell, J. A., S. Gleason, R. Méndez‐Alonzo, Y. Chang and M. Westoby. 2014. Bark functional ecology: evidence for tradeoffs, functional coordination, and environment producing bark diversity. New Phytologist 201(2):486-497. Doi: 10.1111/nph.12541. [ Links ]
Rosell, J. A. , M. Castorena, C. A. Laws and M. Westoby. 2015. Bark ecology of twigs vs. main stems: functional traits across eighty-five species of angiosperms. Oecologia 178(4): 1033-1043. Doi: 10.1007/s00442-015-3307-5. [ Links ]
Rosell, J. A. 2016. Bark thickness across the angiosperms: more than just fire. New Phytologist 2111:90-102. Doi: 10.1111/nph.13889. [ Links ]
Roth, I. 1981. Structural patterns of tropical barks. Encyclopedia of Plant Anatomy Vol. IX, Part 3. Schweizerbart publishers. Stuttgart, Germany. 609 p. [ Links ]
Sala, O. E., F. S. Chapin III, J. J. Armesto, E. Berlow, J. Bloomfield, R. Dirzo, E. Huber-Sanwald, L. F. Huenneke, R. B. Jackson, A. Kinzig, R. Leemans, D. M. Lodge, H. A. Mooney, M. Oesterheld, N. LeRoy P., M. T. Sykes, B. H. Walker, M. Walker and D. H. Wall. 2000. Global biodiversity scenarios for the year 2100. Science 287(5459):1770-1774. Doi:10.1126/science.287.5459.1770. [ Links ]
Salgado N., B. (ed.). 2016. La ecología funcional como aproximación al estudio, manejo y conservación de la biodiversidad: protocolos y aplicaciones. Instituto de Investigación de Recursos Biológicos Alexander von Humboldt. Bogotá, Colombia. 236 p. [ Links ]
Sterck, F. J., L. Poorter and F. Schieving. 2006. Leaf traits determine the growth-survival trade-off across rain forest tree species. The American Naturalist 167(5):758-765. Doi: 10.1086/503056. [ Links ]
Violle, C., M.-L. Navas, D. Vile, E. Kazakou, C. Fortunel, I Hummel and E. Garnier. 2007. Let the concept of trait be functional. Oikos 116(5):882-892. Doi: 10.1111/j.0030-1299.2007.15559.x. [ Links ]
Violle, C. , B. J. Enquist, B. J. McGill, L. Jiang, C. H. Albert, C. Hulshof, V. Jung and J. Messier. 2012. The return of the variance: intraspecific variability in community ecology. Trends in Ecology Evolution 27(4):244-252. Doi: 10.1016/j.tree.2011.11.014. [ Links ]
Westoby, M., D. S. Falster, A. T. Moles, P. A. Vesk and I. J. Wright. 2002. Plant ecological strategies: some leading dimensions of variation between species. Annual review of ecology and systematics 33(1): 125-159. Doi: 10.1146/annurev.ecolsys.33.010802.150452. [ Links ]
Wellstein, C., S. Chelli, G. Campetella, S. Bartha, M. Galiè, F. Spada and R. Canullo. R. 2013. Intraspecific phenotypic variability of plant functional traits in contrasting mountain grasslands habitats. Biodiversity and Conservation 22(10): 2353-2374. Doi: 10.1007/s10531-013-0484-6. [ Links ]
Williams, L. J. and H. Abdi. 2010. Fisher’s least significant difference (LSD) test. Encyclopedia of research design 218: 840-853. [ Links ]
Williamson, G. B. and M. C. Wiemann. 2010. Measuring wood specific gravity… Correctly. American Journal of Botany 97(3):519-524. Doi: 10.3732/ajb.0900243. [ Links ]
Wright, I. J. and K. Cannon. 2001. Relationships between leaf lifespan and structural defences in a low‐nutrient, sclerophyll flora. Functional Ecology 15(3): 351-359. Doi:10.1046/j.1365-2435.2001.00522.x. [ Links ]
Wright, I. J. and M. Westoby. 2002. Leaves at low versus high rainfall: coordination of structure, lifespan and physiology. New Phytologist 155(3): 403-416. Doi: 10.1046/j.1469-8137.2002.00479.x. [ Links ]
Wright, I. J., P. B. Reich, M. Westoby, D. D. Ackerly, Z. Baruch, F. Bongers, J. Cavender-Bares, T. Chapin, J. H. C. Cornelissen, M. Diemer, J. Flexas, E. Garnier, P. K. Groom, J. Guilias, K. Hikosaka, B. B. Lamont, T. Lee, C. Lusk, J. J. Midgley, M.-L. Nvas, Ü. Niinemets, J. Oleksyn, N. Osada, H. Poorter, P. Poot, L. Prior, V. I. Pyankov, C. Roumet, S. C. Thomas, M. G. Tjoelker, E. J. Veneklass and R. Villar. 2004. The worldwide leaf economics spectrum. Nature 428:821-827. Doi: 10.1038/nature02403. [ Links ]
Wright, I. J. , N. Dong, V. Maire, I. C. Prentice, M. Westoby, S. Díaz, R. V. Gallagher, B. F. Jacobs, R. Kooyman, E. A. Law, M. R. Leishman, Ü. Niinemets, P. B. Reich, L. Sack, R. Villar, H. Wang and P. Wilf. 2017. Global climatic drivers of leaf size. Science 357(6354):917-921. Doi: 10.1126/science.aal4760. [ Links ]
Xu, F., W. Guo, W. Xu, Y. Wei and R. Wang. 2009. Leaf morphology correlates with water and light availability: What consequences for simple and compound leaves? Progress in Natural Science 19(12):1789-1798. Doi: 10.1016/j.pnsc.2009.10.001. [ Links ]
Yates, M. J., G. Anthony Verboom, A. G. Rebelo and M. D. Cramer. 2010. Ecophysiological significance of leaf size variation in Proteaceae from the Cape Floristic Region. Functional Ecology 24(3):485-492. Doi: 10.1111/j.1365-2435.2009.01678.x. [ Links ]
Zobel, M. 1997. The relative of species pools in determining plant species richness: an alternative explanation of species coexistence? Trends in Ecology& Evolution 12(7):266-269. Doi:10.1016/S0169-5347(97)01096-3. [ Links ]
Received: May 15, 2019; Accepted: December 16, 2019