Services on Demand
Journal
Article
Indicators
-
Cited by SciELO
-
Access statistics
Related links
-
Similars in SciELO
Share
Revista mexicana de ciencias forestales
Print version ISSN 2007-1132
Rev. mex. de cienc. forestales vol.7 n.37 México Sep./Oct. 2016
Articles
Climate change and prediction of fires for the year 2050 at the La Primavera Forest, Jalisco
1Departamento de Ecología, Centro Universitario de Ciencias Biológicas y Agropecuarias. Universidad de Guadalajara. México. Correo-e: fhuerta@cucba.udg.mx
During the last two decades, forest fires have increased in frequency and intensity in various parts of the world. The main causes of these patterns are associated both with land-use activities and with climate change. The La Primavera Forest is a highly important natural reserve due to its proximity to the city of Guadalajara; therefore, the prediction of fires is of great interest, as the frequency and intensity of forest fires are a major cause of deterioration of the area. The objective of this work was to build a spatial model in order to generate a fire prediction scenario for the year 2050 in the La Primavera Forest, for which purpose the data of climate projections for western Mexico and the historical occurrences of fires in the last few years were taken as a reference. The model predicts a fire zone that covers the protected area almost in its entirety. The most prominent effect of climate change in the area is associated to the reduction of precipitations and to an increase in the temperature, which results in an increase of the surface area affected by the fires. Under this scenario, the main challenge would be to aptly define the mitigation policies and strategies for promoting a healthier La Primavera Forest in terms of conservation, productivity, diversity and resilience, which will ensure the ongoing provision of ecosystemic services under a changing climate.
Key words: Environmental degradation; future scenarios; MaxEnt; Spatial model; GIS; land use
Durante las últimas dos décadas, los incendios se han incrementado en frecuencia e intensidad en diversas partes del mundo. Las principales causas de estos patrones se asocian, tanto a las actividades de uso de suelo como a los cambios en el clima. El Bosque La Primavera es un área natural protegida de gran importancia, por su cercanía a la ciudad de Guadalajara; de tal suerte que la predicción de incendios es de gran interés, ya que la frecuencia e intensidad de los mismos son una fuerte causa de deterioro de la zona. El objetivo de este trabajo fue construir un modelo espacial para generar un escenario de predicción de incendios para el año 2050 en el Bosque La Primavera, para ello se tomaron como referencia los datos de las proyecciones climáticas para el occidente de México y las ocurrencias históricas de incendios de los últimos años. El modelo predice un área de incendios que cubre casi la totalidad del polígono del área protegida. El mayor efecto del cambio climático en la zona está asociado a la disminución de las precipitaciones y a un aumento en la temperatura, lo cual incide en un incremento del área afectada por los incendios. Bajo ese escenario, el principal desafío será definir, acertadamente, las políticas públicas y estrategias de mitigación, con la finalidad de promover que el Bosque La Primavera sea más saludable, en términos de conservación, productividad, diversidad y resiliencia, lo que asegurará una provisión continua de servicios ecosistémicos bajo un clima cambiante.
Palabras clave: Degradación ambiental; escenarios futuros; MaxEnt; Modelo espacial; SIG; uso de suelo
Introduction
During the last two decades, forest fires have increased in frequency and intensity in various parts of the world. Between 1980 and 2009, there were 50 000 occurrences of fires in Europe, covering an average of 480 000 ha per year. Portugal has the highest fire density and the largest relative area of all the countries in southern Europe. Therefore, fires are the most important issue related to the forests, as they affect the landscape, the vegetation, the soil and the quality of the air (Nunes et al., 2016).
In Canada, various studies predict a profound impact of climate change on the frequency, extension and severity of fires in northern forests (Bergeron et al., 2004). In the United States of America, the forest area affected by fires has increased six-fold (Mann and Kump, 2009). The marked, sudden increase in the frequency and duration of large forest fires is associated to an abrupt change toward unusually warm springs, reduced winter precipitations, early melting of snow in the spring, and drier, longer summers (Westerling et al., 2006), while an increase of forest fires is predicted in Mexico as a result of extreme climatic variations (Dryry and Veblen, 2008; Pompa- García and Sensibaugh, 2014).
In Mexico, the next high forest fire season is predicted to begin with the next solar minimum, which will occur between 2017 and 2019 (Velasco-Herrera, 2016). Furthermore, it is estimated that precipitations will decrease by up to 10 % and temperatures will increase by 2 to 4 °C in the western region, under the harshest climate change scenario (Durán, 2010; Ibarra- Montoya et al., 2011).
At the La Primavera Wildlife Conservation Area (APFFLP, Spanish acronym), locally known as Bosque La Primavera, is one of the main protected areas in western Mexico. Due to its proximity to the Metropolitan Area of Guadalajara (ZMG, Spanish acronym), it is the main natural “lung” for this large city, and therefore poses a challenge for science within the context of the prediction of fires, as the frequency and intensity of these is a major cause of the deterioration of this area, where an average of five forest fires per year have occurred since 1949 (Semarnat, 2000).
The time of the year with the most intense fires coincides with the nesting and reproduction season of certain birds and mammals that abandon their offspring, their nests or their lairs, or perish due to the smoke and the flames.
Sugar cane production entails the periodical use of fire, which destroys not only the leaves of the crop during the harvest but also, very frequently, all of the surrounding vegetation. The surface areas planted with sugar cane increase every year, and this in turn increases the frequency of forest fires (Semarnat, 2000).
If a predictive model for fires is developed, preventive actions are bound to become more effective, even with the limited financial resources available in that protected area. Huerta-Martínez and Ibarra-Montoya (2014) provide a spatial and temporal description of a 15-year period of fires in that area and highlight the years 1984, 1987, 1995, 1998, 2005 and 2012 as critical, not only for their intensity but also for the surface area affected by them; in the last year, 7 744 ha were affected. Data provided by the Government of the State of Jalisco point out that between 10 and 20 % of the trees died as a consequence of fires (Huerta-Martínez and Ibarra-Montoya, 2014). However, no estimations of CO2 emissions due to these phenomena exist. On the other hand, the data suggest a close relationship with extreme climatic variations, specifically with the El Niño phenomenon (Huerta-Martínez and Ibarra-Montoya, 2014).
Thus, the objective of the present work was to build a spatial model in order to develop a fire prediction scenario for the year 2040 at APFFLP based on the data of the climatic projections for western Mexico published by Durán (2010) and the historical occurrences of fires described by Huerta-Martínez and Ibarra-Montoya (2014), in order to contribute information to support decision-making processes in regard to the protection of this natural reserve.
Materials and Methods
Study area
APFFLP is located in the central region of the State of Jalisco, Mexico, between the extreme coordinates 103°28’ to 103°42’ W and 20°32’ to 20°44’ N (Figure 1). APFFLP has approximately 22 points of access through dirt roads and paths, three of which are used mainly for recreational purposes. According to Köppen’s climate classification modified by García (1987), the predominant climates are temperate subhumid C (w1)(w) and semi-warm subhumid (A) C (w1)(w). The mean annual temperature is 20.6 °C, January being the coldest month, and June, the warmest. The mean annual humidity is 63 %; the predominant winds come from the southwest and reach a maximum speed of 53 km h-1 (Semarnat, 2000).
The main vegetation consists of oak, pine-oak and pine forests, tropical deciduous rainforests and induced grasslands (Semarnat, 2000).
Development of a fire prediction scenario within the context of Climate Change
Monthly and annual precipitation and maximum, minimum and mean temperature data for the years 1961-1990 (reference climatology) and 2041-2060 were used to determine the areas that might potentially catch fire at the La Primavera Forest by the year 2050. These climate data were taken from the Earth System Grid (ESG) database of WorldClim (Worldclim, 2015) and were worked in 30 arcseconds raster images, in the Idrisi Selva system (Eastman, 2012). For the 2014-2060 period, the general circulation models (GCMs) HadGEM2-AO (Hadley) and MIROC5 (Miroc) were considered, under two of the four scenarios outlined by the IPCC, which assume different levels of efforts to reduce greenhouse gas (GHG) emissions globally (rcp45 and rcp60).
These scenarios are part of the climate modeling developed by the Coupled Model Intercomparison Project, Phase 5 (CMIP5) (http://cmip-pcmdi.llnl.gov/cmip5/) (Taylor et al., 2012). The rcps refer to the radiative forcement expressed in W m-2; furthermore, it is assumed that this varies with increased GHG. RCP45 assumes a radiative forcement of ≈4.5 W m-2 and that GHG emissions will reach a peak around the year 2040, after which they will diminish. Most probably, the temperature will exceed 2 °C; while RCP60 considers part of a radiative forcement of ≈6 W m-2 with emissions that will reach the peak around the year 2080, besides the application of GHG- reducing technologies and strategies. Table 1 describes the total variables utilized.
Table 1 Climatic variables considered in the development of a fire prediction model for the year 2050 at the La Primavera Forest.
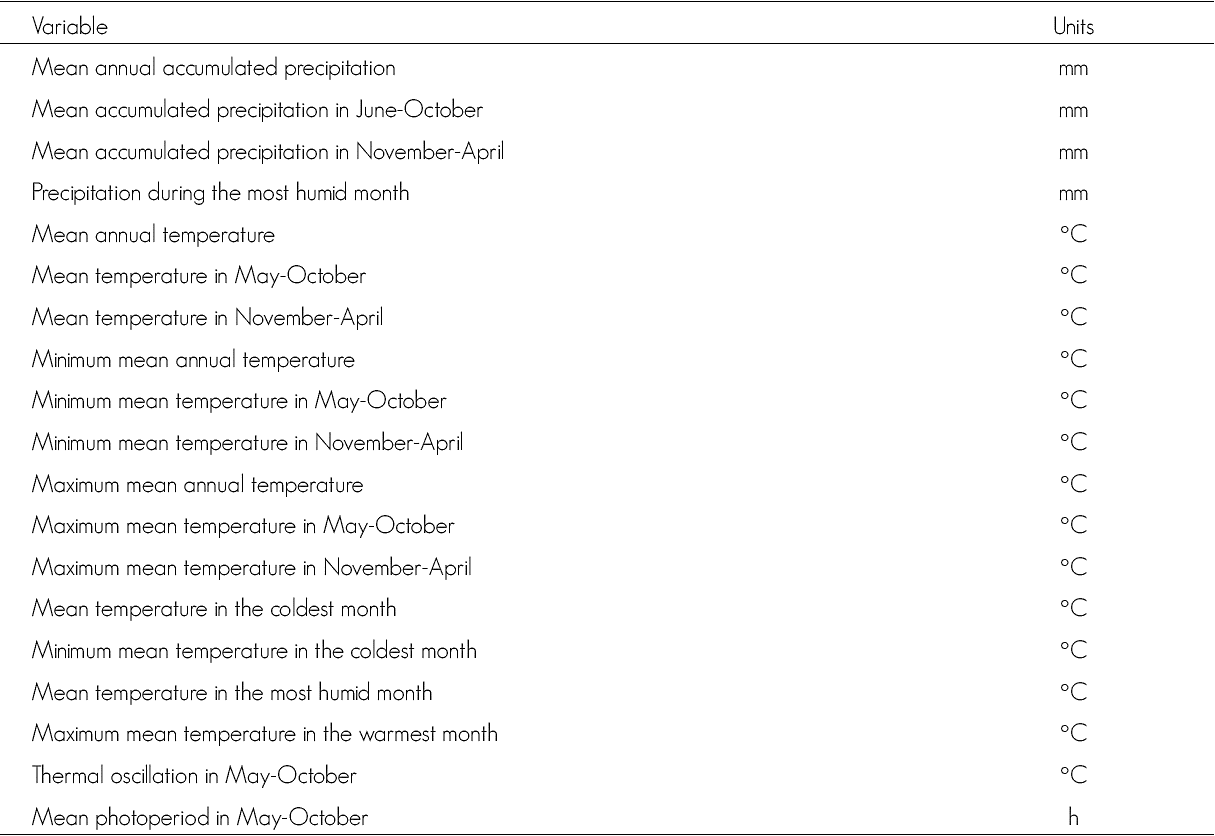
In order to build the predictive scenario, a spatial model with a maximum enthropy, version 3.33k (MaxEnt) was generated. The algorithm of this system was adapted by Steven J. Phillips and his colleagues (Phillips and Dudik, 2008; Phillips et al., 2006) for the construction of potential distribution models.
In general, the algorithm detects the non-random relationships between two sets of data: a) the georeferenced records of the occurrence of fires, and b) a set of coverages, raster images, ditigal data representing those climatic variables that are relevant for determining the potential areas where fires may occur, at a particular analysis scale (Pearson and Dawson, 2003; Phillips et al., 2006). The MaxEnt system models the distribution of fire occurrences using only data on historical events; the distribution of a fire is represented with a probability function P divided by a set of sites X in the study area.
A model of P is built with a set of restrictions derived from historical data of the occurrence of fires. The restrictions are expressed as simple functions of known climatic variables. The MaxEnt algorithm forces the mean of each function of each variable to approach the actual mean of the variable in those areas where fires occur. Out of all the possible combinations of functions, the one that succeeds in minimizing the enthropy function --measured using Shannon’s index-- is selected.
The general expressions of the function of probability for i climatic variables is:
Where:
P(x)= Function of probability
l = Weighting coefficients vector
f = Vector corresponding to functions of climatic variables
Z = Normalization constant to ensure that P(x) is equivalent to the unit.
The P(x) values obtained represent relative suitability values for the occurrence of fires and therefore constitute the base for a model of potential fires.
The model was fed the data of the occurrences of events of interest for the 1998-2012 period at APFFLP, provided by the Department of Research of the extinct Executive Direction of Bosque La Primavera--today the “Bosque La Primavera” Decentralized Pubic Agency (DPA)--and, on the other hand, the climatic variables projected for the year 2050. According to the MaxEnt model, 75 % of the occurrence records were utilized as training points, and 25 %, as validation points. A convergence threshold of 10-5 with 1 000 iterations was used as the upper limit for each run. The area under the curve (AUC) -a graphical output showing the discrimination capacity of an occurrence (sensitivity) versus the discrimination capacity of an absence (specificity)- was analyzed in order to estimate the predictive capacity of the model (Moreno et al., 2011). The analyzed output format was cumulative (0 to 100 interval), indicating an area’s relative suitability for fires per pixel, plus a probability of occurrence of a fire (logistic output).
Since the model is not deterministic, it produces somewhat different results from one run to another, even with the same data input, adjusted for the random assignation of the modeling process, 100 independent predictive models were developed for each analysis occurrence, and a subset of the 10 best models was selected based on two criteria: 1) models (20) with less than 10 % omission error, and, of these, 2) the 10 models closest to the mean were selected in areas where potential occurrences were predicted (Anderson et al., 2003). These 10 models were examined in spatial terms, and a consensus map was developed using pixel values of 0 to 10, the number 10 representing those areas where all models agree as to the fire occurrence prediction.
Results and Discussion
The spatial model had an AUC of 0.81, which is indicative of a good robustness for classifying the occurrences of fires at APFFLP (Parolo et al., 2008). The prediction of the developed spatial model shows a continuous area covering a large portion of the surface area of APFFLP (Figure 2) with a high probability of fire under greater drought and warmth conditions, indicate as a lower humidity (-10% in precipitation) and a higher temperature (+2 °C).
The construction of the spatial model is one of the few applications of spatial modeling within the context of prediction of potential fire areas (Mandallaz and Ye, 1997; García et al., 1999; Muñoz et al., 2005; Pompa-García et al., 2013; Pérez-Verdín, 2013), and so far it is the only model integrating CMIP5 variables.
The pattern of burnt areas predicted by the spatial model for the year 2050 coincides with the history of forest fires described by Huerta-Martínez and Ibarra-Montoya (2014); for this reason, climate change can be predicted to be a high-risk scenario at APFFLP, where fires are likely to become more frequent and more intense and to cover larger areas. This agrees with the predictions quoted by Bergeron et al. (2004), Westerling et al. (2006), Mann and Kump (2009), van Mantgem et al. (2009) and Allen et al. (2010), all of whom point out that the characteristics of forest fires are changing, due to global climate change among other factors.
Land-use change activities are frequent at APFFLP (Semarnat, 2000); this increases the frequency and severity of forest fires (González et al., 2011). Land-use change is promoted especially for the purpose of planting corn, agave and sugar cane. Furthermore, as larger forest areas are abandoned, young, unstable forest masses with little or no management increase. This situation, together with anthropic activity, accounts for 95 % of forest fires, some of which are intentional, besides the cultural use of fire, deeply ingrained in rural environments.
Each year, fires at APFFLP result in the burning of a large percentage of the total forest surface area (Huerta-Martínez and Ibarra-Montoya, 2014). However, fires related to so-called high-intensity extreme weather phenomena play a major role, since they develop in conditions of high-temperature and wind in combination with low humidity; therefore, these conditions are likely to occur repeatedly and very frequently.
Prominent among the environmental consequences of high-intensity fires at APFFLP are the reduction of biodiversity, increased desertification (including the degradation of soils and vegetation), and pollution of groundwater and of the atmosphere; this renders the environmental recovery of burnt areas extremely difficult and complex as, after the fire, the ecosystem is affected and the fauna must find a new natural habitat in order to survive--a somewhat involved endeavor, as the vegetal mantle disappears almost completely (Weber and Flannigan, 1997; Gitay et al., 2002). Furthermore, after intense fires, the soil becomes virtually sterile due to the mineralization of organic matter, limiting recolonization by native species.
Finally, increased erosion significantly reduces the possibilities of recovery (Pompa-García and Sensibaugh, 2014). Likewise, moderately intense fires are excellent promoters of natural repopulation; such is the case particularly of Pinus oocarpa Schiede ex Schltdl., of which there is a high density of young individuals in areas where moderate fires occurred within a period of 5 to 8 years.
As in other regions (Bergeron et al., 2004; Pausas et al., 2008; Mann and Kump, 2009; Conde et al., 2011), climate change at APFFLP is not merely theoretical, and its effects are beginning to make themselves felt. In this respect, the climatic scenario projected for 2050 resulted in an increase in the surface area affected by fires, and those areas dominated by plantations and by greatly fragmented remaining ecosystems will be particularly vulnerable, since, according to our in-field observations, these types of forests are invaded by exotic species that generate a large amount of fuel due to their high abundance and spatial distribution, as in the case of Verbesina spp.
A major priority for facing the fires predicted to occur at APFFLP is the development of an “adaptation plan” (PACMUN, 2015), understood as “the adjustment [carried out] in natural or human systems in response to actual or expected climatic stimuli and their effects, which moderate the damage or exploit beneficial opportunities”; among the latter, we may include: integrating prevention for the protection of basins against forest fires; better management of public forests, considered as primary green infrastructures; use of forest biomass for energy without reducing the carbon storage capacity, as in Italy (Keskitalo et al., 2015), thereby reducing the fuel and, thus, the risk of high-intensity fires; in other words, adopting a good practices code. Within this context, an option is the diversification of the forest, which would imply promoting the development and maintenance of various activities and uses of the territory within the APFFLP, e. g.: agricultural use, native forest management, tourism and recreation, and exclusion areas for restoration, as well as adequately assessing the ecosystemic services associated to the relevant coordination between government institutions and landowners.
In general, the forest sector in Mexico and across the world faces a change of paradigm as to the management and practices associated to fast-growing productive plantations. This entails significant political, institutional and cultural challenges to which both states and businesses will have to adapt.
Recent international initiatives, like those defined by the Forest Stewardship Council (FSC), are an example that aims at promoting and regulating the good management and sustainability of forests and plantations. In this regard, the main responsibility of the forest sector in Mexico is to promote relevant policies directed, in general, toward land use planning and, in particular, toward the diversification of the forest sector, as well as to the urgently needed sectorial institutionality, with which to face and manage the challenges imposed by society and by climate change. These actions are the first step for an effective adaptation in the face of climate change, for contributing to reduce the risk of high-intensity fires and to promote making APFFLP a healthier forest in terms of conservation, productivity, diversity and long-term resilience. According to Ibarra-Montoya and Huerta-Martínez (2016), the only major variable in the occurrence of fires in this area that can be manipulated and controlled by humans is land use; hence, it is there, that an effect must be sought in order to avoid the occurrence of first-magnitude forest fires like those of the years 1998, 2005 and 2012.
The authors hope that the present study may contribute to promote specific actions by the pertinent authorities to prevent fires, and that the efforts may be oriented toward building a close coordination between the local communities, the authorities and the academic world.
Conclusions
The spatial model developed under the climate change scenario using MaxEnt proved to be a reliable tool for predicting the areas of APFFLP where forest fires are likely to occur by the year 2050. According to the model, most of the areas have high probabilities of fires under conditions of less precipitation and a higher temperature. Thus, in the face of this scenario, there is a pressing need to develop preventive rather than corrective actions.
Conflict of interests
The authors declare that they have no conflict of interests.
Contribution by author
Francisco Martín Huerta Martínez: validated the in-field information and collected it in specific databases; he also provided the structure for the manuscript; José Luis Ibarra Montoya: revised the manuscript, carried out the spatial analysis and generated the predictive model.
Acknowledgements
We wish to express our gratitude to Biologist Karla Gutiérrez García for her valuable support in building the GIS for APFFLP, as well as for the edition of the map resulting from the modeling. To Consejo Nacional de Ciencia y Tecnología (the National Council for Science and Technology) for its support by providing Dr. Ibarra-Montoya J. L. with a post-doctoral scholarship (No. 100395).
REFERENCES
Allen, C. D., A. K. Macalady, H. Chenchouni, D. Bachelet, N. Mc-Dowell, M Vennetier, T. Kitzberger, A. Rigliing, D. D. Bres-Hears, E. H. Hogg, P. González, R. Fensham, Z. Zhangm, J. Castro, N. Demidova, J. Lim, G. Allard, S. W. Running, A. Semerci and N. Cobb. 2010. A global overview of drought and heat-induced tree mortality reveals emerging climate change risks for forests. Forest Ecology and Management 259(4): 660-684. [ Links ]
Anderson, R. P., D. Lew and A. Peterson. 2003. Evaluating predictive models of species distributions: criteria for selecting optimal models. Ecological Modelling 162: 21-232. [ Links ]
Bergeron, Y., M. Flanningan, S. Gauthier, A. Leduc and P. Lefort. 2004. Past, current and future fire frequency in the Canadian boreal forest: Implications for sustainable forest management. Ambio 33(6): 356-360. [ Links ]
Conde, C., F. Estrada, B. Martínez, O. Sánchez y C. Gay. 2011. Regional climate change scenarios for Mexico. Atmósfera 24 (1): 125-140. [ Links ]
Dryry, S. and T. Veblen. 2008. Spatial and temporal variability in fire occurrence within the Las Bayas forestry reserve, Durango, México. Plant Ecology 197: 299-316. [ Links ]
Durán, P. 2010. Modelo de distribución de los pastizales en zonas áridas de México, ante los efectos del cambio climático. Tesis de Doctorado. Universidad Nacional Autónoma de México. México, D.F., México. 163 p. [ Links ]
Eastman, J. R. 2012. Idrisi selva manual, manual versión 17. Clark Labs, Clark University. Worcester, MA, USA. 322p. [ Links ]
García, E. 1987. Modificaciones al sistema de clasificación climática de Köppen, Instituto de Geografía, UNAM, México, DF., México. 256 p. [ Links ]
García, V., S. Woodard, P. Adamowicz y B. Lee. 1999. Dos modelos para la predicción de incendios forestales en Whitecourt Forest, Canada. Investigación Agraria, Sistemas y Recursos Forestales 8(1): 5-23. [ Links ]
Gitay, A., A. Suárez, R. Watson, y J. Dokken. 2002. Cambio climático y biodiversidad. Documento Técnico V del IPCC. Ginebra, Suiza. 85 p. [ Links ]
González, M., A. Lara, R. Urrutia, y J. Busnich. 2011. Cambio climático y su impacto potencial en la ocurrencia de incendios forestales en la zona centro sur de Chile. Bosque 32 (3): 215-219. [ Links ]
Huerta-Martínez, F. M. y J. L. Ibarra-Montoya. 2014. 15 Años de incendios en el Bosque La Primavera (Jalisco, México): Un acercamiento a sus posibles causas y consecuencias. CienciaUAT 8(2): 32-43. [ Links ]
Ibarra-Montoya, J. L. y F.M. Huerta-Martínez. 2016. Modelado espacial de incendios: una herramienta predictiva para el Bosque La Primavera, Jalisco, México. Revista Ambiente & Água 1(1): 35-49. [ Links ]
Ibarra-Montoya, J. L. , R. Román, K. Gutiérrez, J. Gaxiola, V. Arias and M. Bautista. 2011. Change in land cover and land use in the north of Jalisco, Mexico: An analysis of the future in a context of climate change. Revista Ambiente & Água 6(2): 1-128. [ Links ]
Keskitalo, E. C. H., M. Legay, M. Marchetti, S. Nocentini and P. Spathelf. 2015. The Role of Forestry in National Climate Change Adaptation Policy: Cases from Sweden, Germany, France and Italy. International Forestry Review 17(1):30-42. [ Links ]
Mandallaz, D. and R. Ye. 1997. Prediction of forest fires with Poisson models, Canadian Journal of Forest Research 27(10):1685-1694. [ Links ]
Mann, M. E. and L. R. Kump. 2009. Dire predictions: understanding global warming. DK publishing. New York, NY, USA. 208 p. [ Links ]
Moreno, R., R. Zamora, J. R. Molina, A. Vásquez y M. Herrera. 2011. Predictive modeling of microhabitats for endemic birds south Chilean temperate forest using maximum entropy (Maxent) Ecological Informatics 6(6):364-370. [ Links ]
Muñoz, R., E. Treviño, J. Verástegui, J. Jiménez, y O. Aguirre. 2005. Desarrollo de un modelo espacial para la evaluación del peligro de incendios forestales en la Sierra Madre Oriental de México. Investigaciones Geográficas 56:101-117. [ Links ]
Nunes, A. N., L. Lourenço and A. C. Castro Meira. 2016. Exploring spatial patterns and drivers of forest fires in Portugal (1980-2014). Science of the Total Environment Science of the Total Environment http://dx.doi.org/10.1016/j.scitotenv.2016.03.121 . (17 de octubre de 2016). [ Links ]
Plan de Acción Climática Municipal (PACMUN). 2015. Plan de Acción Climática Municipal. http://pacmun.org.mx (23 de mayo de 2015). [ Links ]
Parolo, G., G. Rossi and A. Ferrarini. 2008. Toward improved species niche modelling: Arnica montana in the Alps as a case study. Journal of Applied Ecology 45:1410-1418. [ Links ]
Pausas, J. G., J. Llovet, A. Rodrigo and R. Vallejo. 2008. Are wildfires a disaster in the Mediterranean basin? - A review. International Journal of Wildland Fire 17(6): 713-723. [ Links ]
Pearson, R. G. and T. P. Dawson. 2003. Predicting the impacts of climate change on the distribution of species: are bioclimatic envelope models useful? Global Ecology and Biogeography 12: 361-371. [ Links ]
Pérez-Verdín, G., M. Márquez-Linares, A. Cortés-Ortíz y M. Salmerón-Macías. 2013. Análisis espacio-temporal de la ocurrencia de incendios forestales en Durango, México. Madera y Bosques 19(2): 37-58. [ Links ]
Phillips, S. J., R. P. Anderson and R. E. Schapire. 2006. Maximum entropy modeling of species geographic distributions. Ecological Modeling 190: 231-259. [ Links ]
Phillips, S. J. and M. Dudik. 2008. Modeling of species distributions with Maxent: new extensions and a comprehensive evaluation. Ecography 31:161-175. [ Links ]
Pompa-García, M., L. Vázquez-Vázquez, M. Zapata-Molina y R. Solís-Moreno. 2013. Modelo conceptual del potencial de incendios forestales en Durango: Avances preliminares. Revista Mexicana de Ciencias Forestales 3(13): 95-102. [ Links ]
Pompa-García, M. y M. Sensibaugh. 2014. Ocurrencia de incendios forestales y su teleconexión con fenómenos ENSO. CienciaUAT . 27(2): 06-10. [ Links ]
Secretaría del Medio Ambiente y Recursos Naturales (Semarnat). 2000. Programa de Manejo Área de Protección de Flora y Fauna La Primavera. Comisión Nacional de Áreas Naturales Protegidas. México, D.F., México. 132 p. [ Links ]
Taylor, K. E., R. J. Stouffer and G. A. Meehl. 2012. An Overview of CMIP5 and the experiment design. Bulletin of the American Meteorological Society 93(4):485-498. [ Links ]
van Mantgem, P. J., N. L. Stephenson, J. C. Byrne, L. D. Daniels, J. F. Franklin, P. Z. Fule, M. E. Harmon, A. J. Larson, J. M. Smith, A. H. Taylor and T. T. Veblen . 2009. Widespread Increase of Tree Mortality Rates in the Western United States. Science 323 (5913): 521-524. [ Links ]
Velasco-Herrera, G. 2016. Mexican forest fires and their decadal variations. Advances in Space Research 58(10):2104-215. [ Links ]
Weber, M. G. and M. D. Flannigan. 1997. Canadian boreal forest ecosystem structure and function in a changing climate: Impacts on fire regimes. Environmental Reviews 5(3-4): 145-166. [ Links ]
Westerling, A. L., H. G. Hidalgo, D. R. Cayan and T. W. Swetnam. 2006. Warming and earlier spring increase western U.S. forest wildfire activity. Science 313(5789): 940-943. [ Links ]
WorldClim. 2015. WorldClim. Global Climate Data. http://www.worldclim.org (08 de mayo de 2015). [ Links ]
Received: September 02, 2015; Accepted: December 07, 2016