Services on Demand
Journal
Article
Indicators
-
Cited by SciELO
-
Access statistics
Related links
-
Similars in SciELO
Share
Revista mexicana de ciencias pecuarias
On-line version ISSN 2448-6698Print version ISSN 2007-1124
Rev. mex. de cienc. pecuarias vol.14 n.4 Mérida Oct./Dec. 2023 Epub Nov 17, 2023
https://doi.org/10.22319/rmcp.v14i4.6397
Artículos
Contamination of commercial dry dog food by Aspergillus flavus and aflatoxins in Aguascalientes, Mexico
aUniversidad Autónoma de Aguascalientes. Centro de Ciencias Agropecuarias. Av. Universidad 940, Col Cd. Universitaria, 20131, Aguascalientes, Ags, México.
b Universidad Autónoma de Aguascalientes. Centro de Ciencias Básicas. México.
cInstituto Nacional de Tecnología Agropecuaria, Rafaela- Santa Fe, Argentina.
Commercial dry food (CDF) for dogs is a whole grain ration thoroughly mixed and die-cut with heat and pressure to give it the shape of kibble. CDF is formulated with several agro-industrial ingredients and by-products of agricultural and livestock origin. Contamination by Aspergillus flavus and aflatoxins (AFs) in foods has been shown to be a global problem that causes harm to human and animal health. The objective was to evaluate the presence of fungal microbiota and contamination by AFs in CDF. A random sample (n= 77) of marketed CDF was selected in Aguascalientes, Mexico. The samples were processed and cultured by serial dilutions, obtaining monosporic isolates, which were characterized morphologically, toxigenically (HPLC), and molecularly (PCR). The concentration of AFs in CDF was quantified by HPLC. Fungal growth was observed in 53.2 % of CDF, and 7.8 % exceeded the maximum permissible limit (MPL=106 CFU/g). The genera Aspergillus, Penicillium, Cladosporium, Mucor, Alternaria, and Fusarium were found (69.4, 12.9, 9.4, 4.7, 1.7, and 1.1 %, respectively). All CDF samples showed contamination by AFs (14.8 ± 0.3 μg/kg), and 11.8 % exceeded the MPL (20.0 μg/kg) suggested by the regulations; contamination was significantly associated (P<0.05) with some ingredients used, CDF moisture, and inclusion of fungicides and sequestrants. The results obtained suggest that the CDF manufacturing process does not wholly eliminate contamination by fungi or by the AFs present in the ingredients used for its formulation; consequently, these remain in the finished product, putting at risk the health of dogs and the efficacy of the food chain.
Keywords Aspergillus flavus; Aflatoxins; Food chain; Kibble
El alimento comercial seco (ACS) para perro es una ración integral completamente mezclada y troquelada con calor y presión para darle forma de croqueta. El ACS está formulado con diversos ingredientes y subproductos agroindustriales de origen agrícola y pecuario. La contaminación por Aspergillus flavus y por aflatoxinas (AFs) en los alimentos se ha demostrado que es un problema global que causa daños a la salud humana y animal. El objetivo fue evaluar la presencia de microbiota fúngica y contaminación por AFs en el ACS. Una muestra aleatoria (n=77) de ACS comercializado se seleccionó en Aguascalientes, México. Las muestras fueron procesadas y cultivadas por diluciones seriadas, obteniendo aislados monospóricos, los cuales se caracterizaron morfológica, toxigénica (HPLC) y molecularmente (PCR). La concentración de AFs en ACS se cuantificó por HPLC. En el 53.2 % de ACS se observó crecimiento fúngico y 7.8 % superaron el límite máximo permisible (LMP=106 UFC/g). Se encontraron los géneros Aspergillus, Penicillium, Cladosporium, Mucor, Alternaria y Fusarium (69.4, 12.9, 9.4, 4.7, 1.7 y 1.1%, respectivamente). Todas las muestras de ACS mostraron contaminación por AFs (14.8 ± 0.3 µg/kg) y el 11.8 % excedió el LMP (20.0 µg/kg) sugerido por la normatividad; la contaminación se asoció significativamente (P<0.05) con algunos ingredientes empleados, humedad del ACS e inclusión de fungicidas y secuestrantes. Los resultados obtenidos sugieren que el proceso de elaboración del ACS no elimina completamente la contaminación por hongos ni por las AFs presentes en los ingredientes empleados para su formulación; en consecuencia, éstos permanecen en el producto terminado poniendo en riesgo la salud de los perros y eficacia de la cadena alimenticia.
Palabras clave Aspergillus flavus; Aflatoxinas; Cadena alimentaria; Croqueta
Introduction
Commercial dry food (CDF) for dogs is a whole grain ration thoroughly mixed and die-cut by heat and pressure in the shape of kibble; it is composed of several agro-industrial products and by-products of agricultural and livestock origin, so they are important as a frequent output from agro-industrial supply chains1. In Mexico, the use of CDF has become popular to achieve the integration of dogs into the urban lifestyle; also, several brands of CDF have proliferated to meet the variety of nutritional needs of these pets, according to their activity, breed, age, and some special conditions2. In Mexico, the National Council of Manufacturers of Balanced Feed and Animal Nutrition registers 22 factories that produce 1.3 thousand tonnes of CDF annually3, which is complemented by an abundant offer of international brands4.
In the manufacture of CDF, agro-industrial products, and by-products of different bromatological compositions are incorporated to comply with its nutritional design5. The nutritional and sanitary quality of these ingredients is transferred to the final formulation, so it has been pointed out that CDF presents risks of contamination by various pathogens, such as mycotoxigenic fungi6-8. Fungal contamination occurs at multiple stages of the production of plant ingredients, such as flowering, harvesting, processing, or storage of cereals; in addition to the permanence of metabolic residues of mycotoxins in meat, dairy products, and eggs9-11.
The toxigenic fungal genera found in the ingredients for CDF formulation are Aspergillus spp., Penicillium spp., and Fusarium spp.12-14. Likewise, the mycotoxins frequently found in food ingredients are aflatoxins (AFs)12-13. The presence of AFs represents a risk factor for animal health and economic losses for agribusiness because it reduces the nutritional value of the food product15.
The poisoning of dogs by AFs causes hemodynamic, digestive, and nervous alterations, as well as changes in the biochemistry and clotting ability of the blood; which are especially sensitive to AFs because they have a reduced activity of the enzyme glutathione S transferase (GST), essential for the detoxification pathway of xenobiotics16. Although there are no specific maximum permissible limits (MPLs) for AFs in the CDF for dogs4, the MPLs established for foods intended for other domestic animals have been suggested to be used17, especially the guidelines indicated by the Codex Alimentarius or by the European Community (20.0 or 5.0 μg/kg, respectively)17,18.
Reports of outbreaks of clinical forms due to AFs poisoning in dogs are scarce, but their geographical distribution is very diverse: North America, Latin America, Asia, and Africa19-21. This coincides with a worldwide distribution of toxigenic fungi both in CDF and in the ingredients with which they are made22,23. In addition, the way CDF is dispensed, in generally large bags or sacks, allows the increase in the concentration of AFs, because the dog must ingest all the content that is in each bag, but the fungal spores and toxins are resistant to the manufacturing process2. In summary, the presence of toxigenic fungi and their toxins can be considered a severe problem for the dog to adequately perform its zootechnical function as a companion, guardian, or sports animal. In addition, national and international agribusiness must carry out better strategies to reduce fungal contamination and its mycotoxins in CDFs. Therefore, this study aimed to evaluate the presence of fungal microbiota and contamination by AFs in the CDF for dogs.
Material and methods
Study design
The study was conducted in Aguascalientes, Mexico (22°27'35 - 21°37'20" N; 101°50'07" - 102°52'27" W). The climate is semi-dry with an average annual temperature of 18 °C, with an average rainfall of 526 mm, and the main rainy season in summer24. A list of shopping centers, pet stores, veterinarians, and grocery stores that sold CDF was obtained, and a visit was made to get information on the brands and types in the establishments. A total of 145 types of CDF (Table 1) were found, which were considered as a sampling frame. The sample size was calculated in 58 types of CDF using the following formula to estimate proportions in a finite population25:
Where: n= sample size (58); N= population size (145 types of CDF); Z= standard normal distribution value (1.96); p= prevalence or expected proportion of contamination with Aspergillus spp. or with AFs in the CDF, a proportion value P= 0.5 was used, q= 1-p; d= desired precision (maximum error= 0.10).
Table 1 Characteristics of commercial dry dog food marketed in central Mexico
Type of food | Supply | Sampling | Protein | Moisture | Fiber | Price | |
---|---|---|---|---|---|---|---|
(N) | (n) | (n/N%) | (min. %) | (max. %) | (max. %) | *US$/kg±(SE) | |
Origin | |||||||
National | 120 | 64 | 53.3 | 24.0 | 11.0 | 4.0 | 3.9b ±0.24 |
International | 25 | 13 | 52.0 | 26.0 | 11.0 | 4.0 | 5.9a ± 0.92 |
Commercial classification | |||||||
Standard | 87 | 52 | 59.8 | 22.0 | 12.0 | 5.0 | 2.6b ± 0.17 |
Premium | 58 | 25 | 43.1 | 27.0 | 11.0 | 4.0 | 7.7a ± 0.37 |
Prescription (Age) | |||||||
Puppy | 55 | 27 | 49.1 | 27.0 | 11.0 | 4.0 | 5.1a ± 0.45 |
Adult | 90 | 50 | 55.6 | 22.0 | 11.0 | 4.0 | 3.7b ± 0.30 |
Prescription (Size) | |||||||
General | 72 | 43 | 59.7 | 22.0 | 12.0 | 5.0 | 2.2a ± 0.10 |
Specific | 73 | 34 | 46.6 | 26.0 | 11.0 | 4.0 | 6.8a ± 0.39 |
Total | 145 | 77 | 53.1 |
*Price in reference US dollars (www.banxico.org.mx: January 2020).
ab Means with different literal show significant differences (P<0.05).
The selection of samples was performed using the snowball sampling technique26, for which the establishments were visited successively in alphabetical order, and samples of CDF sold were acquired. The purchase of the CDF was suspended when the same types that had been previously acquired were found in three successive stores. Finally, 77 different types of CDF were purchased (Table 1).
The type of CDF was classified according to the prescription (age and size) and commercial identification (standard and premium) declared by the manufacturer. The composition of the CDFs was recorded from the nutritional information reported by the manufacturer to identify the ingredients used. The CDFs were classified by the presence or absence of cereals, oilseeds, vegetable oil, legumes, tubers, animal by-products, fungicides, and ingredients with sequestering capacity and type of CDF.
Sample handling
The samples were dried in an oven with forced air circulation and pulverized (500-800 μm) in a universal continuous mill and stored inside sealed bags in refrigeration (4-5 °C) until processing (<2 wk).
The fungal isolation was performed using the direct plating technique with serial dilution for the count of fungal colonies in the CDF. Samples were diluted (10-1, 10-2, 10-3, and 10-4) and seedings were performed on rose bengal agar + chloramphenicol and Czapek. The incubation period in the dark was 27-30 °C for 7 d27. Preparations of fungal colonies were made with cotton blue staining using lactophenol to observe microscopic characteristics28. The isolates were identified with macroscopic and microscopic morphological characteristics29,30.
Molecular analysis
Genomic DNA was extracted from monosporic isolates consistent with the morphology of A. flavus using previously standardized methods31. The (1 %) agarose gel electrophoresis technique was used to verify the quality of the DNA obtained. DNA samples were deposited in the gel with loading buffer (Platinum II Green PCR Buffer 5X Thermo Fisher Scientific, Waltham, MA, USA) to place them in the electrophoretic chamber with loading buffer (TAE 1X, 95 volts, 40 min). The resulting bands were observed in an image documenter (GEL DOC XR, BIO-RAD Molecular Image CA, USA) with the Quantity One software (version 4.6.7.).
Polymerase chain reaction (PCR) was performed to amplify genomic DNA fragments in the region of internal transcribed spacers (ITS1-5.8S-ITS2- rRNA), calmodulin gene (CaM), and the primer gene of the aflatoxin biosynthetic pathway (aflR) following previously described protocols32,33. The following primers were used for ITS1: 5´-TCCGTAGGTGAACCTGCGG-3´; ITS4: 5´-TCCTCCGCTTATTGATATG -3´; CMDA7-F: 5´-GCCAAAATCTTCATCCGTAG-3´; CMDA8-R: 5´-ATTTCGTTCAGAATGCCAGG-3´; aflR-F: 5´-GGGATAGCTGTACGAGTTGTGCCAG-3´; aflR-R: 5´-TGGKGCCGACTCGAGGAAYGGGT-3´ (Thermo Fisher Scientific, Waltham, MA, USA).
The enzyme Taq-polymerase (Platinum Green Hot Start PCR 2X Master Mix, Thermo Fisher Scientific) was used for amplification, and amplification reactions were performed in a thermal cycler (Labnet, Multigene, USA). The amplification protocol was introduced for the ITS1-5.8S-ITS2 RNAr region using a denaturation period of 3 min at 94 °C, followed by 35 cycles (denaturation at 94 °C/1 min, annealing at 54 °C/1 min and extension at 72 °C/1 min) and with a final extension of 9 min at 72 °C. The conditions for amplification of the CaM gene were with a denaturation period, a cycle of 1 min/94 °C followed by 30 cycles (1 min/94 °C, for annealing 1 min/53 °C and for extension 1 min/72 °C) and a final extension period of 10 min at 72 °C was added. Likewise, for the amplification of the aflR gene, a pre-denaturation period of 1 min at 94 °C was used, followed by 35 cycles (denaturation at 94 °C/1 min, annealing 63 °C/1 min, and extension 72 °C/1 min) and with a final extension of 10 min at 72 °C. The quality of the PCR products (ITS, CaM, and aflR) was verified by the 1 % agarose gel electrophoresis technique. A ladder with a marker of molecular weight (1.0 μL, 100 bp DNA ladder, 0.5 μg/μL. No. 15628019/15628050. Invitrogen DNA Ladder) together with 1.0 μL of the buffer (BlueJuice Gel Loading Buffer 10X) were included. The size in base pair (bp) of the amplicon for molecular identification was: ITS, 600-800; Calm, 468 and aflR, 796. The bands were visualized in the image documenter using the Quantity One software (version 4.6.7.). PCR products were purified with the ExoSAP-IT PCR Product Cleanup reagent (Affymetrix, Thermo Fisher Scientific Inc. Santa Clara, California, USA).
Mycotoxin quantification
The quantification of the concentration of AFs was performed in duplicate according to the AOAC official method 990.3334. The content of AFs was extracted using solid phase tubes (SPE; SupelcleanTM LC-18 SPE tube, Sigma-Aldrich, USA), methanol:water, acetic acid, tetrahydrofuran (THF), and hexane. Trifluoroacetic acid-derived extracts were injected into an HPLC system with a fluorescence detector (Varian Pro Star binary pump; FP detector 2020, Varian Associates Inc., Victoria, Australia), C18 column and column protector (LC-18 and LC-18; Thermo Fisher Scientific, Waltham, MA, USA). AFs estimates were obtained with the help of a software (Galaxie Ver. 1.9.302.530), and concentrations were calculated using standard curves of purified AFs (Sigma Aldrich, St. Louis, MO, USA).
The following mycotoxins were also quantified in the CDF: zearalenone (ZEA), ochratoxin (OTA), fumonisins (FUM), and deoxynivalenol (DON) by indirect ELISA analysis4 (Ridascreen Fast: Zearalenon R5502, Fumonisin R5602, Ochratoxin A R5402, Deoxynivalenol R5902, R-Biopharm, Germany).
Statistical analysis
Data were analyzed using a normality test with the Kolmogorov-Smirnov method at a 95 % confidence level. The comparison of the sample means for each variable was performed by means of the Tukey test (HSD) with a statistical software (Statgraphics Centurion, version 16.1.03). To identify the risk of exceeding the MPL established for the concentration of AFs, the Chi-square test (χ2) of the probability ratio or odds ratio (OR) was performed, calculating the portion of CDF that exceeded the MPL for the concentration of AFs and that was exposed to a specific factor (formulation with the inclusion of cereals, oilseeds, vegetable oil, legumes, tubers, by-products of animal origin, fungicides and ingredients with sequestering capacity and type of CDF) divided by the portion of CDF that exceeded the MPL for the concentration of AFs but was not exposed to that specific factor. A probability level of P<0.05 was considered in all analyses.
Results
Most CDF acquired (82.8 %) were manufactured by national manufacturers, while 17.2 % were made by international commercial brands (Table 1). More than half of the CDF samples (41/77= 53.2 %) had fungal contamination, while 7.8 % (6/77) contained a fungal concentration above the maximum recommended levels (106 CFU/g). Eighty-five (85) purified fungal isolates were obtained, which showed morphological characteristics corresponding to the following main toxigenic genera (Figure 1): Aspergillus spp. (69.4 %), Fusarium spp. (1.1 %) and Penicillium spp. (12.9 %). Isolates with morphology corresponding to the genera Cladosporium spp., Mucor spp., and Alternaria spp. (9.4, 4.7, and 1.7 %, respectively) were also identified. Of the isolates of Aspergillus spp., 40.7 % (24/59) corresponded to the morphology of A. flavus30; 75.0 % (18/24) of the A. flavus isolates demonstrated in vitro the capacity to produce aflatoxins (9.8 ± 0.64 μg/kg in 7 d) and also expressed the CaM and aflR genes and the ITS region (Figure 2) by PCR analysis.
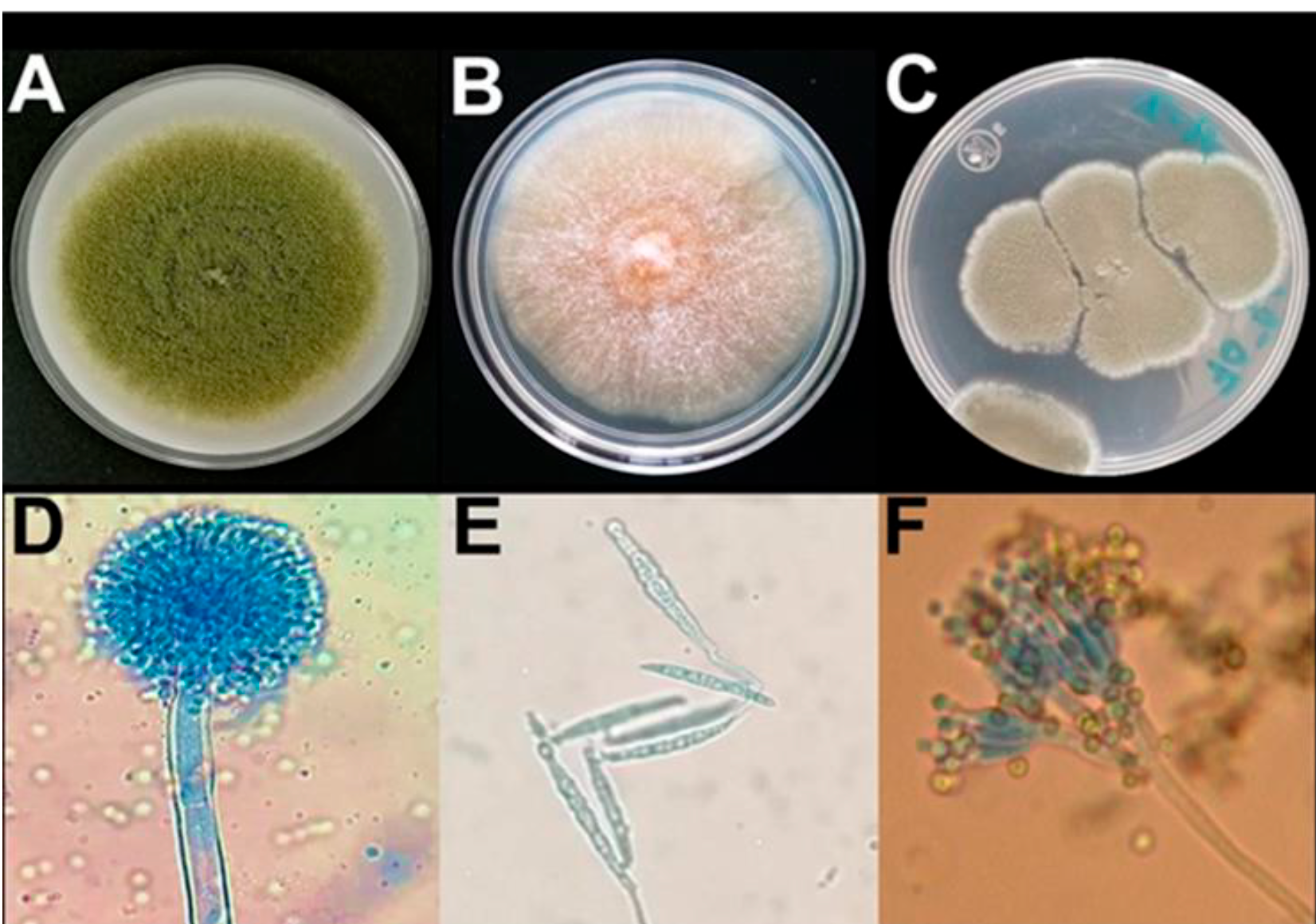
Figure 1 Macroscopic and microscopic morphological structure (40x) of monosporic isolates. Panels: A) Aspergillus spp., B) Fusarium spp., C) Penicillium spp., D) Aspergillus spp., E) Fusarium spp., and F) Penicillium spp.
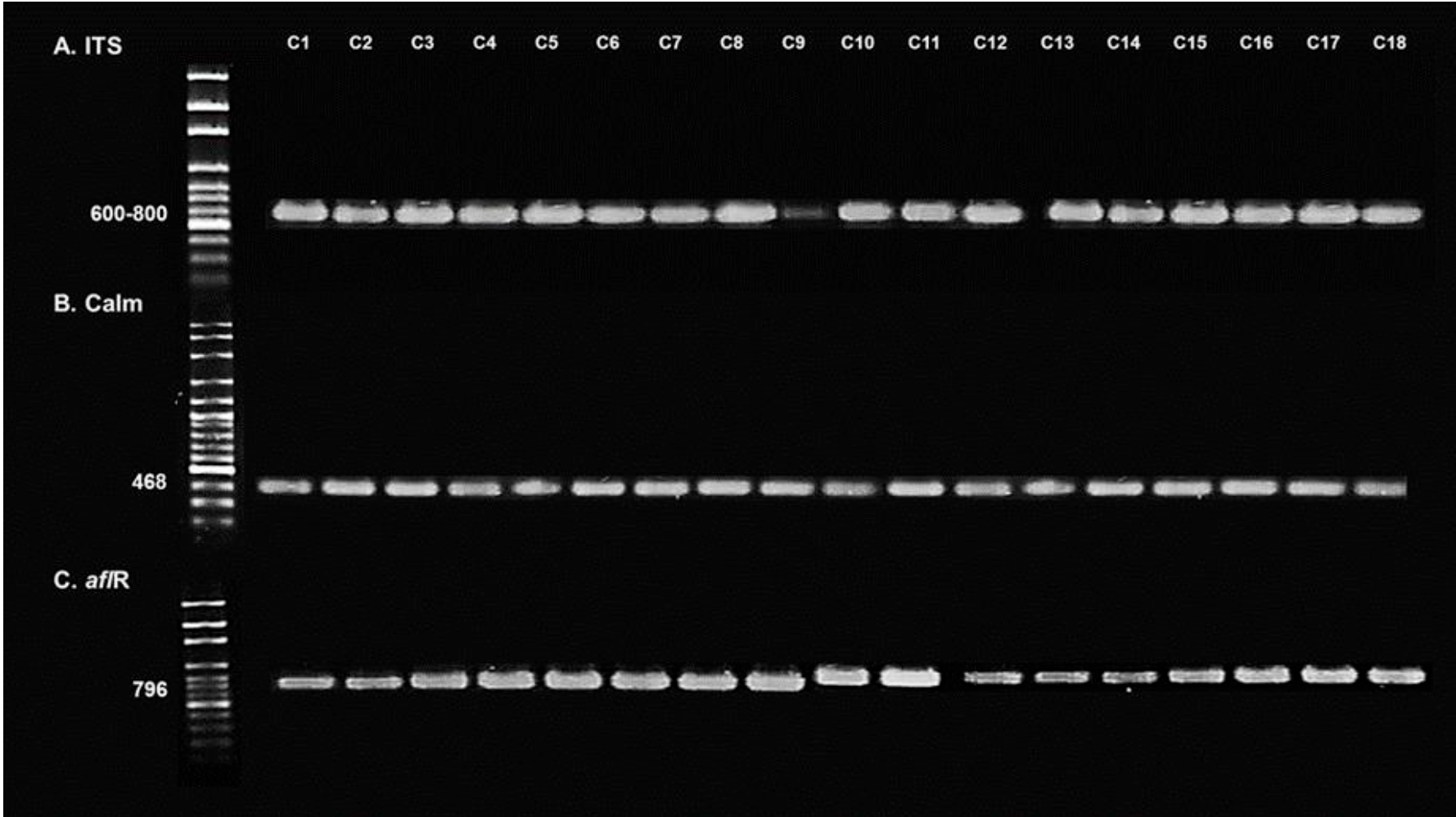
Panel A: amplification of the internal transcribed spacer (ITS) region. Panel B: amplification of Calmodulin (Calm). Panel C: amplification of the primer gene of the aflatoxin biosynthetic pathway (aflR). First lane: 100-2000 base pair molecular weight marker; C1-C18: isolates of Aspergillus flavus.
Figure 2 1 % agarose gel electrophoresis of PCR products
All CDF samples showed detectable concentrations of AFs (Figure 3). The frequency of AFs concentration showed a normal approximation (P=0.14); the minimum concentration was 8.6 μg/kg and the maximum concentration was 22.2 μg/kg; a mean concentration of 14.8 ± 0.3 μg/kg was estimated, with a 95.0 % confidence interval of 14.2-15.4 μg/kg. It was also detected that approximately one in ten (11.8 %) of the CDF analyzed exceeded the MPL of AFs recommended by most legislations of American countries for the use of cereals (20.0 μg/kg)35, while all the CDF exceeded the European recommendations (5.0 μg/kg)18. Concentrations of OTA, FUM, and DON were below detection limits; while the estimated concentrations of ZEA (228 ± 13.8 μg/kg) in no case exceeded the MPL (400 μg/kg) suggested to regulate this mycotoxin35.
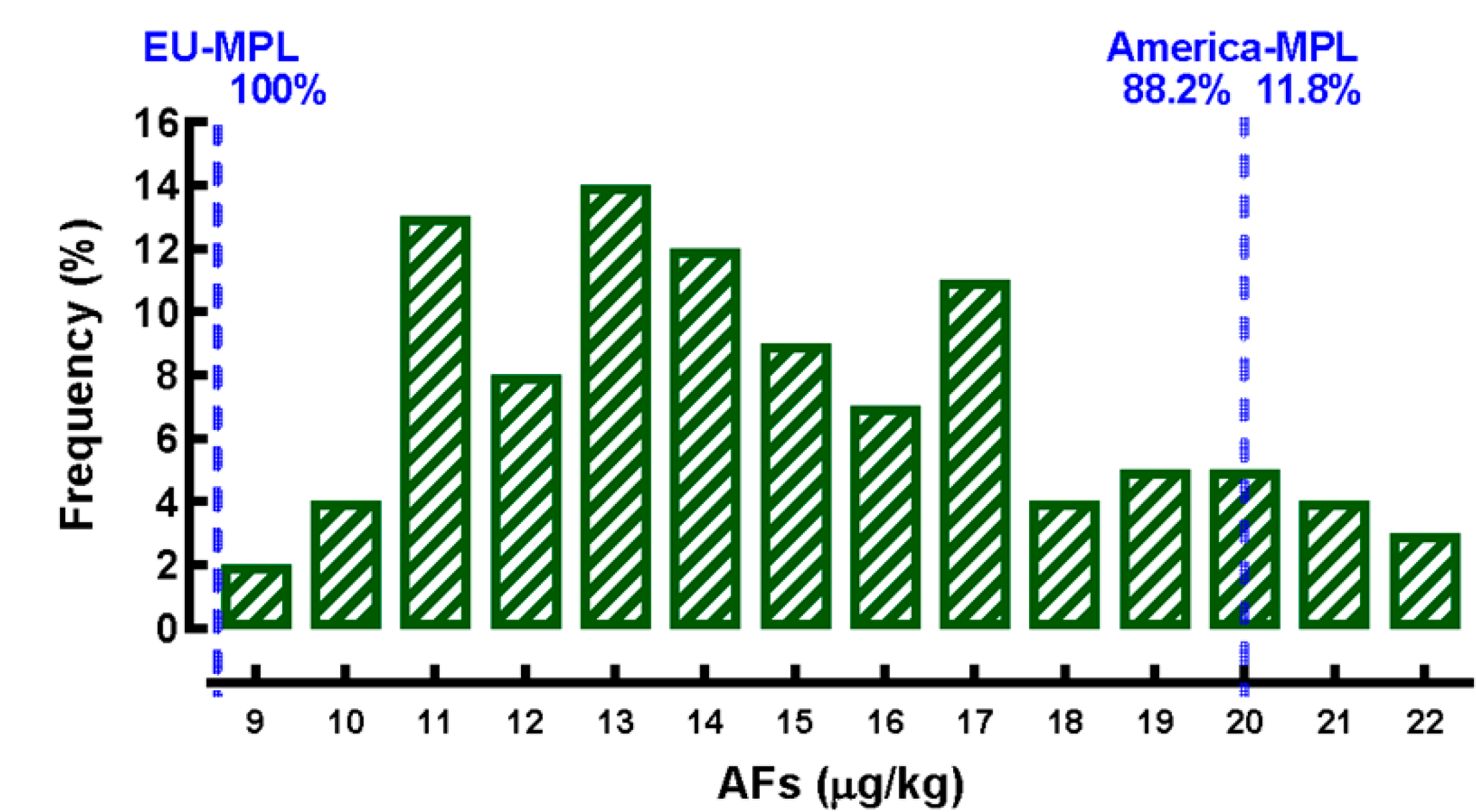
MPL= maximum permissible limit (MPL): Americas (20 μg/kg); European Union (5.0 μg/kg).
Figure 3 Frequency of the concentration of aflatoxins (AFs) in commercial dry dog food in central Mexico
In this study, no significant difference (P>0.05) was observed between the concentration of AFs and the general characteristics of the CDF, such as origin, commercial classification (standard or premium), or prescription by age or size of the dog; nor was any statistical association detected that would allow these characteristics to be identified as risk factors that generate concentrations above the MPL (Table 2).
Table 2 Association between commercial dry dog food characteristics and aflatoxin concentration
Type of food | (n) | Mean | ±SE | >MPL | P value | OR |
---|---|---|---|---|---|---|
(µg/kg) | (%) | (χ2) | ||||
Origin | ||||||
National | 64 | 15.0a | ±0.32 | 12.5 | 0.49 | 1.71 |
International | 13 | 13.9a | ±0.61 | 7.7 | ||
Commercial classification | ||||||
Standard | 52 | 15.1a | ±0.34 | 11.5 | 0.93 | 0.95 |
Premium | 25 | 14.2a | ±0.50 | 12.0 | ||
Prescription (age) | ||||||
Puppy | 27 | 15.0a | ±0.48 | 18.5 | 0.05 | 2.6 |
Adult | 50 | 14.7a | ±0.35 | 8.2 | ||
Prescription (size) | ||||||
General | 43 | 15.3a | ±0.38 | 14.0 | 0.32 | 1.7 |
Specific | 34 | 14.2a | ±0.42 | 8.8 |
SE= standard error; MPL= maximum permissible limit (20 μg/kg); P(χ2)= Chi-square; OR= odds ratio.
ab Means with different literal show significant differences (P<0.05).
The average concentration of AFs in the CDF presented significant differences associated with the characteristics of the CDF and with the ingredients used. The CDF with moisture higher than 10 % showed an estimated concentration of AFs significantly higher (P<0.05) than that in CDF containing lower moisture. It was also detected that there was a significant three times higher risk (OR χ2 P<0.05) of finding concentrations above the MPL in those CDF that registered moisture greater than 10 % (Table 3) in relation to the CDF that had moisture less than 10 %. Although it was also detected that there was a higher risk for CDF that contained a higher concentration of protein, fat, and ash (>22, >12, and >7 %, respectively) in their formulation, the statistical association was not significant (P>0.05).
Table 3 Association between bromatological analysis of commercial dry dog food and aflatoxin concentration
Characteristic | (n) | Mean | ±SE | >MPL | P value | OR |
---|---|---|---|---|---|---|
(µg/kg) | (%) | (χ2) | ||||
Relative moisture | ||||||
>10% | 31 | 17.4a | ± 0.36 | 19.4 | 0.01 | 3.4 |
≤10% | 46 | 13.0b | ± 0.29 | 6.5 | ||
Protein | ||||||
>22% | 47 | 14.9a | ± 0.36 | 14.9 | 0.12 | 2.5 |
≤22% | 30 | 14.7a | ± 0.46 | 6.7 | ||
Fat | ||||||
>12% | 22 | 14.9a | ± 0.53 | 18.2 | 0.11 | 2.2 |
≤12% | 55 | 14.8a | ± 0.34 | 9.1 | ||
NFE | ||||||
Present | 47 | 14.4a | ± 0.52 | 12.8 | 0.78 | 1.1 |
Absent | 107 | 15.0a | ± 0.34 | 11.2 | ||
Fiber | ||||||
>4% | 30 | 15.2a | ± 0.46 | 13.3 | 0.61 | 1.3 |
≤4% | 47 | 14.5a | ± 0.36 | 10.6 | ||
Ash | ||||||
>7% | 38 | 15.5a | ± 0.40 | 15.8 | 0.11 | 2.3 |
≤7% | 39 | 15.1a | ± 0.39 | 7.7 |
SE= standard error; MPL= maximum permissible limit (20 μg/kg); P(χ2)= Chi-square; OR= odds ratio; NFE= nitrogen-free extract.
ab Means with different literal show significant differences (P<0.05).
The average concentration of AFs in the CDF that contained wheat was significantly higher (P<0.05) compared to the estimated concentration of AFs in the CDF that did not use this ingredient (Table 4). Nevertheless, when calculating the risk of exceeding the MPL, there was no significant association (P>0.05) between the proportion of CDF that contained wheat and those that did not include it in their formulation. No significant difference (P>0.05) was observed between the AFs concentration means in the presence or absence of any by-product of animal origin in the CDF. However, a significant association (P<0.05, χ2) was detected in the proportion of CDF that exceeded the MPL among the CDF that presented fishmeal and fish oil in their formulation, compared to those that did not; therefore, the risk (OR) of finding concentrations above the MPL was three times higher than in the CDF that registered absence of the ingredients. All the samples purchased used meat and bone meal in the formulation of CDF, so no association with these ingredients could be established.
Table 4 Association between aflatoxin concentration and the inclusion of agro-industrial foods and by-products in commercial dry dog food
Ingredient | (n) | Mean | ± SE | >MPL | P value | OR |
---|---|---|---|---|---|---|
(µg/kg) | (%) | (χ2) | ||||
Wheat | ||||||
Present | 47 | 15.5a | ± 0.35 | 12.8 | 0.60 | 1.3 |
Absent | 30 | 13.8b | ± 0.44 | 10.0 | ||
Barley | ||||||
Present | 22 | 15.5a | ± 0.53 | 18.2 | 0.11 | 2.2 |
Absent | 55 | 14.5a | ± 0.33 | 9.1 | ||
Corn | ||||||
Present | 55 | 15.0a | ± 0.34 | 9.1 | 0.11 | 0.45 |
Absent | 22 | 14.3a | ± 0.53 | 18.2 | ||
Rice | ||||||
Present | 38 | 15.2a | ± 0.40 | 13.2 | 0.57 | 1.4 |
Absent | 39 | 14.4a | ± 0.40 | 10.3 | ||
Oilseeds | ||||||
Present | 27 | 15.1a | ±0.48 | 14.8 | 0.37 | 1.6 |
Absent | 50 | 14.6a | ±0.35 | 10.0 | ||
Vegetable oil | ||||||
Present | 38 | 14.8a | ±0.41 | 13.2 | 0.57 | 1.3 |
Absent | 39 | 14.8a | ±0.40 | 10.3 | ||
Legumes | ||||||
Present | 49 | 14.8a | ±0.36 | 12.2 | 0.77 | 1.1 |
Absent | 28 | 14.8a | ±0.47 | 10.7 | ||
Tubers | ||||||
Present | 45 | 14.9a | ±0.37 | 13.3 | 0.45 | 1.5 |
Absent | 32 | 14.7a | ±0.44 | 9.4 | ||
Egg and milk | ||||||
Present | 27 | 15.1a | ±0.48 | 18.5 | 0.05 | 2.6 |
Absent | 50 | 14.7a | ±0.35 | 8.0 | ||
Fishmeal and fish oil | ||||||
Present | 31 | 15.3a | ±0.45 | 19.4 | 0.01 | 3.4 |
Absent | 46 | 14.5a | ±0.37 | 6.5 |
SE= standard error; MPL= maximum permissible limit (20 μg/kg); P(χ2)= Chi-square; OR= odds ratio.
ab Means with different literal show significant differences (P<0.05).
CDF that contained fungicides or mycotoxin mineral sequestering agents showed significantly lower mean AFs concentration (P<0.05) compared to the estimated concentration of AFs in CDFs where these additives were not included (Table 5). In addition, a significant protective association (P<0.05, χ2) was detected when comparing the proportion of CDF that exceeded the MPL but did not include these components and those that did add them to their formulation, so the risk (OR) of presenting concentrations above the MPL was lower than in the CDF that included fungicides or sequestering agents.
Table 5 Association between the inclusion of fungicides and sequestering agents with the concentration of aflatoxins in commercial dry dog food
Ingredient | (n) | Mean | ± SE | >MPL | P value | OR |
---|---|---|---|---|---|---|
(µg/kg) | (%) | (χ2) | ||||
Fungicides | ||||||
Present | 46 | 13.5a | ± 0.33 | 6.5 | 0.01 | 0.29 |
Absent | 31 | 16.7b | ± 0.40 | 19.4 | ||
Organic adsorbents | ||||||
Present | 43 | 14.7a | ± 0.38 | 9.3 | 0.30 | 0.59 |
Absent | 34 | 14.9a | ± 0.43 | 14.7 | ||
Mineral sequestrants | ||||||
Present | 43 | 13.9a | ± 0.36 | 7.0 | 0.04 | 0.35 |
Absent | 34 | 15.9b | ± 0.41 | 17.7 |
SE= standard error; MPL= maximum permissible limit; P(χ2)= Chi-square; OR= odds ratio.
ab Means with different literal show significant differences (P<0.05).
Discussion
Commercial dry food or kibble has represented an important market for various industries that produce food for dogs incorporated into the urban lifestyle3. As in other products of agricultural and animal origin, contamination by fungal microbiota and mycotoxins is virtually inevitable11. The present study detected contamination by toxigenic Aspergillus flavus in one third (18/77= 31.2 %) of a random sample of CDF, as well as a detectable concentration of aflatoxins in all samples; in addition, 11.8 % of the CDF exceeded the maximum permissible limit of AFs (20.0 μg/kg) suggested by the regulations35. This finding has not been previously reported in Mexico and contamination by AFs puts at risk the health of dogs and the proper performance of their zootechnical function (company, guard, work, etc.) for which they are raised36. Likewise, it economically affects the agro-industrial branches that provide the ingredients by altering the safety of the product and deteriorating its economic and nutritional value15.
In this study, it was found that CDF had low to moderate concentrations of other mycotoxins. The levels of OTA, FUM and DON were estimated to be below the detection limits. The concentration of ZEA reached concentrations close to half (57.0 %) the maximum permissible level used in European countries that regulate this mycotoxin (400 μg/kg)35; however, this finding of absence of significant concentrations of mycotoxins other than AFs does not guarantee that these contaminants could not be present in other circumstances, because mycotoxins are common contaminants in cereals that are used as common ingredients in the manufacture of dog food37; this suggests that CDF manufacturing should be properly managed due to the severity of mycotoxin contamination38.
Although the information on the presence of A. flavus and AFs in various ingredients in human food is extensive, studies of contamination in CDF are scarce, despite being formulated with similar ingredients2. In this study, Aspergillus spp. was the genus detected most frequently (69.4 %) in CDF, which agrees with several authors12,14,39 who identified the same fungal genera contaminating CDF for dogs in other countries. In the present study, fungal microbiota was found in 53.2 % of the samples, and 7.8 % exceeded the maximum concentration of fungi (106 CFU/g) suggested as the maximum permissible40. The confirmation of the identity of isolates with the morphology of A. flavus was achieved by amplification of genes and gene regions (ITS, CaM, and aflR), which has been proposed as a default barcode for the identification of these fungi with the capacity to produce AFs41,42. These findings suggest that the persistence of active forms of fungi with toxigenic capacity means an additional risk since if the usual processes of kibble production are not able to destroy the fungal microbiota, when the environmental conditions (water activity and temperature) change due to the opening of the bags where the finished product is stored, the spores and sclerotia of A. flavus can give rise to new vegetative forms capable of using food substrates, producing aflatoxins and increasing the pre-existing concentration in CDF43. In addition, the usual amount of CDF contained in the bag is sufficient for a duration of several days or weeks in which the dog has to consume all the material, regardless of its quality and safety44.
In this study, a significant association was found between some characteristics of CDF and the detected concentration of AFs, which was reinforced by the estimation of the increase in the risk of exceeding the MPL. Especially relative moisture above 10 % showed three times more risk of presenting concentrations above the MPL compared to foods with a lower relative moisture (Table 3). This finding coincides with other studies that report that the activity of water present in the food matrix is a relevant factor for the expression of genes regulating the AFs biosynthesis pathway45. Therefore, if the substrate contains more moisture or is rehydrated during storage, AFs concentrations may increase46. This result could be attributed to the fact that the extruded raw material for the formulation of CDF presents in the initial stage of the process an excess of relative moisture content (20-25 %), and although it is reduced by drying to low levels (8-12 %), only the growth of the vegetative forms of the fungal microbiota is inhibited, but its spores and mycotoxins produced within the processed material remain stable45.
The results of this study also showed that there was greater contamination by AFs in the presence of some ingredients used in the manufacture of the food. The CDFs that contained wheat or fishmeal and fish oil had higher concentrations of AFs or a higher risk of exceeding the MPL. In the case of cereals, contamination has been attributed to crop exposure at various stages of production (flowering, harvesting, transport, processing, or storage)47. These ingredients are widely used as a source of carbohydrates, fiber, proteins, fats, minerals, and vitamins48; on the other hand, fishmeal and fish oil are products resulting from the processing of whole fish or by-products (cooking, pressing, dehydration and milling) and constitute a source of protein that is rich in fatty acids of high nutritional value (eicosapentaenoic acid, docosahexaenoic acid, and omega-3 acid)49. These ingredients are included in formulas because of their low cost and because they maintain an acceptable nutritional value for the dog’s physiology, in addition, their inclusion does not affect the palatability and digestibility of nutrients38. This suggests that AFs contamination may be common in CDF with the presence of cereals or fish by-products50,51. Therefore, the quality of these ingredients should be guaranteed, and proper handling and effective process management of the finished product should be carried out to ensure protection against contamination by AFs52.
The results of this study showed that CDF that included fungicides or mineral sequestrants in their formulation had both a lower mean concentration of AFs and a lower percentage of AFs above the MPL (P<0.05) compared to those that did not, which suggest a protective association of these agents against the risk of AFs contamination higher than the MPL. This finding suggests that the use of fungicidal and sequestering agents is a helpful method to reduce the toxic effects of AFs, since fungicides have an inhibitory effect on fungal growth by acidifying their cytoplasmic content53; while mineral sequestrants exert their protective association through β-dicarbonyl chemisorption of AFs, which reduces their bioavailability through gastrointestinal absorption54,55.
Surprisingly, in this study, no association (P>0.05) was found between the concentration of AFs or the proportion that exceeded the MPL suggested by the regulations (Table 2) against some characteristics considered as evidence of quality by users (premium foods, international origin, or higher price). This suggests that consumer confidence is based on criteria other than the safety of CDF, such as marketing diffusion, supposed association between quality and price, palatability, or appearance2.
Conclusions and implications
In the present study, considerable contamination by toxigenic Aspergillus flavus was detected, as well as a significant concentration of aflatoxins in all samples collected in a random and representative sampling of commercial dry dog food. These findings suggest that the health of dogs and the proper development of their zootechnical function are at risk, and it could also affect the agro-industrial branches that provide this food since the safety of the product is altered, and its economic and nutritional value deteriorates. The results of the study indicated that some bromatological characteristics and the formulation used in the preparation of CDF generated a greater risk of contamination by fungi and mycotoxins; it follows that there is a need to design and implement more effective strategies to verify the safety of ingredients and processes used in the manufacture of CDF. In addition, the establishment of maximum permissible levels of AFs specific for CDF and research on prolonged exposure of dogs to low concentrations of mycotoxins should be encouraged.
Acknowledgements
The authors wish to thank the funding from the National Council of Science and Technology of Mexico (CONACYT No. 738906) and the Autonomous University of Aguascalientes (project No: PIP/SA 22-2).
REFERENCES
1. Carranza-Trinidad R, Valdivia-Flores AG. Supply chain: An input-output perspective. An example of application in the dairy products industry. Int J Supply Chain Oper Resil 2018;3(3):236-247. doi: 10.1504/IJSCOR.2018.093258. [ Links ]
2. Martínez-Martínez L, Valdivia-Flores AG, Guerrero-Barrera AL, Quezada-Tristán T, Rangel-Muñoz, EJ, Ortiz-Martínez, R. Toxic effect of aflatoxins in dogs fed contaminated commercial dry feed: A Review. Toxins 2021;13(1):65. doi:10.3390/toxins13010065. [ Links ]
3. CONAFAB (Consejo Nacional de Fabricantes de Alimentos Balanceados y de la Nutrición Animal). Producción pecuaria y alimento balanceado en México. México. 2021. ULR: https://www.conafab.org/informativos/anuario-estadistico. [ Links ]
4. Kara K. Comparison of some mycotoxin concentration and prevalence in premium and economic class of adult dog foods. Ital J Anim Sci 2022;21(1):1380-1389. doi.org/10.1080/1828051X.2022.2117105. [ Links ]
5. Beloshapka A, Buff PP, Fahey Jr GC, Swanson KS. Compositional analysis of whole grains, processed grains, grain co-products, and other carbohydrate sources with applicability to pet animal nutrition. Foods 2016;5(2):23. doi:10.3390/foods5020023. [ Links ]
6. Castaldo L, Graziani G, Gaspari A, Izzo L, Tolosa J, Rodríguez Y, Ritieni A. Target analysis and retrospective screening of multiple mycotoxins in pet food using UHPLC-Q-Orbitrap HRMS Toxins 2019;11(8):434. doi:10.3390/toxins11080434. [ Links ]
7. Fuentes S, Carvajal M, Ruiz S, Martínez N, Gómez A, Rojo F. Presence of mutagens and carcinogens, called aflatoxins, and their hydroxylated metabolites in industrialized food for dogs. J Microb Biochem Technol 2018;10(3):76-86. doi:10.4172/1948-5948.1000399. [ Links ]
8. Shao M, Li L, Gu Z, Yao M, Xu D, Fan W. Mycotoxins in commercial dry pet food in China. Food Addit Contamb 2018;11(4):237-245. doi:10.1080/19393210.2018.1475425. [ Links ]
9. Signorini M, Gaggiotti M, Molineri A, Chiericatti C, deBasilico M, Basilico J, Pisani M. Exposure assessment of mycotoxins in cow’s milk in Argentina. Food Chem. Toxicol 2012;50(2):250-257. doi.org/10.1016/j.fct.2011.09.036. [ Links ]
10. Ott L, Appleton H, Shi H, Keener K, Mellata M. High voltage atmospheric cold plasma treatment inactivates Aspergillus flavus spores and deoxynivalenol toxin. Food Microbiol 2021;95:103669. doi:10.1016/j.fm.2020.103669. [ Links ]
11. Alshannaq A, Yu J. Occurrence, toxicity, and analysis of major mycotoxins in food. Int J Environ Res Pub Health 2017;14(6):632. doi:10.3390/ijerph14060632. [ Links ]
12. Witaszak N, Stępień Ł, Bocianowski J, Wáskiewicz A. Fusarium species and mycotoxins contaminating veterinary diets for dogs and cats. Microorganisms 2019;7(1):26. doi:10.3390/microorganisms7010026. [ Links ]
13. Hołda K, Wiczuk W, Hać-szymańczuk E, Głogowski, R. Comprehensive microbiological evaluation of dry foods for growing dogs marketed in Poland. Anim Sci 2017;56(1):81-89. doi:10.22630/AAS.2017.56.1.10. [ Links ]
14. Singh SD, Chuturgoon AA. A comparative analysis of mycotoxin contamination of supermarket and premium brand pelleted dog food in Durban, South Africa. J S Afr Vet Assoc 2017;88(1):1-6. doi:10.4102/jsava.v88i0.1488. [ Links ]
15. Hernandez-Valdivia E, Valdivia-Flores AG, Cruz-Vazquez C, Martínez-Saldaña MC, Quezada-Tristan T, Rangel-Muñoz EJ, Jaramillo-Juarez F. Diagnosis of subclinical aflatoxicosis by biochemical changes in dairy cows under field conditions. Pak Vet J 2021;41(1):33-38. [ Links ]
16. Dereszynski D, Center S, Randolph J, Brooks M, Hadden A, Palyada, K, et al. Clinical and clinicopathologic features of dogs that consumed foodborne hepatotoxic aflatoxins: 72 cases (2005-2006). JAVMA J Am Vet Med Assoc 2008;232(9):1329-1337. doi:10.2460/javma.232.9.1329. [ Links ]
17. FAO/ WHO (Food and Agriculture Organization of the United Nations- World Health Organization). General standard for contaminants and toxins in food and feed. Codex Alimentarius 2019. Codex stan 193-1995. https://www.fao.org/fao-who-codexalimentarius/sh-proxy/es/?lnk=1&url=https%253A%252F%252Fworkspace.fao.org%252Fsites%252Fcodex%252FStandards%252FCXS%2B193-1995%252FCXS_193e.pdf. [ Links ]
18. Commission Directive 2003/100/EC of 31 October 2003 amending Annex I to Directive 2002/32/EC of the European Parliament and of the Council on undesirable substances in animal feed. Off J Eur Union 2003; 285:33-37. https://www.legislation.gov.uk/eudr/2003/100/adopted. [ Links ]
19. Reis-Gomes A, Marcolongo-Pereira C, Sallis E, Pereira D, Schild A, Faria R, Meireles M. Aflatoxicosis in dogs in Southern Rio Grande do Sul. Pesq Vet Bras 2014;34(2):162-166. doi:10.1590/S0100-736X2014000200011. [ Links ]
20. Arnot L, Duncan N, Coetzer H, Botha C. An outbreak of canine aflatoxicosis in gauteng province, South Africa. J S Afr Vet Assoc 2012;83(1):1-4. doi:10.4102/jsava.v83i1.2. [ Links ]
21. Bruchim Y, Segev G, Sela U, Bdolah T, Salomon A, Aroch I. Accidental fatal aflatoxicosis due to contaminated commercial diet in 50 dogs. Res Vet Sci 2012;93(1):279-287. doi:10.1016/j.rvsc.2011.07.024. [ Links ]
22. Álvarez-Días MF, Torres-Parga B, Valdivia-Flores AG, Quezada-Tristán T, Alejos-De La Fuente JI, Sosa-Ramírez J, Rangel-Muñoz EJ. Aspergillus flavus and total aflatoxins occurrence in dairy feed and aflatoxin M1 in bovine milk in Aguascalientes, México. Toxins 2022;14(5):292. doi:10.3390/toxins14050292. [ Links ]
23. Rangel-Muñoz EJ, Valdivia-Flores AG, Moreno-Rico O, Hernández-Delgado S, Cruz-Vázquez C, De Luna-López MC, et al. Characterization of Aspergillus flavus and quantification of aflatoxins in feed and raw milk of cows in Aguascalientes, Mexico. Rev Mex Cienc Pecu 2020;11(2):435-454. doi:10.22319/rmcp.v11i2.5686. [ Links ]
24. INEGI (Instituto Nacional de Estadística, Geografía e Informática). Anuario estadístico y geográfico de Aguascalientes. INEGI, Aguascalientes, 2017. https://www.inegi.org.mx/contenido/productos/prod_serv/contenidos/espanol/bvinegi/productos/nueva_estruc/anuarios_2017/702825092078.pdf. [ Links ]
25. Segura-Correa JC, Honhold N. Métodos de muestreo para la producción y salud animal. Universidad Autónoma de Yucatán. Mérida, Yucatán, México. 2000. https://www.researchgate.net/publication/273945817_Métodos_de_muestreo_para_la_produccion_y_la_salud_animal. [ Links ]
26. Espinoza P, López R, Lozano S. Muestreo de bola de nieve. En Espinoza P, López R, Lozano S, Técnicas de muestreo. México: UNAM 2018:(2-4). https://www.studocu.com/pe/document/universidad-cesar-vallejo/sistemica/pdf-proyectofinal-bola-de-nieve-compress/28006947. [ Links ]
27. Santibáñez Escobar R, Martínez Ibarra JA, Tapia González JM, Avellaneda Cevallos JH, Hernández Gallardo M, Montañez Valdez OD. Identificación y cuantificación de hongos micotoxigénicos en alimento para bovinos. Cienc Tecnol 2011;4(1):19-23. doi:10.18779/cyt.v4i1.96. [ Links ]
28. Leck, A. Preparation of lactophenol cotton blue slide mounts. Community Eye Health 1999;12(30):24. https://www.cehjournal.org/article/preparation-of-lactophenol-cotton-blue-slide-mounts/. [ Links ]
29. Jayawardena RS, Hyde KD, de Farias AR, Bhunjun CS, Ferdinandez HS, Manamgoda D, Udayanga D, et al. What is a species in fungal plant pathogens? Fungal Divers 2021; 109(1):239-266. doi.org/10.1007/s13225-021-00484-8. [ Links ]
30. Klich MA. Identification of common Aspergillus species. First ed. Lousiana, USA: Centraalbureau voor Schimmelcultures, Utrecht, the Netherlands; 2002. [ Links ]
31. Fani SR, Moradi M, Probst C, Zamanizadeh H, Mirabolfathy M, Haidukowski M, Logrieco A. A critical evaluation of cultural methods for the identification of atoxigenic Aspergillus flavus isolates for aflatoxin mitigation in pistachio Orchards of Iran. Eur J Plant Pathol 2014;140:631-642. doi:10.1007/s10658-014-0499-1. [ Links ]
32. Shapira R, Paster N, Eyal O, Menasherov M, Mett A, Salomon R. Detection of aflatoxigenic molds in grains by PCR. Appl Environ Microbiol 1996;62(9):3270-3273. doi:10.1128/aem.62.9.3270-3273.1996. [ Links ]
33. White TJ, Bruns T, Lee S, Taylor J. Amplification and direct sequencing of fungal ribosomal RNA genes for phylogenetics. In PCR Protocols; Innis MA, et al, editors. Academic Press, Inc.: New York, NY, USA, 1990:315-322. doi:10.1016/B978-0-12-372180-8.50042-1. [ Links ]
34. Scott PM. Mycotoxin methodology. Food Addit Contam 1995;12(3):395-403. doi:10.1080/02652039509374321. [ Links ]
35. FAO (Food and Agriculture Organization). Worldwide Regulations for mycotoxins in food and feed 2003. FAO Food Nutr paper 81 2003:59. https://www.fao.org/3/y5499e/y5499e00.htm. [ Links ]
36. Case L, Carey D, Hirakawa D, Daristotle L. Feeding management throughout the life cycle. In Case L, Carey D, Hirakawa D, Daristotle L. Canine and feline nutrition. 3rd ed. USA: Mosby. 2011:191-294. doi.org/10.1016/C2009-0-39175-8. [ Links ]
37. Rodríguez M, Ramos A, Prim M, Sanchis V, Marín S. Usefulness of the analytical control of aflatoxins in feedstuffs for dairy cows for the prevention of aflatoxin M1 in milk. Mycotoxin Res 2019;36(1):11-22. doi:10.1007/s12550-019-00362-y. [ Links ]
38. Tegzes J, Oakley B, Brennan G. Comparison of mycotoxin concentrations in grain versus grain-free dry and wet commercial. Toxicol- Commun 2019;3(1):61-66 doi:10.1080/24734306.2019.1648636. [ Links ]
39. Błajet-Kosicka A, Kosicki R, Twarużek M, Grajewski J. Determination of moulds and mycotoxins in dry dog and cat food using liquid chromatography with mass spectrometry and fluorescence detection. Food Addit Contam B Part 2014;7(4):302-308. doi:10.1080/19393210.2014.933269. [ Links ]
40. GMP (Good Manufacturing Practices + International B.V.). Specific Feed Safety Limits. GMP+ International. The Netherlands, 2021: 1-78. https://www.gmpplus.org/media/zuwj0gam/ts-1-5-specific-feed-safety-limits.pdf. [ Links ]
41. Samson RA, Visagie CM, Houbraken J, Hubka V, Perrone G, Seifert KA, et al. Phylogeny, identification and nomenclature of the genus Aspergillus. Stud Mycol 2014,78:141-173. doi:10.1016/j.simyco.2014.07.004. [ Links ]
42. Okayo RO, Andika DO, Dida MM, K’Otuto GO, Gichimu BM. Morphological and molecular characterization of toxigenic Aspergillus flavus from groundnut kernels in Kenya. Int J Microbiol 2020;(2020):8854718. doi:10.1155/2020/8854718. [ Links ]
43. Medina A, Akbar A, Baazeem A, Rodríguez A, Magan N. Climate change, food security and mycotoxins: Dowe know enough?. Fungal Biol Rev 2017;31(3):143-154. doi:10.1016/j.fbr.2017.04.002. [ Links ]
44. Bates N. Aflatoxicosis in dogs. Companion Animal 2021;26(8):197-202. doi.org/10.12968/coan.2021.0034. [ Links ]
45. Chiewchan N, Mujumdar A, Devahastin S. Application of drying technology to control aflatoxins in foods and feeds: A Review Dry Technol 2015;33(14):1700-1707. doi:10.1080/07373937.2015.1068795. [ Links ]
46. Gallo A, Solfrizzo M, Epifani F, Panzarini G, Perrone, G. Effect of temperature and water activity on gene expression and aflatoxin biosynthesis in Aspergillus flavus on almond medium. Int J Food Microbiol 2015;217:162-169. doi.org/10.1016/j.ijfoodmicro.2015.10.026. [ Links ]
47. Coppock RW, Christian RG, Jacobsen BJ. Aflatoxins. In Gupta RC. Veterinary toxicology. Basic and clinical principles. 3rd ed. Hopkinsville, Kentucky, USA: Elsevier Inc. 2018:983-994. doi:10.1016/B978-0-12-811410-0.00069-6. [ Links ]
48. Macías A, Rial C, Acosta A, Henríquez L, Almeida M, Rodríguez Á, et al. Risk assessment of the exposure to mycotoxins in dogs and cats through the consumption of commercial dry food. Sci Total Environ 2020;708:134592. doi:10.1016/j.scitotenv.2019.134592. [ Links ]
49. Dillon G, Cardinall C, Keegan J, Yiannikouris A, Brandl W, Moran C. The analysis of docosahexaenoic acid (DHA) in dried dog food enriched with an Aurantiochytrium limacinum biomass: Matrix extension validation and verification of AOAC method 996.06. J AOAC Int 2021;104(1):68-77. doi:10.1093/jaoacint/qsaa097. [ Links ]
50. Wan J, Chen B, Rao J. Occurrence and preventive strategies to control mycotoxins in cereal-based food. Compr Rev Food Sci 2020;19(3):928-953. doi:10.1111/1541-4337.12546. [ Links ]
51. Alshawabkeh K, Alkhalaileh NI, Abdelqader A, Al-Fataftah AR, Herzallah SM. Occurrence of aflatoxin B1 in poultry feed and feed ingredients in Jordan using ELISA and HPLC. Am-Eurasian J Toxicol Sci 2015;7(4):316-20. doi:10.5829/idosi.aejts.2015.7.4.10172. [ Links ]
52. Shad Z, Ghavami M, Atungulu GG. Occurrence of aflatoxin in dairy cow feed ingredients and total mixed ration. Appl Eng Agric 2019;35(5):679-686. doi:10.13031/aea.13454. [ Links ]
53. Atungulu G, Mohammadi-Shad Z, Wilson S. Mycotoxin issues in pet food. In Ricke S, et al., editors. Food and feed safety systems and analysis. 1rst ed. Academic Press 2018:25-44. doi:10.1016/B978-0-12-811835-1.00002-6. [ Links ]
54. Di Gregorio M, de Neeff D, Jager A, Corassin C, de Pinho A, de Albuquerque R, et al. Mineral adsorbents for prevention of mycotoxins in animal feeds. Toxin Rev 2014;33(3):125-135. doi:10.3109/15569543.2014.905604. [ Links ]
55. Tomašević-Čanović M, Daković A, Rottinghaus G, Matijaševic´ S, Đuričić M. Surfactant modified zeolites-new efficient adsorbents for mycotoxins. Microporous Mat 2003;61(1-3):173-180. doi:10.1016/S1387-1811(03)00365-2. [ Links ]
Received: January 17, 2023; Accepted: June 12, 2023