Services on Demand
Journal
Article
Indicators
-
Cited by SciELO
-
Access statistics
Related links
-
Similars in SciELO
Share
Revista mexicana de ciencias pecuarias
On-line version ISSN 2448-6698Print version ISSN 2007-1124
Rev. mex. de cienc. pecuarias vol.13 n.2 Mérida Apr./Jun. 2022 Epub June 20, 2022
https://doi.org/10.22319/rmcp.v13i2.5832
Review
Heat stress in dairy cattle with emphasis on milk production and feed and water intake habits. Review
a Universidad Autónoma de Baja California. Instituto de Ciencias Agrícolas, 21751, Valle de Mexicali, B.C. México.
The negative impact of heat stress (HS) in dairy cattle results in considerable economic losses at world level, as it reduces the milk production, reproductive efficiency, and productive life in the cows. In addition, the continuous genetic improvement results in highly productive cows, which are, however, less tolerant to HS because they produce greater metabolic heat. This, together with global warming, will turn HS into a hard-to-control challenge for the daily industry. In response dependent on the degree of HS, the dairy cattle carry out a series of physiological, metabolic and behavioral adjustments as thermoregulatory mechanisms for removing excess body heat and reducing the endogenous production of body heat, in order to maintain the normothermia. However, fertility and milk secretion decrease as a direct effect of hyperthermia and an indirect effect of lower dietary nutrient intake. Food and water intake are closely associated to the reduction of the productivity of dairy cattle exposed to HS. Notably, the impact of HS on productivity of dairy cattle varies among breeds, among which Bos taurus, particularly the Holstein breed, are less tolerant to HS. The identification of genes associated to thermotolerance utilized in selection programs using genetic markers to breed high milk-producing cows in warm climates is currently being studied. Therefore, the purpose of this review is to carry out a comprehensive analysis of the effects of HS on milk production, activation of thermoregulatory mechanisms and feed intake behavior in dairy cattle.
Keywords Holstein cattle; Thermoregulation; Hyperthermia; Climate change; Feed intake behavior
El impacto negativo del estrés por calor (EC) en la ganadería lechera repercute en cuantiosas pérdidas económicas a nivel mundial, dado que reduce la producción de leche, la eficiencia reproductiva y la vida productiva de las vacas. Adicionalmente, el mejoramiento genético continuo resulta en vacas muy productivas, pero menos tolerantes al EC debido a que producen mayor calor metabólico. Esto en conjunto con el calentamiento global convertirá al EC en un reto difícil de controlar para la industria lechera. Como respuesta dependiente del grado de EC, el ganado lechero realiza una serie de ajustes fisiológicos, metabólicos y conductuales como mecanismos de termorregulación para disipar el exceso de calor corporal y reducir la producción endógena del mismo, todo dirigido a mantener la normotermia. Sin embargo, la secreción láctea y la fertilidad se reducen por efecto directo de la hipertermia e indirectamente por la reducción en el consumo de nutrientes dietéticos. Los consumos de alimento y de agua están asociados estrechamente con la reducción de la productividad en ganado lechero expuesto a EC. Cabe mencionar que el impacto del EC en la productividad del ganado lechero varía entre razas, siendo las razas Bos taurus menos tolerantes al EC, particularmente la raza Holstein. Actualmente, se investiga en la identificación de genes asociados con la termotolerancia, los cuales son empleados en programas de selección por marcadores genéticos para producir vacas altas productoras de leche en climas cálidos. Por lo tanto, el objetivo de esta revisión es hacer un análisis comprensivo de los efectos del EC sobre la producción de leche, activación de mecanismos de termorregulación y conducta de ingesta en ganado lechero.
Palabras clave Ganado Holstein; Termorregulación; Hipertermia; Cambio climático; Conducta de ingesta
Introduction
Heat stress (HS) is defined as the sum of external environmental forces that act on the animal causing an increase in body temperature, and the activation of physiological and behavioral adjustments in the first place1. In dairy cattle, these adjustments represent adaptation mechanisms activated to attempt of maintaining a balanced body homeostasis2. As global warming continues to augment, the prevalence of HS in dairy cattle will also increase in frequency, duration, and severity3,4. Therefore, mitigating the negative effects of HS on the productivity of dairy herds has become a challenge for the dairy industry worldwide5.
The HS problem is higher in warm geographical areas where it can start during the late spring and extend beyond the summer, and consequently low fertility and milk production in cows can be prolonged until the fall2,6. The magnitude of the HS is determined by the combined effects among ambient temperature (AT), relative humidity (RH), solar radiation, and wind speed1,2. In the particular case of dairy cattle, the temperature-humidity index (THI) is estimated based on AT and RH, first, in order to determine the degree of HS to which the animals are exposed and, secondly, for the purpose of making herd management decisions during the hot season1,7. Bouraoui et al5 reported that milk production diminished by 21 % when the THI increased from 68 to 78 units, or when the AT exceeded 27 °C, regardless of the age or lactation stage.
The negative impact of HS on fertility and milk production has given rise to much research in the last 50 years, and today a deep knowledge of the physiological, metabolic, endocrine, and molecular mechanisms has been attained, leading to a reduction of the productivity of the cattle resulting from HS. However, several of these mechanisms are triggered by two key factors: the reduction in feed intake and the increase in water intake8. In fact, milk production is closely related to the dry matter (DM) and water intake in milk producing cows subjected to chronic HS9. The first physiological and intake behavior adjustments in dairy cattle are considered to be homeostatic mechanisms8, whose main purpose is to increase evaporative heat losses (i.e., breath and sweat) and to reduce the production of endogenous heat in order to maintain normothermia10-12. It should be noted that the genotype plays an important role in defining the impact of HS on the dairy cattle production (13,14, among which Holstein is one of the most susceptible breeds, while the pure native cattle of warm regions, or their cross with introduced breeds, are more tolerant15-18. Thus, the objective of the present review is to carry out a comprehensive analysis of the effects of HS on milk production, activation of thermoregulatory mechanisms, and intake behavior of dairy cattle.
Climate change and the future of dairy production
The production of greenhouse gases such as steam, carbon dioxide, methane, and nitrous oxide, among others, alter the permeability of the atmosphere, letting the sun rays through while preventing the release of the heat radiated by the Earth’s surface19. Thus, these gases cause global warming and, therefore, climate change, which tends to increase the amount of land surface with warm climate and, in extreme cases, desertification20. Studies by climatologists suggest that the AT may increase by more than 2 °C by the year 205019.
Today, the profitability of the dairy industry is endangered by the climate change, which has reduced the availability of natural resources, pasturelands, and production of grains and fodder, while it has favored the cattle losses due to natural disasters, as well as the prevalence of parasites and unforeseen diseases2,21. The increase of agroecological areas with high AT as a result of climate change has also decreased milk production and increased production costs8,22. In fact, HS is expected to increase in severity, duration, and presence in the near future21. In the United States of America, economic losses in the dairy industry due to HS were estimated in 897 million dollars22, and they recently projected economic losses of 0.4 and 1.2 % by 2050 and 2100, respectively, in the dairy basin in the northeast of that country21. In Mexico, there are no reports of losses generated by HS in dairy cattle; however, such losses are evident, based on the seasonality of the milk production of dairy basins in warm weather6,23. It is worth mentioning that the world food safety is jeopardized by climate change, considering that the demand of meat and milk will increase by 73 and 58 %, respectively, by 2050 in relation to the 2010 production levels24.
Thermoregulation and metabolic heat production in dairy cattle
Bovines, like any other farm animal, are homeothermal and, therefore, have the ability to maintain a relatively stable internal body temperature regardless of the environmental conditions of their surroundings25. In absence of thermal insults or in presence of fever, the body temperature of the dairy cattle ranges between 38.0 and 38.5 °C and is the combined reflex of the balance between environmental heat gains and metabolic heat production in a homeostasis state1. In fact, in a thermoneutral environment and without milk production, cows can maintain their normothermia because they use mainly non-evaporative means (conduction, convection, and radiation), which do not entail an increase in the energetic cost associated to thermoregulation26. If the rectal temperature (RT) is ≥42 °C, the body homeostasis balance can be disrupted, resulting in the death of the animal22.
It should be noted that the body temperature is not stable and exhibits a circadian rhythm which varies by approximately 1°C between maximum and minimum temperatures registered through the day1. The body temperature reaches its maximum peak between 8 and 10 h after the AT has reached its maximum level; however, dairy cows can adapt to changes in AT and RH throughout the year27,28. This is because its thermoneutral zone is very broad, ranging between -0.5 and 20.0 °C1,2,10. According to certain studies, the maximum critical AT at which the Holstein cattle can maintain a stable body temperature in the range between 24 and 27° C29.
On the other hand, the milk synthesis in mammary gland of cows demands a great amount of metabolic activity, and therefore, the endogenous heat production increases in proportion to the level of milk production25,27. It should be noted that the genetic improvement of dairy cattle has focused on increasing the milk production parameters, which has turn cows into metabolic heat producing machines, making them highly susceptible to warm climates17,30,31. Cows that produce 18 to 31 L/day generate 28 to 48 % more metabolic heat than dry cows32. A cow weighing 700 kg and producing 60 kg/d of milk generates approximately 44,171 kcal of heat per day; the same cow produces 25,782 kcal of heat per day at the end of the lactation period (20 kg/d)27. Dairy cows that convert feed into milk more efficiently produce less metabolic heat and have a lower skin temperature33. Thus, cows with a high milk production will have to make a greater effort in order to regulate their internal temperature under thermoneutral conditions, while under AT conditions they will be less tolerant to HS.
Physiological thermoregulation of dairy cattle under heat stress
High AT´s combined with a high percentage of RH further limit the ability of dairy cows to dissipate heat, and, in consequence, they experience HS and the activation of physiological thermoregulation mechanisms for dispelling the heat load2,5,26. Certain climate indexes have been developed in order to predict the level of HS in dairy cattle; however, the THI proposed by Hahn34 is the most commonly used worldwide. This index combines the climate values of AT and RH into an equation (THI = [0.81 × AT] + [RH / 100] * [AT - 14.4] + 46.4), and dairy cows are considered to begin to suffer from HS when they exhibit 72 units1. Armstrong35 classified HS into three types according to the THI: mild (72 to 78 units), moderate (79 to 89 units), and severe (90 to 98 units) (Figure 1).
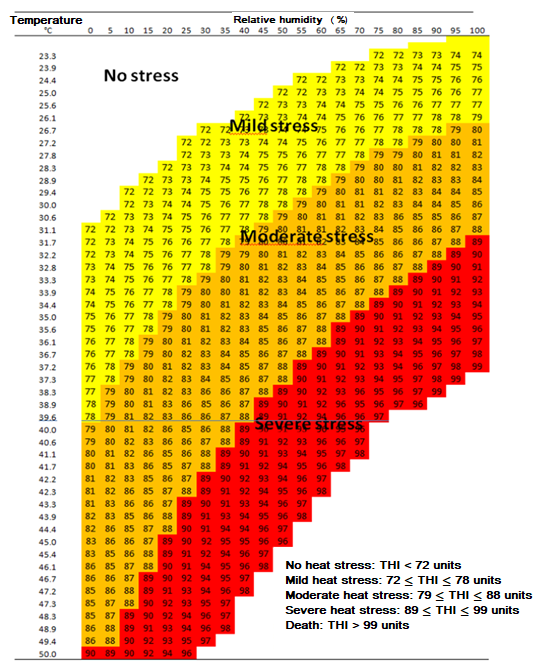
Figure 1 Classification of heat stress according to the temperature-humidity index (THI) in dairy cattle (based on Armstrong, 1994).
However, recent studies11,36 have reported that a THI of 68 is already a critical value at which dairy cows will begin to exhibit symptoms of HS. Another study37 found HS symptoms in highly producing dairy cows when the daily average THI was above 68 units or when the minimum THI was above 65 units. The climate in which the dairy cattle develop also plays an important role in defining the onset of HS based on the THI, given that under tropical conditions the negative effects of HS began at a THI > 70 units1, and at a THI >60 under temperate conditions9. This suggests that the pressure caused by genetic selection to improve dairy production counters tolerance to HS in dairy cattle17.
An imminent response to presence of HS in dairy cattle is an increase in the RT due to excessive body heat accumulation from the environment. This activates body heat losses through both evaporative and non-evaporative means6,10,26. However, the activation sequence of physiological thermoregulation mechanisms will depend upon the HS type (acute or chronic) and degree (slight, moderate, or severe)11. A more severe or more chronic HS will determine the reduction of body heat loss through non-evaporative means, while evaporative heat losses due to sweat and panting will increase considerably8. Maia et al26 observed 85 % body heat losses due to skin evaporation, and only 15 % through the respiratory tract in Holstein cows exposed to AT >30 ºC.
A high RH reduces the effectiveness of body heat losses through evaporative means10. The results obtained by Bonmanova et al7 showed that RH was the limiting factor for HS in humid climates, while in dry climates the limiting factor was AT. Furthermore, the activation of these evaporative thermoregulatory mechanisms entails additional energy expenditure unlike with non-evaporative mechanisms, whereby there is a greater energetic expenditure for maintenance in heat-stressed dairy cows. An increased respiratory frequency (RF) requires an additional energy expenditure of 7 to 25 % due to movement of the muscles of the respiratory tract38, and the water vaporization process through the skin and respiratory tract demands an energy expenditure of 2.43 J/ml of evaporated water1. For this reason, the activation of heat losses through evaporative means is an option for the animal when non-evaporative losses are unable to release the body heat load under HS. In this sense, the RF increases with a THI above 73 units, while the RT increases with a THI >80 units39.
On the other hand, diurnal variations in climatic conditions and the changes in animal behavior improve the physiological thermoregulatory capacity of heat-stressed dairy cattle. An AT ≤21°C during the nighttime, with a duration of 3 to 6 h, favors the total body heat loss accumulated in the daytime, as the animal promotes a redistribution of blood flow toward the skin, allowing an efficient release of the body heat load throughout non-evaporative means (radiation and convection)40. A wind speed between 1.8 and 2.8 m/s also promotes body heat losses through the skin28. Likewise, cows adopt a standing position in order to reduce the body area exposed to sun rays and the heat gain from contact with the ground41. When under HS, they also reduce their feed intake per day and eat at those times of the day when AT is lowest8. This allows their daily water intake to increase42.
Heat stress and feed intake
The reduction in milk production by heat stressed dairy cattle has traditionally been associated to a reduction in feed intake8, and the degree at which it diminishes depends on the duration and severity of the prevalent HS, as well as on the adaptation level of the breed14,18,25. Thus, the AT has an inverse relationship with DM intake under chronic HS, while, under acute HS, feedd intake is rapidly reduced one day after the exposure43. In fact, feed intake begins to decline at an AT of 26 °C in lactating cows1, and its reduction can reach up to 40 % at an AT≥40 °C44.
Given that the presence of HS is determined based on the estimation of THI in dairy cattle and that this climatic parameter is easier to obtain than a daily measurement of feed intake, certain researchers opted to establish the association between them, and results showed a reduction of 0.51 kg in DM intake per unit which increases THI within the range of 72 to 84 units8. Another study found that the variation in minimum THI has a greater association with the changes in feed intake than the variation in maximum THI45.
Besides the reduction in feed intake, heat stressed cows also modify their feed intake schedules in order to reduce their endogenous heat production during the hottest hours of the day. Metabolic heat associated to ruminal fermentation represents 3 to 8 % of the total endogenous heat produced by bovines, depending on the dietary fiber level12. Dairy cows have a daily natural feed consumption pattern, being high after receiving fresh diet, as well as in the afternoon and evening46,47. However, dairy cattle under HS prefer to eat very early in the morning and at nightfall, as digestion and metabolic heat production reach their peak 3 to 4 hours after feed intake, just before the hottest period of the day32. It is worth noting that HS in dairy cattle also impacts the ruminal kinetic of the ingested feed, as it decreases the motility of the gastrointestinal tract, changes the patterns of fermentation and production of volatile fatty acids, and increases the retention time of feed in rumen and its digestibility38,47.
In order to mitigate the reduction of DM intake and sustain milk production during the warm season of the year, certain cattle breeders modify the dietary chemical composition to increase the energy level, which involves increasing the content of grains and reducing the amount of dietary fiber12,39. This nutritional strategy benefits the cattle by allowing them to meet their nutritional requirements with a lower feed intake. However, the modification in the dietary ingredients can have negative consequences if too many adjustments are made; for instance, a high blood urea concentration due to a high protein digestion in rumen increases the RT48, or a high concentrate diet can contribute to potentiate the acidosis issue generated by HS in cows12.
Heat stress and water intake
An insufficient availability of drinking water has adverse effects on the productivity and welfare of dairy cattle under any environmental conditions, but it becomes more critical at high AT42. Regardless of the climate, lactating cows require larger amounts of water, as both the body and the milk are composed of more than 90 % water38,49. Nevertheless, these cows can vary their water intake according to the amount of DM consumed in the diet and daily kilograms of milk produced50. A restriction in water intake can reduce milk production by up to 26%, and this reduction can be even greater in HS conditions51.
Dairy cows satisfy their water requirements from three sources: direct water intake, water from foods, and water derived from the tissue oxidative metabolism42. However, the main source of water for cattle is direct intake under any climate condition (83 %)49. Water intake is affected by such factors as: DM intake, milk production, and intake of potassium, sodium, and nitrogen52. Cows under thermoneutral conditions can consume 14 to 171 kg/d of water, with a daily average of 82 kg, and, based on the milk production and dietary sodium intake, water intake is estimated to be 4 to 4.5 L/kg of milk produced42. On the other hand, a water intake of 146 g per gram of ingested sodium52 was found in absence of a thermal insult. In dry cows without heat stress, water intake is significantly lower (15 to 61 L/d)53, but increases by 27 % with the onset of milk production during the early lactation54.
Dairy cows can experience severe dehydration when they lose 12 % of their body weight due to lack of water intake; such dehydration can be lethal if the total body water loss reaches approximately 20 %55. Given that HS increases water loss in ruminants considerably, its intake at adequate levels becomes critical for dairy cows54. In warm areas, water intake can increase between 10 and 20 % during the summer, when even a cow classified as low producing can drink more than 100 L/d32. A recent study reported that the water intake can increase by up to 50 % as the THI approaches 80 units 56. A cow is estimated to increase its water intake by 1.52 kg for each Celsius degree that the AT increases42. Furthermore, the time devoted every day to water intake increases from 0.26 to 0.5 h as the THI changes from 56.2 to 73.8 units57 or the AT increases from 15 to 33 °C58. The greater water intake under HS conditions is attributed to an increase in the urine volume (25 %), evaporation through the respiratory tract (panting), and sweating1, which are thermoregulatory mechanisms that help dissipate excess body heat load in this type of environments59.
On the other hand, as previously stated, water intakes in dairy cattle are very high under thermoneutral conditions and even higher under HS, which leads this species to have different water intake behaviors compared to other species. Under thermoneutral conditions, Holstein cows with a medium milk production drink water approximately eight times a day, consuming an average of 12.9 L on each occasion60, while high producing Holstein cows drink water more frequently (12 to 16 times) but consume a smaller amount (5.4 to 6.0 L) on each occasion61. In the absence of HS, these cows prefer to drink 97 % of their total daily intake during the daytime, rather than at night60. Conversely, when under HS, the water intake behavior among these animals becomes highly variable at AT above 30 °C62. This may be due to individual differences in tolerance to heat and, therefore, in milk production. In general, heat stressed cows resort to the water trough more frequently than unstressed cows, but they prefer to drink water at times when solar radiation is at its lowest or absent63. The reason for this low water intake behavior during the daytime, particularly during the hottest hours of the day (on three to five occasions), is that cows prefer to remain motionless, rather than gaining heat by seeking this nutrient. Therefore, the construction design of pens or grazing areas is crucial for the cow’s decision to taken a drink of water under hot weather.
The water troughs located at a large distance from the feed troughs, particularly when these have no shade, causes that heat stressed cows have to choose whether to move toward the shaded area or toward the water trough after eating56. So, it is recommended to strategically distribute water troughs both in pasturelands and pens in order to prevent cows having to walk more than 250 m32; likewise, these animals prefer high and long water troughs64. The cleanliness and temperature of the water are factors that largely determine intake in sufficient amounts by cows exposed to high AT. Like any other species, dairy cattle avoids drinking foul-smelling water, being necessary to continually wash the water troughs under any climate60. Cows also prefer to drink their warm water (≥24 ºC), rather than cool (15 a 20 ºC) or cold (≤10 ºC), under both thermoneutral65 and HS66 conditions. It is noteworthy that this preference of heat stressed cows to drink warm rather than cold water is contradictory, as it has been proven that the cold or cool water intake reduces both RT and RF67. Given the importance of this nutrient, it is essential to measure the water intake of dairy cattle, whether heat stressed or not, in order to maintain an optimal milk production and the good health of cows42,50.
Impact of heat stress on milk production
Dairy cows reach their optimal milk production at a temperature range of 5 to 25 °C, which is considered as the comfort zone for lactating cows1. Exposure of cows to ATs above the upper limit of this comfort zone may reduce milk production by 10 to 40 %38. However, the impact of HS on milk production depends on the stage of lactation, intensity of the heat, and cow’s genetic potential to synthesize milk. Thus, in presence of HS, high producing dairy cows experience a greater reduction in milk production than medium or low producing cows12,25. Medium-lactation dairy cows decrease their milk production by 35 % in response to HS, while early-lactation cows reduce it only by 14 %25. High-producing dairy cows tend to exhibit a larger bone structure and gastrointestinal tract capacity, which allows them to ingest and digest more food; however, metabolic heat production also increases and reduces their ability to maintain normothermia under HS conditions1. In fact, an increase in milk production from 35 to 45 kg/day reduces by 5 °C the AT at which HS begins68.
Milk production starts to diminish at an AT of 27 °C, regardless of the cow’s age or lactation stage1; this negative effect becomes evident 24 to 48 h after the onset of any type of HS43. A study carried out on Israeli Holstein cows of 3rd and 4th lactation found a reduction of 0.38 kg/cow/day in milk production for every Celsius degree that the AT increased during the summer69. As expected, there is a close negative association (r= - 0.76) between the THI and milk production5; the same applies to the AT. Therefore, this production parameter decreases by 0.41 kg/cow/day for every unit of THI above 6840. Nevertheless, there are studies reporting a lower reduction in milk production (0.2 kg/cow/d) per unit of THI above 7270. Variations in milk production level and AT may explain this discrepancy between studies.
Reduction in milk production occurs by direct and indirect effect of HS. Direct effects include adjustments in energy and protein metabolism in order to prioritize the availability of nutrients for the cows’ thermoregulation processes instead of sending them to the mammary gland for milk synthesis71. Likewise, HS increases the presence of oxidative stress, which alters the cellular metabolic and molecular activity in the mammary secretory tissue, reducing the cellular efficiency for synthesis of milk components30. Indirectly, the mechanism is associated with a reduction in DM intake, since maintenance energy requirements increase as a result of the activation of physiological thermoregulation mechanisms in a scenario where availability of body nutrients is limited due to low feed intake3,38. A reduction in the efficiency of energy use for milk production by 30 to 50 % has been reported for dairy cows in warm climates1.
The HS negatively affects not only milk production but also its composition56. A reduction in fat and protein content combined with an increase in somatic cell count (SCC) are results commonly associated to HS5. The results of a study contrasting spring thermoneutral conditions versus severe summer HS showed that fat (3.58 %) and protein (2.96 %) contents in milk decreased by 30 and 23 %, respectively, during the summer5. The same study detected an increase in SCC by 110 % due to summer HS. Another study reported a 40 % decrease in milk fat content of Holstein cows under moderate to severe HS, as well as a 17 % decrease in protein content1. Bernabucci et al72 observed a slightly lower casein concentration in milk during the summer compared to winter (2.18 vs 2.58 %). The reduction in the casein percentage may account the low cheese production during that season73.
Adaptive responses of dairy breeds to heat stress
Holstein cattle is the genotype par excellence for the global dairy industry; however, they have little resistance to HS. Genetic improvement programs for this breed have focused on selecting traits associated to increase milk quality and production, whose effects are contrary to their ability to tolerate high AT´s28. Thus, the heritability index for milk production in Holstein cows can diminish due to HS and, consequently, the selection response based on this trait may not be as expected in warm climates17. In spite of this, Holstein cattle continues to be used in warm regions because it maintains a larger average milk production than other genotypes adapted to the region, mainly in technified and semi-technified production systems13,18.
Although Holstein cattle has proven to maintain a higher milk production than such breeds as Simmental (20.4 vs 28.1 L)13 and Jersey (26.6 vs 34.2 L)16 under tropical HS conditions, their milk is characterized by having a high SCC and low fat and protein content. In addition, the reduction in milk production as a result of HS is more pronounced in Holstein cows; this is an unmistakable symptom of lower tolerance to high AT´s13,18. Therefore, the use of the Holstein breed in warm climates, particularly in tropical and subtropical regions, cannot be justified based on the milk production level, given that HS conditions reduce the quality of this product and compromise the health and welfare of cows.
The use of animal genetic resources of the region, including native and introduced cattle breeds that are highly adapted to the warm-humid climate31,74, is recommended for milk production in tropical climates. Some studies also suggest crossbreeding between the Holstein and zebu breeds in order to generate cattle with a dairy genotype tolerant to warm climates10,15. Thus, dairy cattle breeds adapted to HS exhibit greater tolerance to these environmental conditions because, phenotypically, they have thinner and less dense hair, as well as a higher density of sweat glands in the skin, which allows them to more easily release their body heat load by convection and radiation14,75. In addition, this type of cattle under HS relatively maintain their feed intake and thyroid gland activity, which explains the lower variation in milk production compared to non-adapted cattle75. The main breeds used in the Mexican tropics for milk production are Brown Swiss, Jersey, Holstein, Gyr, and Brahman, as well as their crosses; however, other management and feeding factors, along with the climate conditions, lead to a low productive and reproductive efficiency76.
On the other hand, the cells of HS-adapted cattle activate heat shock transcription factors to coordinate protective mechanisms in response to the thermal insult, including expression of genes, activation of heat shock proteins (HSP), and carbohydrate metabolism77. In HS tolerant bovines (Bos indicus) compared to HS intolerant cattle (Bos taurus), cells exhibit a greater HSP expression (mainly of 70 kDa78 and 90 kDa79) in response to HS, which improves their viability and immune activity80. However, it has been proven that dairy cattle mononuclear cells may exhibit an HSP-72 overexpression in response to hyperthermia, which is counter-productive for their immune response and tolerance to heat81. This became evident in a study in which mononuclear cells of Holstein and Brown Swiss cattle were cultivated in vitro at 39 (normal), 40, 41, 42, and 43 ºC, and results showed that hyperthermia conditions triggered excessive mRNA production for HSP-72 and low DNA synthesis in Brown Swiss cattle compared to Holstein81. Contrary to the predictions, these findings suggest that Holstein cattle resists better hyperthermia than Brown Swiss cattle, although the latter can maintain an adequate productive efficiency under HS as long as their thermoregulatory capacity is not compromised and the core temperature does not rise above 40 ºC81.
Genetic markers associated to thermotolerance in dairy cattle
A large number of studies have focused on identifying the gene expression associated to thermotolerance in HS-adapted dairy cattle, and this information is being utilized to establish marker-assisted genetic selection programs82. Likewise, work is being carried out to identify males expressing thermotolerance genes, which are then selected as sires in order to transmit these characteristics to their offspring28. Special attention is being given to the search for these genes in high-producing dairy cattle, given the interest of the dairy industry in having HS tolerant genotypes that will produce large volumes of high quality milk.
In Mexico, there is little research on the identification of genes linked to thermotolerance in dairy cattle. Hernández-Corderos et al83 identified seven genes (AVPR1A, Furin, IGFBP5, IGFBP6, PMCH, PRLR, and STAT5B) associated with milk production in heat-stressed Holstein cattle reared in the Yaqui Valley, Sonora; specifically, a single-nucleotide polymorphism (SNP) within each gene related to the prolactin and somatotropin pathway. Another study84 reported the identification of six genes associated with milk production and its fat and protein contents (SFXN1, LOC781028, ANKRD31, LOC100296562, LOC107131388, and WDR41) in a genome-wide association analysis conducted in Holstein cattle reared in the desert region of Mexicali, Baja California, Mexico. Further studies of Mexican Holstein cattle located in warm climate are required to identify other genes that may be directly associated with thermoregulatory traits, which, in combination with those already known to be related to milk production, may serve as genetic markers to recognize HS tolerant cattle.
In the United States, a recent study82 identified three genomic regions (BTA5, BTA14, and BTA15) which account a large part of the variation in milk production of heat-stressed Holstein cows. Ten genes were identified within these regions as associated to thermotolerance, given that they are involved in various cellular processes in response to HS, such as activation of HSP (PEX16, HSF1, EEF1D, and VPS28), reduction of oxidative stress, modulation of the apoptosis process (MAPK81P1, CREB3L1), DNA maintenance (TONSL), and thermotolerance (CRY2). Other genomic regions associated with the presence of thermotolerance genes in American Holstein cattle are BTA-2485 and BTA-2686, which are related to RT and milk production, respectively. Regarding the Chinese Holstein cattle, there is also evidence that they carry SNPs associated to thermotolerance genes such as ATP1A187, HSP90AA188, HSF1, HSP70A1A, and HSPB128.
Certain HS-thermotolerant genes have also been identified in other dairy cattle breeds. The SLICK haplotype gene has been identified in Senepol cattle and has been introduced into the Holstein breed with positive results for HS tolerance without compromising milk production89. The genes HSP90AB1 and HSPB8 have been found in Sahiwal dairy cattle, native breed to India90. It is worth noting that, seemingly, dairy breeds of the Mexican tropic have not been typified to detect genes associated with thermotolerance at high AT´s; this, therefore, remains a topic for future research.
Conclusions and implications
Heat stress is a key factor that conditions the milk production level in cattle, being more noticeable in those breeds that are sensitive to high AT´s. Dairy cattle start to experience HS when environmental conditions promote a THI of 72 units, although high-producing dairy breeds can begin to experience it at 68 units. Facing the HS environment, the cattle make physiological and metabolic adjustments to reduce endogenous heat production while releasing excess body heat load; however, the activation of thermoregulation mechanisms decreases the capacity of milk synthesis and secretion of cows. At least 40 % of the reduction in milk production is associated with low DM intake, and the rest, with direct effects on the general metabolism and cellular thermo-resistance. Today, the high pressure to select traits associated to milk production has resulted in cattle with a lower tolerance to HS; this genetic improvement strategy must therefore be modified by taking into account the presence of thermotolerant genes. In this sense, research on HS in dairy cattle must focus mainly on HS mitigation strategies that help reduce the energetic cost resulting from the activation of thermoregulatory mechanisms, as well as increase the daily feed intake.
Literatura citada
1. Kadzere CT, Murphy MR. Silanikove N, Maltz E. Heat stress in lactating dairy cows: a review. Livest Prod Sci 2002;77:59-91. [ Links ]
2. Herbut P, Angrecka S, Walczak J. Environmental parameters to assessing of heat stress in dairy cattle-a review. Int J Biometeorol 2018;62(12):2089-2097. [ Links ]
3. Min L, Zhao S, Tian H, Zhou X, Zhang Y, Li S, et al. Metabolic responses and “omics” technologies for elucidating the effects of heat stress in dairy cows. Int J Biometeorol 2017;61(6):1149-1158. [ Links ]
4. Theusme C, Avendaño-Reyes L, Macías-Cruz U, Correa-Calderón U, García-Cueto RO, Mellado M, Vargas-Villamil L, et al. Climate change vulnerability of confined livestock systems predicted using bioclimatic indexes in an arid region of México. Sci Total Environ 2021;751:141779. [ Links ]
5. Bouraoui R, Lahmar M, Majdou A, Djemali M, Belyea R. The relationship of temperature-humidity index with milk production of dairy cows in a mediterranean climate. Anim Res 2002;51:479-491. [ Links ]
6. Hernández-Rivera JA, Avendaño-Reyes L. Efecto de época del año (verano vs. invierno) en variables fisiológicas, producción de leche y capacidad antioxidante de vacas Holstein en una zona árida del noroeste de México. Arch Med Vet 2015;47(1):15-20. [ Links ]
7. Bohmanova J, Misztal I, Colef JB. Temperature-humidity indices as indicators of milk production losses due to heat stress. J Dairy Sci 2007;90:1947-1956. [ Links ]
8. Anzures-Olvera F, Macías-Cruz U, Álvarez-Valenzuela FD, Correa-Calderón A, Díaz-Molina R, West JW. Effects of heat-stress on production in dairy cattle. J Dairy Sci 2003;86:2131-2144. [ Links ]
9. Gorniak T, Meyer U, Südekum KH, Dänicke S. Impact of mild heat stress on dry matter intake, milk yield and milk composition in mid-lactation Holstein dairy cows in a temperate climate. Arch Anim Nutrit 2014;68:358-369. [ Links ]
10. Berman A. Are adaptations present to support dairy cattle productivity in warm climates? J Dairy Sci 2011;94:2147-2158. [ Links ]
11. Avendaño-Reyes L. Heat stress management for milk production in arid zones. In: Narongsak Chaiyabutr editor. Milk production - An up-to-date overview of animal nutrition, management and health. Intech Open, London UK; 2012:165-184. [ Links ]
12. Min L, Li D, Tong X, Nan X, Ding D, Xu B, et al. Nutritional strategies for alleviating the detrimental effects of heat stress in dairy cows: a review. Int J Biometeorol 2019;63:1283-1302. [ Links ]
13. Gantner V, Bobic T, Gantner R, Gregic M, Kuterovac K, Novakovic J, et al. Differences in response to heat stress due to production level and breed of dairy cows. Int J Biometeorol 2017;61:1675-1685. [ Links ]
14. Polsky L, von Keyserlingk MAG. Invited review: Effects of heat stress on dairy cattle welfare. J Dairy Sci 2017;100(11):8645-8657. [ Links ]
15. Pereira AM, Baccari F Jr, Titto EA, Almeida JA. Effect of thermal stress on physiological parameters, feed intake and plasma thyroid hormones concentration in Alentejana, Mertolenga, Frisian and Limousine cattle breeds. Int J Biometeorol 2008;52(3):199-208. [ Links ]
16. Smith DL, Smith T, Rude BJ, Ward SH. Short communication: comparison of the effects of heat stress on milk and component yields and somatic cell score in Holstein and Jersey cows. J Dairy Sci 2013;96(5):3028-33. [ Links ]
17. Santana ML, Bignardi AB, Pereira RJ, Stefani G, El Faro L. Genetics of heat tolerance for milk yield and quality in Holsteins. Animal 2017;11(1):4-14. [ Links ]
18. Avendaño-Reyes L, Correa-Calderón A, Macías-Cruz U, García-Casillas AC, Mellado M, Robinson PH, et al. Impacts on two dairy breeds of adding a third (night) cooling event under extreme ambient heat. Int J Biometeorol 2021;doi.org/10.1007/s00484-021-02115-y. [ Links ]
19. Al-Ghussain L. Global warming: Review on driving forces and mitigation. Environ Prog Sustain Energy 2019;38(1):13-21. [ Links ]
20. Arnell NW, Lowe JA, Challinor AJ, Osborn TJ. Global and regional impacts of climate change at different levels of global temperature increase. Clim Change 2019;155:377-391. [ Links ]
21. Hristov AN, Degaetano AT, Rotz CA, Hoberg E, Skinner RH, Felix T, et al. Climate change effects on livestock in the Northeast US and strategies for adaptation. Clim Change 2018;146:33-45. [ Links ]
22. St-Pierre NR, Cobanov B, Schnitkey G. Economic losses from heat stress by US livestock industries. J Dairy Sci 2003;86:E52-E77. [ Links ]
23. Fernández IG, Ulloa-Arvizu R, Fernández J. Milk yield did not decrease in large herds of high-producing Holstein cows in semi-arid climate of Mexico. Trop Anim Health Prod 2019;51(1):149-154. [ Links ]
24. FAO. World livestock 2011. Livestock in food security. A. McLeod (ed.). FAO. pp.115 Available at: http://www.fao.org/docrep/014/i2373e/i2373e00.htm [ Links ]
25. Bernabucci U, Lacetera N, Baumgard LH, Rhoads RP, Ronchi B, Nardone A. A metabolic and hormonal acclimation to heat stress in domesticated ruminants. Animal 2010;4:1167-1183. [ Links ]
26. Maia ASC, Da Silva RG, Battiston LCM. Sensible and latent heat loss from the body surface of Holstein cows in a tropical environment. Int J Biometeorol 2005; 50(1):17‐22. [ Links ]
27. Nardone A, Ronchi B, Lacetera N, Bernabucci U. Climatic effects on productive traits in livestock. Vet Res Commun 2006;30(suppl. 1):75-81. [ Links ]
28. Das R, Sailo L, Verma N, Bharti P, Saikia J, Imtiwati, Kumar R. Impact of heat stress on health and performance of dairy animals: A review. Vet World 2016 Mar;9(3):260-268. [ Links ]
29. Brouček J, Novák P, Vokřálová J, Šoch M, Kišac P, Uhrinčať M. Effect of high temperature on milk production of cows from freestall housing with natural ventilation. Slovak J Anim Sci 2009;42(4):167-173. [ Links ]
30. Gao ST, Ma L, Zhou Z, Zhou ZK, Baumgard H, Jiang D, et al. Heat stress negatively affects the transcriptome related to overall metabolism and milk protein synthesis in mammary tissue of midlactating dairy cows. Physiol Genomics; 2019;51:400-409. [ Links ]
31. Elayadeth‐Meethal M, Veettil AT, Maloney SK, Hawkins N, Misselbrook TH, Sejian V, et al. Size does matter: Parallel evolution of adaptive thermal tolerance and body size facilitates adaptation to climate change in domestic cattle. Ecol Evol 2018;8(21):10608-10620. [ Links ]
32. Ohnstad I. Managing heat stress in dairy cows. National animal disease information service 2016; (©NADIS). [ Links ]
33. DiGiacomo K, Marret LC, Wales WJ, Hayes BJ, Dunshea FR, Leury BJ. Thermoregulatory differences in lactating dairy cattle classed as efficient or inefficient based on residual feed intake. Anim Prod Sci 2014;54:1877-1881. [ Links ]
34. Hahn GL. Dynamic responses of cattle to thermal heat loads. J Anim Sci 1999;77(Suppl 2): 10-20. [ Links ]
35. Armstrong DV. Heat stress interaction with shade and cooling. J Dairy Sci 1994;77: 2044-2050. [ Links ]
36. De Rensis F, Garcia-Ispierto I, Lopez-Gatius F. Seasonal heat stress: Clinical implications and hormone treatments for the fertility of dairy cows. Theriogenology 2015;84:659-666. [ Links ]
37. Carter BH, Friend TH, Sawyer JA, Garey SM, Alexander MB, Carter MJ, et al. Effect of feed-bunk sprinklers on attendance at unshaded feed bunks in drylot dairies. Prof Anim Sci 2011;27:127-132. [ Links ]
38. NRC. National Research Coucil. Effect of environment on nutrient requirements of domestic animals. In: Basal metabolism. Washington, DC, USA: National Academy Press; 1981. [ Links ]
39. Renaudeau D, Collin A, Yahav S, De Basilio V, Gourdine JL, Collier RJ. Adaptation to hot climate and strategies to alleviate heat stress in livestock production. Animal 2012; 6:707-728. [ Links ]
40. Burfeind O, Suthar VS, Heuwieser W. Effect of heat stress on body temperature in healthy early postpartum dairy cows. Theriogenology 2012;78:2031-2038. [ Links ]
41. Vizzotto EF, Fischer VA, Thaler NAS, Abreu MT, Stumpf D, Werncke FA, et al. Access to shade changes behavioral and physiological attributes of dairy cows during the hot season in the subtropics. Animal 2015;9:1559-1566. [ Links ]
42. Meyer U, Everinghoff M, Gädek D, Flachowsky G. Investigations on the water intake of lactating dairy cows. Livest Prod Sci 2004;90:117-121. [ Links ]
43. Spiers DE, Spain JN, Sampson JD, Rhoads RP. Use of physiological parameters to predict milk yield and feed intake in heat-stressed dairy cows. J Thermal Biol 2004;29:759-764. [ Links ]
44. Tao S, Dahl GE. Invited Review: Heat stress effects during late gestation on dry cows and their calves. J Dairy Sci 2013;96:4079-4093. [ Links ]
45. Holter JB, West JW, McGilliard MI, Pell AN. Predicting ad libitum dry matter intake and yields of jersey cows. J. Dairy Sci 1996;79:912-921. [ Links ]
46. Rottman LW, Ying Y, Zhou K, Bartell PA, Harvatine KJ. The effects of feeding rations that differ in neutral detergent fiber and starch concentration within a day on production, feeding, behavior total-tract digestibility, and plasma metabolites and hormones in dairy cows. J Dairy Sci 2015;98 4673-4684. [ Links ]
47. Yadav B, Singh G, Verma AK, Dutta N, Sejian V. Impact of heat stress on rumen function. Vet World 2013;6(12):992-996. [ Links ]
48. Hassan A, Roussel JD. Effect of protein concentration in the diet on blood composition and productivity of lactating Holstein cows under thermal stress. J Agric Sci 1975;85: 409-415. [ Links ]
49. NRC. Nutrient requirements of dairy cattle. 7th Rev. Ed. Nat Acad Sci 2001; Washington, DC, USA. [ Links ]
50. Khelil-Arfa H, Bodon A, Maxin G, Faverdin P. Prediction of water intake and excretion flows in Holstein dairy cows under thermoneutral conditions. Animal 2012;10:1662-1676. [ Links ]
51. Burgos M, Senn M, Sutter F, Kreuzer M, Langhans W. Effect of water restriction on feeding and metabolism in dairy cows. Am J Physiol Regulatory Integrative Comp Physiol 2001;280(2):R418-R427. [ Links ]
52. Spek JW, Bannink A, Gort G, Hendriks WH, Dijkstra J. Effect of sodium chloride intake on urine volume, urinary urea excretion, and milk urea concentration in lactating dairy cattle. J Dairy Sci 2012;95:7288-7298. [ Links ]
53. Holter J, Urban W. Water partitioning and intake prediction in dry and lactating Holstein cows. J Dairy Sci 1992;75:1472-1479. [ Links ]
54. Ammer S, Lambertz C, von Soosten D, Zimmer K, Meyer U, Dänicke S, et al. Impact of diet composition and temperature-humidity index on water and dry matter intake of high-yielding dairy cows. J Anim Physiol Anim Nutr 2018;102(1):103-113. [ Links ]
55. Roussel AJ. Fluid therapy in mature cattle. Vet Clin North Am Food Anim Pract 1999;15: 545-557. [ Links ]
56. Pawar MM, Srivastaba AK, Chauhan HD, Damor SV. Nutritional strategies to alleviate heat stress in dairy animals - A Review. Int J Livest Res 2018;8(1):8-18. [ Links ]
57. Cook NB, Mentink RL, Bennet TB, Burgui K. The effect of heat stress and lameness on time budget of lactating dairy cows. J Dairy Sci 2007;90:1674-1682. [ Links ]
58. Beatty DT, Barnes A, Taylor E, Pethick D, McCarthy M, Maloney SK. Physiological responses of Bos taurus and Bos indicus cattle to prolonged, continuous heat and humidity. J Anim Sci 2006;84:972-985. [ Links ]
59. Ji B, Banhazi T, Perano K, Ghahramani A, Bowtell L, Wang C, et al. A review of measuring, assessing and mitigating heat stress in dairy cattle. Biosyst Eng 2020;199:4-26. [ Links ]
60. Cardot V, Le Roux Y, Jurjanz S. Drinking behavior of lactating dairy cows and prediction of their water intake. J Dairy Sci 2008;91:2257-2264. [ Links ]
61. Dado RG, Allen MS. Intake limitations, feeding behavior, and rumen function of cows challenged with rumen fill from dietary fiber or inert bulk. J Dairy Sci 1995;78:118-133. [ Links ]
62. Brscic MF, Gottardo A, Mazzenga A, Cozzi G. Behavioral response to different climatic conditions of beef cattle in intensive rearing systems. Poljoprivreda 2007;13(1):1-5. [ Links ]
63. Jago JG, Roche JR, Kolver ES, Woolford MW. The drinking behavior of dairy cows in late lactation. Proc NZ Soc Anim Prod 2005;65:2209-2014. [ Links ]
64. Pinheiro MFLC, Teixeira DL, Weary DM, vonKeyserlingk MAG, Hötzel MJ. Designing better water troughs: dairy cows prefer and drink more from larger troughs. Appl Anim Behav Sci 2004;89:185-193. [ Links ]
65. Osborne VR, Hacker RR, McBride BW. Effects of heated drinking water on the production responses of lactating Holstein and Jersey cows. Can J Anim Sci 2002;82:267-273. [ Links ]
66. Lanham JK, Coppok CE, Milam KZ, LaBore JM, Nave DH. Effect of drinking water temperature on physiological responses of lactating Holstein cows in summer. J Dairy Sci 1986;69:1004-1012. [ Links ]
67. Wilks DL, Coppock CE, Lanham JK, Brooks KN, Baker CC, Bryson WL, et al. Responses of lactating Holstein cows to chilled drinking water in high ambient temperatures. J Dairy Sci 1990;73:1091-1099. [ Links ]
68. Berman A. Estimates of heat stress relief needs for Holstein dairy cows. J Anim Sci 2005;83:1377-1384. [ Links ]
69. Barash H, Silanikove N , Shamay A, Ezra E. Interrelationships among ambient temperature, day length, and milk yield in dairy cows under a Mediterranean climate. J Dairy Sci 2001;84(10):2314-2320. [ Links ]
70. Ravagnolo O, Miztal I. Genetic component of heat stress in dairy cattle, parameter estimation. J Dairy Sci 2000;83(9):2126-2130. [ Links ]
71. Tao S, Orellana Rivas RM, Marins TN, Chen YC, Gao J, Bernard JK. Impact of heat stress on lactational performance of dairy cows. Theriogenology 2020;150:437-444. [ Links ]
72. Bernabucci U, Lacetera N, Ronchi B, Nardone A. Effects of the hot season on milk protein fractions in Holstein cows. Anim Res 2002;51:25-33. [ Links ]
73. Calamari L, Mariani P. Effects of hot environment conditions on the main milk cheese making characteristics. Zoot Nutr Anim 1998;24:259-271. [ Links ]
74. Kumar S, Alex R, Gaur GK, Mukherjee SS, Mandal DK, Singh U, et al. Evolution of Frieswal cattle: A crossbred dairy animal of India. Indian J Anim Sci 2018;88(3):265-275. [ Links ]
75. Alfonzo EPM, Barbosa da Silva MVG, dos Santos Daltro D, Tempel Stumpf M, Calderaro Dalcin V, Kolling G, et al. Relationship between physical attributes and heat stress in dairy cattle from different genetic groups. Int J Biometeorol 2016;60:245-253. [ Links ]
76. Ramírez-Rivera EJ, Rodríguez-Miranda J, Huerta-Mora IR, Cárdenas-Cágal A, Juárez-Barrientos JM. Tropical milk production systems and milk quality: a review. Trop Anim Health Prod 2019;51(6):1295-1305. [ Links ]
77. Collier RJ, Collier JL, Rhoads RP, Baumgard LH. Invited review: genes involved in the bovine heat stress response. J Dairy Sci 2008;91(2):445-454. [ Links ]
78. Bhanuprakash V, Singh U, Sengar G, Sajjanar B, Bhusan B, Raja TV, et al. Differential effect of thermal stress on HSP70 expression, nitric oxide production and cell proliferation among native and crossbred dairy cattle. J Thermal Biol 2016;59:18-25. [ Links ]
79. Deb R, Sajjanar B, Singh U, Kumar S, Singh R, Sengar G, et al. Effect of heat stress on the expression profile of Hsp90 among Sahiwal (Bos indicus) and Friswal (Bos indicus x Bos taurus) breed of cattle: A comparative study. Gene 2014;536:435-440. [ Links ]
80. Bhanuprakash V, Singh U, Sengar GS, Raja TV, Sajjanar B, Alex R, et al. Comparative expression profile of NOD1/2 and certain acute inflammatory cytokines in thermal-stressed cell culture model of native and crossbred cattle. Int J Biometeorol 2017;61:931-941. [ Links ]
81. Lacetera N, Bernabucci U, Scalia D, Basiricò L, Morera P, Nardone A. Heat stress elicits different responses in peripheral blood mononuclear cells from Brown Swiss and Holstein cows. J Dairy Sci 2006;89(12):4606-4612. [ Links ]
82. Sigdel A, Abdollahl-Arpanahl R, Aguilar I, Peñagaricano F. Whole genome mapping reveals novel genes and pathways involved in milk production under heat stress in US Holstein cows. Front Genet 2019;10:928. [ Links ]
83. Hernández-Cordero AI, Sánchez-Castro MA, Zamorano-Algandar R, Luna-Nevárez P, Rincón G, Medrano JF, et al. Genotypes within the prolactin and growth hormone insulin-like growth factor-I pathways associated with milk production in heat stressed Holstein cattle: Genotypes and milk yield in heat stressed Holstein cows. Genet Mol Res 2017;16(4):gmr16039821. [ Links ]
84. González ME, González VM, Montaño MF, Medina GE, Mahadevan P, Villa C, et al. Genome-wide association analysis of body conformation traits in Mexican Holstein cattle using a mix of sampled and imputed SNP genotypes. Genet Mol Res 2017;16(2):gmr16029597. [ Links ]
85. Dikmen S, Cole JB, Null DJ, Hansen PJ. Genome-wide association mapping for identification of quantitative trait loci for rectal temperature during heat stress in Holstein cattle. PLoS One 2013;8:e69202. [ Links ]
86. Macciotta NPP, Biffani S, Bernabucci U, Lacetera N, Vitali A, Ajmone-Marsan P, et al. Derivation and genome-wide association study of a principal component-based measure of heat tolerance in dairy cattle. J Dairy Sci 2013;100:4683-4697. [ Links ]
87. Liu Y, Li H, Zhou X, Wang G. A novel SNP of the ATP1A1 gene is associated with heat tolerance traits in dairy cows. Mol Biol Rep 2011;38:83-88. [ Links ]
88. Badri TM, Chen KL, Alsiddig MA, Li L, Cai Y, Wang GL. Genetic polymorphism in Hsp90AA1 gene is associated with the thermotolerance in Chinese Holstein cows. Cell Stress Chaperones 2018;23:639-651. [ Links ]
89. Dikmen S, Khan FA, Huson HJ, Sonstegard TS, Moss JI, Dahl GE, Hansen PJ. The SLICK hair locus derived from Senepol cattle confers thermotolerance to intensively managed lactating Holstein cows. J Dairy Sci 2014;97(9):5508-5520. [ Links ]
90. Verma N, Gupta ID, Verma A, Kumar R, Das R, Vineeth MR. Novel SNPs in HSPB8 gene and their association with heat tolerance traits in Sahiwal indigenous cattle. Trop Anim Health Prod 2016;48:175-180. [ Links ]
Received: October 27, 2020; Accepted: July 12, 2021