Services on Demand
Journal
Article
Indicators
-
Cited by SciELO
-
Access statistics
Related links
-
Similars in SciELO
Share
Revista mexicana de ciencias agrícolas
Print version ISSN 2007-0934
Rev. Mex. Cienc. Agríc vol.10 n.2 Texcoco Feb./Mar. 2019
https://doi.org/10.29312/remexca.v10i2.871
Investigation note
Design, construction, verification and testing of a low cost germinator
1Universidad del Papaloapan-Campus Tuxtepec. Circuito Central 200, Col. Parque Industrial, San Juan Bautista, Tuxtepec, Oaxaca, México. CP. 68300. Tel. 01(287) 8759240. (axavila@unpa.edu.mx; fmendoza@unpa.edu.mx).
2Instituto Nacional de Investigaciones Nucleares-Departamento de Sistemas Nucleares. Carretera México-Toluca s/n, La marquesa, Ocoyoacac, México. CP. 52750. Tel. 01(55) 53297200, ext. 12497. (hector.hernandez@inin.gob.mx).
Automated germinators are an indispensable tool to optimize agricultural processes. However, they have high costs, so the design, construction and operation of devices with accessible prices for small producers is required. The objective of this work was to design, build and verify a prototype of low cost germinator, under the guidelines of a prototype design. Said germinator was built using materials available in the region and is able to automatically control the temperature and photoperiod through circuits W1401 and Tem-8 48386 respectively. Furthermore, by means of simple switches, the light intensity can be controlled. Additionally, a temperature verifier was built with an Arduino Uno card. The verification system allowed the monitoring of temperature changes in real time (every minute). The prototype was tested by germinating seeds of Capsicum chinense, using different volumes of imbibition water. According to the results obtained, the built prototype is adjusted to the requirements recommended by the International seed testing association (ISTA), as well as by the Food and Agriculture Organization (FAO) for the evaluation of seeds, but also showed a higher yield, compared to a commercial incubator and even a very minor construction and operation cost.
Keywords: arduino; germination; low cost
Los germinadores automatizados, son una herramienta indispensable para optimizar los procesos agrícolas. Sin embargo, tienen altos costos, por lo que se requiere el diseño, construcción y operación de aparatos con precios accesibles para pequeños productores. El objetivo del presente trabajo fue, diseñar, construir y verificar un prototipo de germinador de bajo costo, bajo las directrices de diseño de un prototipo. Dicho germinador, se construyó usando materiales disponibles en la región y es capaz de controlar automáticamente la temperatura y fotoperiodo mediante los circuitos W1401 y Tem-8 48386 respectivamente. Además, mediante conmutadores simples se puede controlar la intensidad lumínica. Adicionalmente, se construyó un verificador de temperatura con una tarjeta Arduino Uno. El sistema de verificación permitió el monitoreo de los cambios de temperatura en tiempo real (cada minuto). El prototipo se probó germinando semillas de Capsicum chinense, usando distintos volúmenes de agua de imbibición. De acuerdo con los resultados obtenidos, el prototipo construido se ajusta a los requerimientos recomendados tanto por la International seed testing association (ISTA), como por la Food and Agriculture Organitation (FAO) para la evaluación de semillas, pero, además mostró un rendimiento mayor, en comparación con una incubadora comercial y aun costo de construcción y operación muy menor.
Palabras clave: arduino; bajo costo; germinación
The germination process of the seeds is linked to the beginning of human civilization (Moreno-Casasola, 1996). The study of germination allowed to domesticate and select plant species, such as Zea mays L. (7000 BC.) and Phaseolus vulgaris L. (5000 BC.) (McDonald and Copeland, 1997; Hirst, 2017). At the beginning of the 20th century, the germination of seeds of commercial interest began to be studied systematically, but until the decades of the 50’s, 60’s and 70’s, with the so-called ‘green revolution’ (FAO, 1996; Borlaug and Dowswell, 2011) the technological development was achieved that allowed to have a strict control of the environmental variables.
The first germinators, closet type, were developed by Oomen-Koppe (1969) and Justice (1972). Currently, FAO recommendations are based on these authors and teams must meet the requirements listed in Table 1.
Table 1 Specifications of the germinators according to international organizations.
Characteristics | FAO/ISTA | Commercial incubator |
Humidity [%] | 70-90 | Amb-99 |
Temperature range [°C] | 10-35 | 0-50 |
Temperature variation [°C] | ± 1 | ±1 |
Light [lx] | 750-1250 | 0-15000 |
Movement of the air | Minimum | Continuous |
Supply of fresh air | 1 replacement hr-1 | Not specified |
Cycle day/night [hr] | 8-12/16-12 | Programmable |
Condensation | Null | Null |
Source: built with data from Oomen and Koppe (1969) and the operations manual of the Kenton PQH-150S germinator
Currently, in the agricultural companies there are laboratories, where feasibility and vigor are analyzed, according to the standards of the International Seed Testing Association (ISTA, 2017) or the United Nations Organization for Food and Agriculture (FAO, 2003). These laboratories have facilities and ad hoc equipment for the routine execution of these tests, in order to guarantee the optimal germination characterization conditions (FAO, 2003; ISTA, 2017).
Germination tests allow the detection of lots of seeds, with low levels of germination, either due to excessive storage time or the lack of the genetic attributes necessary to face the environmental challenges of the plots where they are to be planted. These tests allow decisions to be made without exposing the money, both the cost of the seeds, as well as the operations of sowing, spending on fuels and wages (Rodríguez et al., 2008).
The equipment used in these tests, indispensable for modern agriculture, is expensive and costly, unattainable for small producers interested in the efficient management of their resources (FAO, 2003). Therefore, there is a need to have germinators, with automated monitoring systems that control temperature, lighting and photoperiod, but with low cost and low level of technology (Tapia, 2002; Urquia, 2014), which allows producers enthusiastic, with basic knowledge in electronics and electricity or laboratories of agronomy schools in developing countries, its design, construction and validation.
Currently, electronics have had a very fast development and have generated a wide variety of digital circuits of last generation, which are moving to simpler circuits, which are still robust and their costs are increasingly accessible. This is the case of circuits W1401 and W1209, they can have average retail prices of $10.50 and $5.50 USD, respectively. There are also analog timers (TEM-8 48386) and digital timers (DK-C-02) with an average price of $7.20 and $7.75 USD, respectively.
Additionally, there are circuits such as the Arduino Uno board, which functions as an open-source prototype platform, which has its own programming environment and which may have a cost in Mexico, retailing at $12.80 USD. In the case of Arduino, it has the advantage of being a very versatile platform and of having a great variety of peripherals, sensors and actuators. In addition, it has a large number of programs or routines (sketch’s) of free access and in some cases, already tested, which allows it to be able to control all the factors, since it can be connected to a computer and work as a control card (McRoberts, 2009; Monk, 2010; Timmis, 2011).
However, in the case of the Arduino Uno board, it requires that the sketch work properly and in case it fails or requires adjustments, it is necessary to have knowledge in programming and electronics, relatively specialized to be able to handle it. Instead, you can establish a simple connection that allows you to function as a data acquisition card and the results can be processed in common spreadsheets, such as Microsoft Excel or Office Libre in order to analyze them and verify the operation of an incubator in real time (McRoberts, 2009; Monk, 2010).
The objective of the work was to design, build and verify a germinator, low cost and low technical level that can be used routinely in research work and that lends itself to transfer technology to the rural environment, based on the guidelines of design, construction, verification and testing of a prototype.
The design of the germinator was made in such a way that its construction was simple. The team consists of two main parts, the incubator and the verification team. In turn, the incubator was subdivided into three sections: a) the temperature control circuit (CCT); b) wardrobe; and c) the lid or cabinet door with brightness control (CL). In Figure 1, the schematic diagram of the germinator and the verifying equipment is shown. It should be noted that all the materials, including the circuits, the plywood, the hardware, the fittings, the frames and the lamps, as well as the materials used in the verification equipment, had a total cost of $54.8 USD.
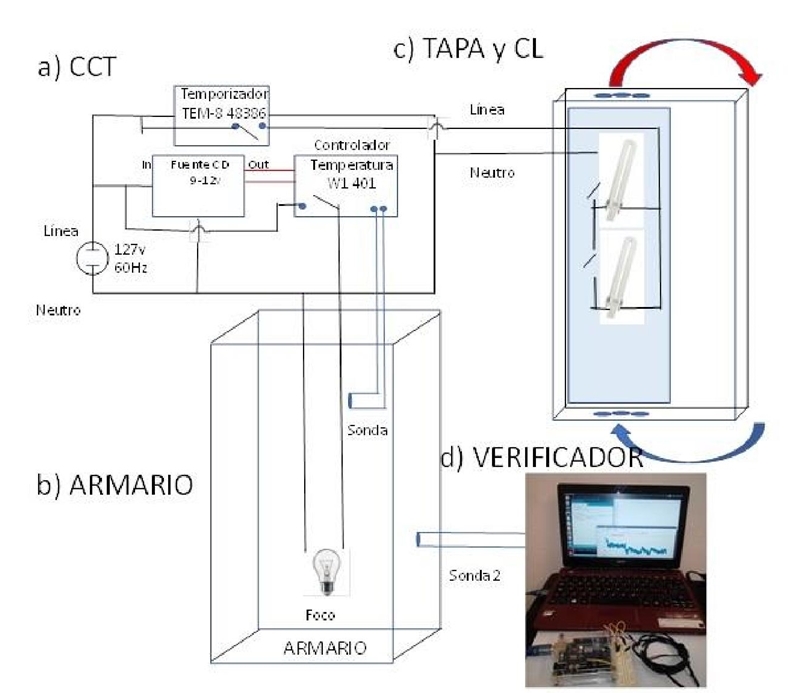
Figure 1 Schematic and electrical diagram. The general electrical circuits are shown from the power supply to 127 V to the period photocontroller and the voltage reduction to 12 v DC (red lines), which feed the temperature control circuit.
The CCT (Figure 1a) is designed to use a 12 V power supply, a photoperiod controller and a temperature controller circuit. The incubator cabinet was constructed with removable frames in order to allow the flow of hot air from the lower zone. The removable frames were constructed of plywood using wire mesh as a background, with a separation of 2 cm, with respect to each of the four internal sides of the cabinet. With this, it was possible to generate the convection of the air and the temperature became passively uniform avoiding the use of fans, on the one hand, they increase the drying of the plants or the substrates and on the other, they make the circuit more complicated.
The temperature control circuit is connected to the housing and the veiled incandescent bulb, which supplies heat. The intensity of the lighting is achieved by a simple on/off circuit of compact fluorescent lamps (CFL).
The door or lid contains a compartment, which contains LFC’s that provide lighting. In order that the lamps did not introduce heat energy into the interior of the chamber, the lamps were isolated, allowing only the passage of diffused light and the generated heat is extracted by a passive system casing-tube type. Where, the air, by convection forces the efficient exchange of heat. A timer controls the ignition of the LCF’s, placed on the lid.
The verifier circuit was designed and built using an Arduino Uno card, with an ATMega 328 processor at 16 MHz and 0.5 KB of internal memory. The card was programmed in its own working environment (version 1.7.11, http://www.arduino.org/downloads), through the USB port of an Acer One Notebook AMD E1 processor at 1 GHz with 2 GB of RAM. The electronic circuit uses a resistance of 22 KΩ ±5% and a thermistor of 10 KΩ nominal value (real 7.8KΩ 35 °C) as shown in Figure 2. The code was developed in Arduino environment and was modified to adjust it to the real values of the thermistor (Monk, 2010).
Calculation of energy efficiency
The electric consumption of the germinator was compared with that of a commercial incubator (Felisa mod FE-132AD), measuring the temperature with the verifier and the electrical consumption by means of the Fluke 377 multimeter, for 15 min. With the results, the performance of both devices was calculated, depending on the volume of air inside the working chamber of the appliances, in the range of 33 to 36 °C, the results were divided into two stages: the start and the operation.
The start was considered 33-36 °C (approximately 6 min). The operation stage was measured from minute 6, until 15 min. For the calculation of energy efficiency, the system was considered as a steady flow. The relationship between heat and temperature change is normally expressed in the form shown in equation 1, where Cs is the specific heat. While the power supplied by the electrical resistance can be evaluated by the mathematical expression of Joule’s law (equation 2).
For the heat calculations of the system (Q), the following formula was used.
Where: m= average air mass; Cs= specific heat of air at 34.5 °C; T2= final temperature 36 °C; T1= initial temperature 33 °C.
The value of Q was related to the electrical power consumed by the resistance of the heating element (light bulb vs. matched resistance) in the following way.
Where: Qabs= is the necessary heat that is supplied to a certain mass to raise its temperature; W (supplied)= is the electrical power that is needed to generate the Qabs heat.
To determine the efficiency of the germinator (Eta), the ratio between the power obtained between the power supplied was calculated:
Where: po= is the power obtained; p= is the power supplied.
Test germination test
Seeds of C. chinense, variety Jaguar, from the Experimental Field Las Huastecas of INIFAP were used. The seeds were treated by the supplier with Pirimifos and Thiram 42% and packed in a dark and vacuum bag. Subsequently, the pre-conditioning experiments were started for the evaluation of germination percentage.
The pre-conditioning and germination were performed in a Petri dish of 10 cm in diameter, where 2 layers of rustic paper (estraza) were placed, food grade (without chemical treatment) and 20 seeds were distributed equidistantly, on top of the two layers of paper and seeds were placed on a layer of flannel previously washed with distilled water three times and dried, on top of which, the respective volumes of distilled water were poured. In this case, they were: 2.5, 3.5 and 7.5 mL. These volumes were chosen because they were around the volume used by other authors to imbibe the seeds (Garruña-Hernández, 2014). The number of germinated seeds was determined daily, for nine days. The results were expressed as daily percentage of germinated seeds.
The prototype was built, based on the design described above, in the chemical-biological laboratory of the UNPA located in San Juan Bautista, Tuxtepec. The materials in their totality were acquired in the region or through national pages not specialized in electronic components.
Through the validation system based on Arduino, the environmental temperature of the laboratory and inside the germinator was monitored, every minute, for 24 h. The behavior of temperature variations in a standard work day is shown in Figure 3.

Figure 3 Comparison of temperature variations between the interior of the germinator and the environment. a) corresponds to the moment of opening the incubator for the collection of experimental data; b) the moment in which the lighting lamps are turned on; and c) corresponds to the turning on of the laboratory air conditioning.
The average of the daily temperature inside the germinator was 27.68 ±0.567 °C, while in the laboratory it was 27.97 ±1.199 °C. That is to say, that the variation of temperature inside the germinator was reduced by 32% with respect to the variation of the ambient temperature and a better control and adjustment to the desired temperature was achieved. On the other hand, the variation is within the range of specifications for a laboratory germinator required by ISTA (2017) and FAO (2003). And it is similar to that reported for other commercial germinators (Table 1).
Comparison of energy efficiency with a commercial incubator
The objective of designing low cost devices implies a decrease in manufacturing and maintenance expenses, as well as in energy consumption expenses. Making efficient systems, designed to take full advantage of the energy supplied, is of great importance if we want to save energy. In terms of energy consumption, an energy efficient system is one that has maximum performance; that is, it makes the most of the energy we provide (Bautista-Guevara, 2013).
To calculate the heat, the following procedure was carried out: the germinator has a volume of 225 L (0.225 m3) of air, using the average value of the air density between 33 and 36 °C (1135 kg m-3) and an atmosphere of pressure, the mass of air inside the germinator was calculated to be 0.2554 Kg. The change in temperature was 3 °C (3 °K), using equation (1) the calculation of heat it gives us a value of 0.775 KJ which is the energy necessary to pass from 33 to 36 ℃ the mass of air, assuming that the walls of the germinator do not absorb heat.
To calculate the performance of the germinator, the power consumption of a resistance of a nominal 100 w bulb was first calculated for the evaluation of the starting stage. The time it took to complete the boot is the same for the commercial incubator and was 5 minutes. With the formula for electrical circuits.
Where: P= is power in watts; V= is voltage; I= is current in amperes.
The power is related to the electric current that is used at a constant potential difference, in this way, the total current of the starting period was 3.4 amps and the voltage of 120 volts gives a power of 408 watts used to raise the temperature of a certain mass of air in a period of time of 6 minutes, its power at the start time was 2 448 KJ, so the yield for the germinator was 31.67%.
For the commercial incubator, the same measurements of electric current and heating of its internal air mass and with the same temperature intervals were made. The volume used was 84.6 L (0.0846 m3); that is, a mass of 0.096 kg and with this the heat needed to heat that mass of air was 0.2915 KJ. For the calculation of the yield, the total current used was 6.2 Amps, the power used was 744 watts and the yield was 6.53%.
The difference in performance between the commercial incubator and the built germinator was 25% favoring the germinator, despite the difference in air masses. The current consumption was higher for the incubator, 6.2 Amperes, while for the germinator it was 3.4 Amperes. Finally, to prove its functioning, the germinator was used to determine the germination time for seeds of habanero pepper (Capsicum chinense) var Jaguar. Which is very appreciated, but it is considered that asynchronous, in its germination Garruña-Hernández et al. (2014).
The germination was tested by programming at 28 °C and with different water supply regimes 2.5, 3.5 and 7.5 ml of water (Figure 4). The differences in the percentage of germination observed in the analyzed times depend on the volume of imbibition and not on the temperature (p≤ 0.05), a direct proportional relationship was also found between the percentage of germination and the volume of imbibition water with a Pearson coefficient: -0.905 and a p= 0 (data under review).
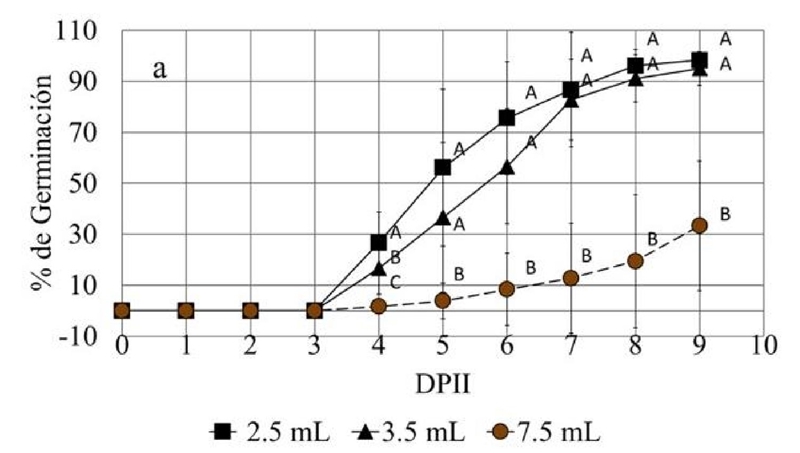
Figure 4 Effect of different volumes of imbibition water on the germination of C. chinense. The seeds were imbibed with 2.5 and 3.5 ml of distilled water, compared to the reference volume (7.5 ml). Germination was evaluated daily.
In summary, a germinator was built, with an internal volume of 225 L, with automatic control of photoperiod, temperature and manual lighting control. The temperature variation is only 0.567 °C, the total cost was US$ 58, which makes it competitive with respect to other commercial germinators that can cost between US$ 1 000 to US$ 2 500 and the time of maximum construction was 4 days.
Conclusions
In the present work, a germinator was built with a very competitive total manufacturing cost ($54.8 USD), even against non-scientific commercial equipment that has not been verified. The verification element, made up of the Arduino card and the computer, allows to collect data in real time, which allows to monitor the operation and make calculations of the germination performance.
The germinator showed, have a high energy efficiency, which can be attributed to design factors, but can also be attributed to the heating elements. It is proposed that the incandescent bulbs are more efficient heating elements than the electrical resistance plugs, perhaps because the tungsten filament requires less energy to reach the threshold temperature and this temperature is higher in the tungsten (of the bulb) than in nickel (from the jacketed resistance).
Literatura citada
Bautista, G. F. 2013. Eficiencia energética y conservación de energía: perspectiva para un desarrollo sostenible. Revista peruana de energía. 3(1):57-87. [ Links ]
Borlaug, N. E. y Dowswell, C. 2011. La inacabada Revolución Verde- el futuro rol de la ciencia y la tecnología en la alimentación del mundo en desarrollo. Agbioworld USA. http://www.agbioworld.org/biotech-info/articles/spanish/desarrollo.html. [ Links ]
FAO. 1996. Enseñanzas de la revolución verde: hacia una nueva revolución verde, Cumbre mundial sobre la alimentación. http://www.fao.org/docrep/003/w2612s/w2612s06.htm. [ Links ]
FAO. 2003. Semillas de calidad declarada. http://www.fao.org/docrep/3/a-a0503s.pdf. [ Links ]
Garruña, H. R.; La Tournerie, M. L.; Ayala, G. O.; Santamaría, J. y Pinzón, L. L. 2014. Acondicionamiento pre-siembra: una opción para incrementar la germinación de semillas de chile habanero (Capsicum chinense Jacq.). Agrociencia. 48(4):413-423. [ Links ]
Hirst, K. H. 2017. Plant domestication. Table of dates and places. trought Co. https://www.thoughtco.com/plant-domestication-table-dates-places-170638. [ Links ]
ISTA. 2017. International rules for seed tensting. International seed testing assositing. http://www.seedtest.org/en/internationalrules-content-1-1083.html. [ Links ]
Justice, O. L. 1972. Essentials of seed testing. In: seed biology. Ed. Kozlowski, T.T. Academic Press New York and London. 3:301-370. [ Links ]
McDonald, M. B. and Copeland, L. O. 1997. Seed production: principles and practices. Springer US. 749 p. [ Links ]
McRoberts, M. 2009. Earthshine design arduino starters kit manual - a complete beginners guide to the Arduino earthshine design. http://math.hws.edu/vaughn/cpsc/226/docs /askmanual.pdf. [ Links ]
Monk, S. 2010. 30 Arduino™ projects for the evil Genius™. The McGraw-Hill. USA. 3ra . rev. http://www.honfablab.org/wordpress/alexandria/technology/30Arduino-Projects-EviGenius.pdf. [ Links ]
Moreno, C. P. 1996. Vida y obra de granos y semillas. Col. La ciencia para todos. 2a (Ed.). Fondo de Cultura Económica (FCE). México DF. [ Links ]
Oomen, W. A. and Koppe, R. 1969. Germination cabinets with day and night cycles. Proc. Int. Seed Test. Ass. 34(1):103-114. [ Links ]
Rodríguez, I.; Adam, G. y Durán, J. M. 2008. Agricultura. tecnología. España. 912:836-842. http://www.mapama.gob.es/ministerio/pags/Biblioteca/Revistas/pdf-Agri%2FAgri-2008-912-completa.pdf. [ Links ]
Tapia, A. 2002. El proceso de investigación y transferencia de tecnología en el sector agrícola. La experiencia del INIFAP. Aportes. Benemérita Universidad de Puebla (BUAP). Puebla-México. 20(7):179-183. [ Links ]
Timmis, H.. 2011 Practical Arduino engineering. 1ra . (Ed). Apress. USA. 328 p. http://www.apress.com/us/. [ Links ]
Urquía, N. 2014. Transferencia de tecnología en el desarrollo rural. FAO. México, DF. https://coin.fao.org/coinstatic/cms/media/20/13941367738530/fao-transferencia-de-tecnologa-en-el-desarrollo-ganadero.pdf. [ Links ]
Received: January 2019; Accepted: February 2019