Services on Demand
Journal
Article
Indicators
-
Cited by SciELO
-
Access statistics
Related links
-
Similars in SciELO
Share
Revista mexicana de ciencias agrícolas
Print version ISSN 2007-0934
Rev. Mex. Cienc. Agríc vol.8 n.5 Texcoco Jun./Aug. 2017
https://doi.org/10.29312/remexca.v8i5.106
Articles
Genotype-environment interaction, stability of yield and grain quality in tuxpeño maize
1Programa de Genética, Posgrado de Recursos Genéticos y Productividad-Colegio de Postgraduados-Campus Montecillo. Carretera México-Texcoco km 36.5. Montecillo, Texcoco, Estado de México. CP. 56230. Tel. 01 (595) 9520200. (fernando.lopez@colpos.mx; zavala@colpos.mx; tcoranat@colpos.mx; sercruz@colpos.mx).
2Campo Experimental Valle de México-Instituto Nacional de Investigaciones Forestales, Agrícolas y Pecuarias. Carretera los Reyes-Texcoco km 13.5. Coatlinchán, Texcoco, Edo. de México. CP. 56250. Tel. 01 (595) 9212742. (esquivel.gilberto@inifap.gob.mx).
3Colegio de Postgraduados-Campus Veracruz. Carretera federal Xalapa-Veracruz km 88.5. Veracruz, Veracruz, México. CP. 91700. Tel. 01 (229) 2010770. (gustavolr@colpos.mx).
4Benemérita Universidad Autónoma de Puebla, Facultad de Ingeniería Agrohidráulica. San Juan Acateno, Teziutlán, Puebla. CP. 73695. Tel. 01 (231) 3122933.
Maize (Zea mays L.) is an essential component of the Mexican diet, with an average of 120 kg per capita consumption. The Tuxpeño breed has stood out for its high yield and its wide adaptation so that once adapted to temperate climate it is necessary to evaluate the quality of its grains with the parameters of the processing industries. In this research the effect of the genotype*environment interaction on yield and physical characteristics of the grain of 14th and 19th cycles of Tuxpeño maize adapted by mass visual selection (SMV) in the Valles Altos of Mexico was studied. In the 2013 and 2014 years, the original population ‘C0’ and the cycles ‘C14’ and ‘C19’ of SMV were evaluated, both in its original environment, moist tropics in the State of Veracruz, and in its selection environment, temperate subhumid in the State of Mexico. The evaluated characteristics were: grain yield (REN), flotation index (IF), hectolitric weight (PH), pedicel, pericarp and grain germ. The data were analyzed with the AMMI model (main additive effects and multiplicative interactions). The results indicate that selection for adaptation, crop conditions and the effect of the environment during its development favorably modified the REN and some of the grain quality characteristics of the genotypes. Montecillo 2013-PV and 2014 PV the best yield was recorded (3.5 t ha-1), outstanding ‘C19’ due to its stability, hard endosperm grains (<IF) and higher pH.
Keywords: Zea mays L.; adaptation; AMMI model; dough-tortilla industry; visual mass selection
El maíz (Zea mays L.) es componente esencial de la dieta de los mexicanos, con un consumo per capita promedio de 120 kg. La raza Tuxpeño ha destacado por su alto rendimiento y su amplia adaptación por lo que una vez adaptada a clima templado es necesario evaluar la calidad de su grano con los parámetros de las industrias procesadoras. En esta investigación se estudió el efecto de la interacción genotipo*ambiente en el rendimiento y las características físicas del grano de los ciclos 14 y 19 del maíz Tuxpeño adaptados mediante selección masal visual (SMV), a los Valles Altos de México. En 2013 y 2014, se evaluó la población original ‘C0’ y los ciclos ‘C14’ y ‘C19’ de SMV, tanto en su ambiente original, trópico húmedo en el estado de Veracruz, como en su ambiente de selección, templado subhúmedo en el Estado de México. Las características evaluadas fueron: rendimiento de grano (REN), índice de flotación (IF), peso hectolítrico (PH), pedicelo, pericarpio y germen de grano. Los datos fueron analizados con el modelo AMMI (efec tos principales aditivos e interacciones multiplicativas). Los resultados indican que la selección para adaptación, las condiciones del cultivo y el efecto del ambiente durante su desarrollo modificaron favorablemente el REN y algunas de las características de calidad del grano de los genotipos. En Montecillo 2013-PV y 2014-PV se registró el mejor rendimiento (3.5 t ha-1), sobresaliendo ‘C19’, por su estabilidad, granos de endospermo duro (< IF) y mayor PH.
Palabras clave: Zea mays L.; adaptación; industria masa-tortilla; modelo AMMI; selección masal visual
Introduction
In México, maize (Zea mays L.) is planted in approximately eight million hectares, it is the crop with the highest agricultural cultivation surface, with an average yield of 3.4 t ha-1 (SIAP, 2015). Maize is an essential component of the Mexican diet, according to the SE (2012) about half of domestic production (12 million tons) is intended for making tortillas, with a per capita consumption of 120 kg, equivalent to 328 g daily (Figueroa et al., 2001), elaborated either by the traditional process (maize-dought-tortilla) using nixtamalized maize dough, or by the combination of both.
The Tuxpeño maize breed has stood out for its high yield, grain quality and resistance to pests and diseases. This race predominates in the state of Veracruz, between altitudes from 0 to 500 m, where climatic humid tropical conditions prevail (Bellon et al., 2005; Wen et al., 2012).
In México, maize breeding programs have been limited in most cases to the use of a few widely adapted breeds: Tuxpeño, in the humid tropics, Celaya and Conico Norteño, in the Bajío; Chalqueño and Cónico, in Valles Altos (Castillo, 1993). However, there are evidences of use of tropical and subtropical germplasm adapted to temperate climates by selection, specifically in Valles Altos Centrales (Molina, 1983; Pérez et al., 2000; 2002; 2007), with gains in yield and adaptability. Tuxpeño race has been especially used by its large genetic base, crossing ability and yield per se, which increases the genetic diversity in any breeding programs (Wen et al., 2012).
The quality of maize grain is largely based on the physical characteristics for commercialization (SE, 2002). The nixtamalized flour industry (IHN) prefers to process maize with a weight of one hundred grains >33 g, flotation index (IF) ≤20, pedicel ≤2%, pericarp between 4.5-5.5% and germ ≤12%; while the dough and tortilla (IMT) industry demands grains with an IF between 13 and 62% of floating grains. Both industries require a test weight ≥74 kg hL-1 (Salinas et al., 2010; Vázquez et al., 2012).
Recent studies of grain and tortilla quality, the AMMI model (additive main effects and multiplicative interactions) has been used for stability and genotype x environment interactions (IG*A) analysis (Vázquez et al., 2012). In maize, this model has shown its efficiency to identify outstanding materials (González et al., 2009; Torres et al., 2011); it is also useful for graphically interpreting the response of genotypes, environments and IG*A; it has the advantage of occupying a minimal number of repetitions in the experiments, which reduces costs in testing and allows to include a significant number of treatments (Zobel et al., 1988; Crossa, 1990). This model has also been used to assess the stability parameters and basic grains quality, such as wheat (Triticum aestivum L.) (Hristov et al., 2010) and rice (Oryza sativa L.) (Fasahat et al., 2014).
In Valles Altos Centrales of México (>2 200 masl), a representative variety of the Tuxpeño breed of tropical origin was subjected to 14 and 19 cycles of visual mass selection (SMV) “year by year” for its adaptation, aiming to increase genetic diversity. The selection criterion was yield and good appearance of plants and cobs, so it was considered convenient to evaluate the yield of advanced selection cycles 14 and 19, together with the original material.
Despite the good yield of the adapted varieties, it is necessary to know the quality of the grain in order to determine which of the three populations meets the specifications demanded by the dough-tortilla and nixtamalized flour industries. The objective of this research was to determine the effect of the genotype*environment interaction on yield stability and physical characteristics of the grain in a variety of Tuxpeño maize subjected to different SMV cycles, evaluated both in its original climate (Veracruz, México), and in its adapted climate (Estado de México). This in order to know its yield potential and grain quality for the dough-tortilla and nixtamalized flour processing industries. The hypothesis proposed is that the genotype with the highest SMV will have the highest yield and will fulfill the characteristics required by the processing industries.
Materials and methods
Background of the evaluated genetic material
The germplasm used was a maize population of Tuxpeño race called ‛V-520C12 (Zero cycle (‘C0). The adaptation of Tuxpeño ‛C’ maize in Valles Altos was carried out “year by year” recurrently during 14 and 19 cycle (‛C14’ and ‛C19’) respectively, by means of visual mass selection (SMV). The criteria for selecting the ‛C14’ and ‛C19’materials for this research were: its reduced variation in early SMV cycles, grain yield, cob characteristics (large, cylindrical, healthy, with straight rows and serrated white grain) and plant characteristics (healthy, vigorous, without grooming, typical of Tuxpeño, etc) (Molina, 1983).
Starting sowing for the SMV with approximately 510 plants in each cycle and discarding the sickest, making possible fraternal crosses between the healthiest plants “year by year”. Initially all cobs were selected, but then when production was higher, only the best 50 cobs were selected visually to form the compound of each selection cycle. The adaptation of materials subjected to SMV (‛C14’ and ‛C19’) was carried out at the Experimental Field of the Postgraduate College, located in Montecillo, Texcoco, Estado de Mexico, at an altitude of 2 250 m, predominating temperate sub-humid climate (García, 1973).
Experiments location
The original ‛V-520C’ variety of the Tuxpeño ‛C0’ breed, as well as its ‘C14’ and ‘C19’ SMV cycles, were evaluated in three experiments from Valles Altos Centrales of México during 2013 and 2014 evaluated in Montecillo and only in 2014 in the town of Coatlinchán, both sites belonging to the municipality of Texcoco, Estado de Mexico. In the same years, three other trials were established with the same materials in the cycles: 2013-PV (spring-summer), 2014-OI (autumn-winter) and 2014-PV in Tepetates of Manlio Fabio Altamirano municipality, State of Veracruz, corresponding to the Gulf Slope (Table 1).
Table 1 Characteristics of the agronomic management and environmental conditions of the evaluation sites.

* = año de siembra; OI= otoño-invierno; PV= primavera-verano; Méx= Estado de México; Ver= estado de Veracruz; Tex= Texcoco; Man= Manlio Fabio Altamirano; £= fuente propio; †= precipitación total acumulada (del periodo siembra/cosecha) donde a= riego inicial + tres riegos auxiliares, b= riego inicial, c=10 riegos auxiliares; Máx= temperatura máxima y Mín= temperatura mínima (durante el periodo siembra/cosecha).
Agronomic management
Agronomic management and the general soil and climatic characteristics of the six localities are described in Table 1. The design used was randomized complete blocks with three replicates (Martínez, 1988), where the experimental unit consisted of two rows of 6 m in length, separated at 80 cm. Two seeds were planted every 50 cm, which made a total of 52 plants per plot, giving a population density of 50 thousand plants per hectare. 140-60 fertilizer formula was used (kg ha-1 of N-P) in Montecillo, while in Coatlinchán it was 140-40 (Table 1), the application of nitrogen was divided into two parts, the first at the time of planting and the second in the last cultivation, applying all the phosphorus at the time of sowing. In both localities an initial irrigation was applied, but only in Montecillo three helping irrigations were given during the crop cycle. While in Tepetates the spring-summer cycles of 2013 and 2014 were rainfed and the autumn-winter of 2014 irrigation was applied every 10 days, from sowing to grain filling. The fertilization dose was 110-46, which was divided as follows: 64-18 at the time of sowing and the rest at the last cultivation. In the six environments herbicide was applied to control weeds, with the active ingredients dicamba, atrazine and nicosulfuron, the doses used were those recommended in each product for maize cultivation in the respective regions.
Evaluated variables
The yield and physical characteristics of grain were evaluated as described by Vázquez et al. (2012, 2015). The yield (REN) was expressed in t ha-1 grain by dry weight of the cob with 12% moisture in each plot extrapolated to one hectare area. The physical characteristics of grain tested were: flotation index (FI), which is an indirect measure of grain hardness (Vázquez et al., 2011) and test weight (PH) (55-10-01 method; AACC, 2016). The pedicel percentages (PIG), pericarp (PEG) and germ (GEG) of the grain were determined following the methodology described by Salinas and Vázquez (2006).
Statistic analysis
Yield and physical characteristics of grain data were analyzed with AMMI model (Zobel et al., 1988, Crossa, 1990), using SAS statistical package for Windows, version 9.0 (SAS Institute, 2002). This model generated a biplot graph for each variable with its respective main component (CP).
Results and discussion
Analysis of variance found highly significant differences (p≤ 0.01) between environments (A), between genotypes (G) and genotype*environment interaction (IG*A) for all variables evaluated (Table 2).
Table 2 Average squares and significance level using the AMMI model of six Tuxpeños maize variables: ‘C0’, ‘C14’ and ‘C19’ evaluated in the state of Veracruz and Estado de Mexico (2013-2014).

**= p≤ 0.01; FV= fuente de variación; A= ambiente; G= genotipos; IG*A= interacción genotipo*ambiente; CP= componente principal; Pi= porcentaje acumulado; CV= coeficiente de variación; X= promedio de la variable; GL= grados de libertad; REN= rendimiento (t ha-1); IF= índice de flotación; PH= peso hectolítrico (kg hL-1); PIG= pedicelo de grano (%); PEG= pericarpio de grano (%); GEG= germen de grano (%).
Yield stability
The significance of the IG*A (p≤ 0.01) of REN, indicates that at least one of the tested genotypes showed better performance or adaptation to a specific environment; likewise, CP1 was highly significant, which was formed by the sum of squares of IG*A, which explained 78.08% of the variability. However, CP2 was not significant and only accounted for 21.92%, both components showed 100% of variability (Table 2).
According to Gauch and Zobel (1989), the AMMI model combines the technique of analysis of variance and the analysis of CP in multiplicative terms, to explain the variability of the IG*A, through a simultaneous graph. In the dimensional chart, CP1 (axis of the ordinates) expresses the differences in the main additive effects (G and A), with positive or negative values, considering that the further away from this axis, there will be more IG*A, and the ones with smaller distance to the axis, will be more stable. On the other hand, the multiplicative interaction effect (axis of the abscissa) shifts from lower to higher (from right to left) according to a scale of the variable, where the lines perpendicular to this axis will have similar means. In conclusion, G or A farther to the axes of ordinates will be more influenced by the IG*A and vice versa (Zobel et al.,1988).
Figure 1A shows that genotypes with low IG*A; that is, those who maintained its behavior across environments were the ‛C0’ ‛Original’ and ‛C14’ ‛intermediate’, with an average yield of 1.6 and 2.2 t ha-1, respectively; while genotype ‛C19’ ‛advanced’ showed a high GI*A across locations, but showed the highest average yield, 3.5 t ha-1 (equaling the national average by SIAP, 2015), representing an increase of 45.7% with respect to the yield of the ‛C0’ cycle. The results indicate that SMV has been effective in increasing favorable gene frequencies for yield and adaptation in Tuxpeño ‛V-520C’ maize, through 19 selection cycles, despite having been evaluated in both tropical (origin) and in tempered (adapted), surpassing the inferior cycles 0 and 14 in the grain yield. In this regard, Pérez et al. (2000, 2007) found similar results in varieties of the Tuxpeño breed, where the more advanced cycles in SMV showed higher yields than the lower cycles.

Figure 1 Average grain yield (a), flotation index (b), hectoliter weight (c), grain pedicel (d), grain pericarp (e), grain germ (f) and CP1 coefficients for the genotype*environment interaction of Tuxpeño maize: ‘C0’, ‘C14’ and ‘C19’ of SMV in the state of Veracruz (T= Tepetates) and Estado de Mexico (M= Montecillos and C= Coatlinchán), 2013-2014.
On the other hand, the Montecillo locality for the agricultural cycle 2013 (M 2013-PV) and 2014 (M 2014-PV) recorded the highest yields (Figure 1a). According to the vectors length the environments discriminated between the three genotypes.
Specifically, it can be mentioned that the environments of Montecillo (M 2014-PV, M 2013-PV), Coatlinchán (C 2014-PV) and Tepetates (T 2014-PV) showed similar effects on genotype discrimination; in contrast, the town of Tepetates in the evaluations: T 2013-PV and T 2014-OI did not discriminate well between genotypes, this could be due to the fact that it was not the environment where the SMV was performed. The two Montecillo environments (2013-PV and 2014-PV) had an average yield equal to ‛C19’ genotype (3.5 t ha-1), followed by T 2014-IO and T 2013-PV environments, the latter was the most stable; its average yields were 2.6 and 2.9 t ha-1, respectively. Finally, environments with lower yield were: C 2014-PV and T 2014-PV, with 1.3 and 0.8 t ha-1, respectively.
The high yields observed in M 2013-PV and M 2014-PV are explained because Montecillo was the selection environment; in addition, this locality had a good soil moisture, since it was planted with an initial irrigation and three irrigations were subsequently applied; there was also a good rainfall distribution, optimal average temperatures before the days at female flowering (DFF) and during the grain filling period (Figure 2a), a higher dose of N and P was used to fertilize, and the soils have a high clay content (Table 1). Here, Barrales et al. (1984) state that yield is associated not only with quantity but also with the distribution of water during the period of female flowering, a very sensitive stage to moisture deficit, since when the shoots are wet and exposed, the pollen is more likely to stay there, thus increasing the yield. The low yield in T 2014-PV was influenced by an excess of poorly distributed water throughout the plant’s development (Table 1 and Figure 2b).
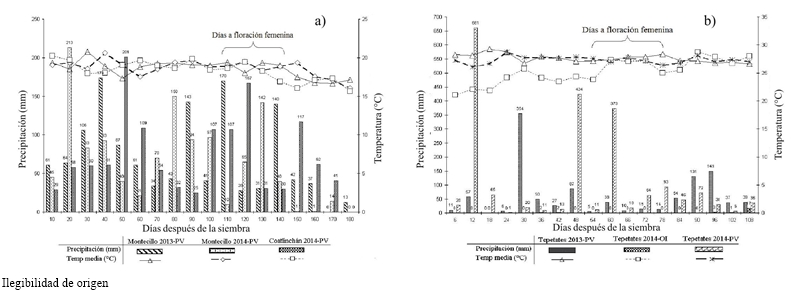
Figure 2 Thermopluviometric relationships of three localities, by municipality: a) Texcoco, México and b) Manlio Fabio Altamirano, Veracruz (2013-2014).
In the same way, the environment of C 2014-PV might have been influenced by a shallow soil (Phaeozem) and poor in P (Table 1), in addition, there was a poor distribution of precipitation, since shortly before female flowering (DFF) there was a remarkable water deficit (Figure 2a). The T 2013-PV and T 2014-OI sites had yields of 2.9 and 2.6 t ha-1, respectively, slightly above the average (Figure 1a). The T 2013-PV locality showed higher yields than its T2014-PV counterpart, the results could be attributed to a higher rainfall volume during female flowering days in 2013 (Figure 2b); however, the precipitation was poorly distributed, causing two deficits: one before and the other during the DFF, which could affect the period of grain filling thus reducing yield as reported by Tanaka and Yamaguchi (1972). A similar situation occurred in T 2014-OI, where temperatures were high and there was little rainfall and irrigation, which caused water stress throughout the development of the plant (Figure 2b). In this regard, Rincón et al. (2006) mentioned that one of the factors that have the greatest effect on the plant is the high temperature (>25 °C), as this results in a significant reduction of grain yield and biomass accumulation, with the cob being the most harmed, followed by a deficit of N in the two localities (Table 1).
Stability of physical characteristics of grain
The AMMI analysis detected high mean values for G, A and IG*A, CP1 and CP2 of the variables flotation index (IF), hectolitric weight (PH), pedicel percentage (PIG), pericarp percentage (PEG), and germ percentage (GEG); except PH at CP2 that was not significant. The CP1, explained the highest variability in the PH and GEG variables, with 92.61 and 86.08%, respectively, and it was the FI where the lowest percentage of variability was explained, with 63.53% (Table 2).
For the IF, the most stable genotype due to the hardness of its grains was ‘C14’, and the most unstable were ‘C0’ and ‘C19’, the most stable localities (low IG*A) were M 2013-PV and M 2014-PV, which also showed the highest yield (Figure 1b). On the other hand, the environments C 2014-PV and T 2014-PV were the most unstable for this variable (also those of lower yield). According to the scale reported by Salinas and Vázquez (2006) for IF,‘C0’ cobs had soft endosperm grains (IF= 72), C14 grains showed intermediate endosperm (IF= 49) and grains of ‘C19’ had hard endosperm (IF= 33). The IG*A, showed that only C 2014-PV had hard grains (IF= 13-37), whereas in Montecillo 2013 and 2014, as well as in Tepetates 2013 and 2014 (all rainfed), the grain texture was intermediate (IF= 38-62), leaving only the 2014-OI grains as soft (IF= 63-87).
Durum grain genotypes had the highest yields, results previously reported by Vázquez et al. (2012). Also, it was observed that the adaptation of the Tuxpeño maize with the SMV in Valles Altos is closely related to the FI, because to a greater selection the grain hardness increased; therefore, the soft grain genotype (‘C0’ with IF= 72) changed to hard grain in the ‘C19’ cycle (IF= 33); that is, the IF was reduced by 54.2%. This can be attributed to maize genetics and environmental conditions, which may have altered the organization and distribution of amylopectin chains in the starch granule, as well as the protein matrix present around the starch granules (Agama-Acevedo et al., 2013) and the SMV “year after year” indirectly achieving greater grain hardness. Meanwhile, Salinas et al. (2013) found for another variety of the Tuxpeño breed an IF of 51, which is different from the result of ‘V520C’ (‘C0’ with an IF of 72), this could be due to differences between varieties, but also because that study was performed in the state of Oaxaca, under different conditions from those of Valles Altos. Based on the IF, the genotypes‘C14’ and ‘C19’ are suitable for the preparation of tortillas following the traditional method of IMT. No genotype reached the hardness degree demanded by the IHN (IF< 20) (Figure 1b).
In PH, CP1 accounted for 92.62% of IG*A (Table 2). The BIPLOT graph for this parameter revealed that the most stable genotype was the ‘C19’ as well as the one with the highest test weight (74.8 kg hL-1), while genotypes ‘C14’ and ‘C0’ values were 73.5 and 69.2 of kg hL-1 respectively, being unstable between environments (Figure 1c); the genotype ‘C0’ was the most unstable (most remote to the axis of the ordinates) with positive interaction, due to its low frequency of genes for adaptation to Valles Altos. Regarding to localities, none of them discriminated well between genotypes, but the most stable in the IG*A was C 2014-PV, this locality reached the highest values for PH (76 kg hL-1), while T 2014-OI had the lowest overall mean (64.6 kg hL-1). The localities with most diverse PH (less stable) were M 2013-PV and T 2013-PV.
The localities that surpassed the PH average were C 2014-PV, T 2014-PV and T 2013-PV, which had a good but poorly distributed precipitation (Figure 2b), and their edaphoclimatic conditions were not less favorable (Table 1). The environment with lower PH was T 2014-OI, the results could be attributed to a minimum amount of precipitation and irrigation during the crop cycle, which limited grain development (Tanaka and Yamaguchi, 1972). In environments with greater precipitation or irrigation, the hectoliter weight was high. Moreover, the ‘C19’ (material with more SMV cycles) showed the highest pH (>74 kg hL-1) between genotypes and between locations, so it is considered suitable for IMT and IHN; this might be because the grain of this genotype was shorter, which could cause a greater compaction (greater number of grains in the container) when obtaining PH. A significant correlation between pH and IF was found (r= -0.72, p≤ 0.01), matching the indicated by Vázquez et al. (2012), thus the genotypes with higher PH were those of lower IF.
For pedicel percentage (PIG) high significance (p≤ 0.01) was observed in the IG*A, in addition its CP1 explained 72% of its interaction (Table 2). According to Figure 1d, the most stable genotype for PIG was ‘C0’, which showed a low average (1.33%), genotypes ‘C19’ (1.34%), and ‘C14’ (1.37%) were unstable. The ‘C14’ that had the highest PIG, also had a higher IG*A; that is, less stability. The locality that best discriminated between genotypes, from highest to lowest, was T 2013-PV, with an average of 1.77% (highest), followed by M 2013-PV (1.37%) and C 2014-PV, with an overall mean of 1.13% (the lowest). The most stable site for PIG was T 2014-PV, followed by T 2014-OI and M 2014-PV, with averages of 1.26%, 1.33% and 1.22%, respectively.
PIG results agree with those of Salinas et al. (2010), who evaluated tropical hybrid materials in the State of Veracruz, whose percentages were between 1.3 and 1.5%. Similarly, Aragón et al. (2012) found that the PIG in its collection SS-551 of the Tuxpeño breed was 1.31%, being very similar to the PIG of the ‘C0’ genotype (1.33%), which is the original material of the same breed. The percentage of PIG of the genotypes ‘C0’ and ‘C19’ were stable through the study environments, all genotypes had PIG values lower than 2% demanded as maximum by the IHN.
The percentage of grain pericarp (PEG) was highly significant (p≤ 0.01) among G, A, IG*A, and in the mean square of CP1 and CP2, which accounted for 74.99% and 25.01% of the variation, respectively (Table 2). Figure 1e shows the general averages of the PEG that recorded the genotypes, which were, from lowest to highest: ‘C14’ (6.07%), ‘C0’ (6.13%) and ‘C19’ (6.36%), being the most Stable ‘C0’, whereas ‘C19’ and ‘C19’ were unstable; that is, showed higher IG*A, but the genotype ‘C14’ recorded the lowest overall mean. Among localities, the ones that discriminated best the genotypes were T 2013-PV and T 2014-OI, since ‘V-520C’ and its selection compounds originated in Veracruz. T 2014-PV was the most stable environment for PEG and where the grains with the lowest percentage (5.93%) were produced. Meanwhile, T 2014-OI, an inverse behavior was observed, with the highest percentage of PEG (6.58%) and greater instability (Figure 1e). Results that were higher than those reported by Salinas et al. (2013) and Aragón et al. (2012) who reported values between 5.4% and 4.8% in the Tuxpeño breed.
These differences in the percentage often depend on the type of maize and its nutritional quality and yield (Aragon et al., 2012). Taking into account the percentage of ‘C19’, and given that this was higher than the lower cycles, it is inferred that SMV affected that variable; this could happen indirectly when SMV was performed, where the selection criterion was the “year-by-year” grain yield, since no increase in the percentage of PER associated to the selection cycle was observed (Figure 1e). All study sites and evaluated genotypes recorded higher percentages of pericarp than those demanded by the IHN, which requires materials with values ranging from 4.5% to 5.5%.
For the germ percentage (GEG) the first two components (CP1 and CP2) explained 100% of the variability (Table 2). It was observed a marked division between the localities of the Estado de México and Veracruz, leaving the genotypes in the middle of such division (Figure 2f). The most stable genotype for GEG was ‘C19’, with a mean of 10.60%, continuing ‘C14’, with 10.43%, and followed by ‘C0’, with 10.31%, which was the least stable genotype. In the localities C 2014-PV and T 2013-PV, the greatest discrimination between genotypes for GEG was observed, while the most stable environment for IG*A was T 2014-OI, which could be favored by the availability of water during the development and reproduction of the crop. The genotypes produced in Valles Altos had a lower percentage of GEG: M 2014-PV (9.06%, the lowest), M 2013-PV (9.44%) and C 2014-PV (9.81%), while Veracruz had higher means: T 2014-OI (11.31%), T 2014-PV (11.37%) and T 2013-PV (11.68%, the highest).
In Estado de México, where there was a better distribution of precipitation, a lower temperature and a higher dose of nitrogen (Table 1 and Figure 2a), the genotypes yielded more and its grains were harder, but with reduced germ size. Contrary with what was observed in Tepetates, which and according to Tanaka and Yamaguchi (1972) may be due to the higher temperature in Tepetates, Veracruz, during the development and reproduction of the crop. The germ results for the three environments in Tepetates (x= 11.4%) were similar to those reported by Salinas et al. (2013) for Tuxpeño breed varieties grown in Veracruz, on the other hand, Zepeda et al. (2009) reported GEG percentages between 8.7 and 11 in hybrid maize evaluated in Valles Altos, aspects that are in line with the results of this paper. GEG and PIG percentages were significantly correlated (r= 0.51, p≤ 0.01), indicating that the genotypes with more germ also had higher pedicel percentages. In the germination percentage, all the genotypes, in all the localities, fulfilled the requirements of the IHN (≤ 12%).
Despite the environment, REN, PH, PEG and GEG of the genotypes increased in parallel with the progress of the SMV, which may have happened during selection for the year-to-year adaptation, where allele frequencies made favorable changes for such characteristics indirectly, similar results were found by De Jesús et al. (1990) and Pérez et al. (2000) with Tuxpeño Crema 1 evaluated only in Valles Altos of México, where SMV produced significant changes in plant and cob attributes between cycles C2-C11 and C2-C12, respectively. While the IF decreased by the same adaptation effect while PIG remained possibly it is a characteristic less affected by the environment, results also observed by Zepeda et al. (2009); Salinas et al. (2010) for both characteristics.
Conclusions
The agroclimatic conditions of Montecillo, Estado de Mexico, were favorable for the yield improvement and industrial quality of Tuxpeño maize with 19 cycles (‘C19’) of visual mass selection. With regard to ‘C0’ the yield of ‘C19’ increased 45.7% and the flotation index decreased 54.2%, in addition ‘C19’ was the most stable for hectoliter weight and percentage of grain germ, obtaining a yield of 3.5 t ha-1, flotation index of 33 (corresponding to hard grains), test weight of 74.8 kg hL-1, pericarp percentage of 6.36% and germ percentage 10.6%. The evaluated characteristics complied with the established by the standard NMX-034 (1), for maize destined to the traditional nixtamalization process. Therefore, it is inferred that the ‘C19’ genotype with the highest visual mass selection of the Tuxpeño ‘V-520C’ maize adapted to Valles Altos of México contributed to increase yield and to obtaining a quality grain for the dough and the tortilla industry.
Literatura citada
AACC (American Association of Cereal Chemists). 2016. Approved Methods of Analysis. 11th Ed. AACC. The Association. St. Paul, MN. USA. [ Links ]
Abdullah, A. and Barnawi, A. 2012. Identification of the type of agriculture suited for application of wireless sensor networks. Rus. J. Agric. Socio. Econ. Sci. 12(2):19-36. [ Links ]
Agame, A. E.; Juárez, G. E.; Evangelista, L. S.; Rosales, R. O. L. and Bello, P. L. A. 2013. Characteristics of maize starch and relationship with its biosynthesis enzymes. Agrociencia. 47:1-12. [ Links ]
Aragón, C. F.; Figueroa, C. J. de D.; Flores, Z. M.; Gaytán, M. M. y Vélez, M. J. J. 2012. Calidad industrial de maíces nativos de la Sierra Sur de Oaxaca. INIFAP. Oaxaca México., Libro técnico núm. 15. 249 p. [ Links ]
Barrales, D. S.; Muñoz, O. A. y Sotres, R. D. 1984. Relaciones termopluviométricas en familias de maíz bajo condiciones de temporal. Agrociencia. 58:127-139. [ Links ]
Bellon, M. R.; Adato, M.; Becerril, J. and Mindek, D. 2005. Impact of improved germplasm on poverty alleviation: the case of Tuxpeño-derived materials in México. CIMMYT. D. F., México. 58 p. [ Links ]
Castillo, G. F. 1993. La variabilidad genética y el mejoramiento de los cultivos. Ciencia. 44:69-79. [ Links ]
Crossa, J. 1990. Statistical analysis of multilocation trials. Adv. Agron. 44:55-85. [ Links ]
De Jesús, M. A.; Molina, G. J. D. y Castillo, G. F. 1990. Selección masal para la adaptación en Chapingo de una población de maíz Tuxpeño. Agrociencia. 1(4):64-84. [ Links ]
Fasahat, P.; Muhammad, K.; Abdullah, A.; Rahman, B. M. A.; Siing, N. M.; Gauch, J. H. G. and Ratnam, W. 2014. Genotype*environment assessment for grain quality traits in rice. Communications in Biometry and Crop Science. 9(2):71-82. [ Links ]
Figueroa, C. J. D.; Acero, G. M. G.; Vasco, M. N. L.; Lozano, G. A.; Flores, A. L. M. y González, H. J. 2001. Fortificación y evaluación de tortillas de nixtamal. Arch. Latinoam. Nutr. 51(3):293-302. [ Links ]
García, E. 1973. Modificaciones al sistema de clasificación climática de Köppen. Instituto de Geografía (2da edición). Universidad Nacional Autónoma de México (UNAM). D. F., México. 246 p. [ Links ]
Gauch, H. G. and Zobel, R. W. 1989. Accuracy and selection success in yield trial analyses. Theoretical and Applied Genetics. 77:473-481. [ Links ]
González, H. A.; Sahagún, C. J.; Vázquez, G. L. M.; Rodríguez, P. J. E.; Pérez, L. D. J.; Domínguez, L. A.; Franco, M. O. y Balbuena, M. A. 2009. Identificación de variedades de maíz sobresalientes considerando el modelo AMMI y los índices de Eskridge. Agric. Téc. Méx. 35(2):189-200. [ Links ]
Hristov, N.; Mladenov, N.; Djuric, V.; Kondic, S. A.; Marjanovic, J. A. and Simic, D. 2010. Genotype by environment interac tions in wheat quality breeding programs in southeast Europe. Euphytica. 174:315-324. [ Links ]
Martínez, G. A. 1988. Diseños experimentales. Métodos y elementos de teoría. Trillas. México. 756 p. [ Links ]
Molina, G. J. D. 1983. Selección masal visual estratificada en maíz. Centro de Genética, Colegio de Postgraduados. Chapingo. Estado de México. México. 36 p. [ Links ]
Pérez, C. A. A.; Molina, G. J. D. y Martínez, G. A. 2000. Adaptación a clima templado de una variedad de maíz tropical mediante selección masal visual estratificada. Agrociencia. 34:533-542. [ Links ]
SIAP. 2015. Servicio de Información Agroalimentaria y Pesquera de la Secretaría de Agricultura Ganadería, Desarrollo Rural, Pesca y Alimentación. México. Cierre de la producción agrícola por cultivo. http://infosiap.siap.gob.mx/aagricola_siap_gb/ientidad/index.jsp. [ Links ]
Pérez, C. A.; Molina, G. J. D. y Martínez, G. A. 2002. Adaptación clima templado de razas tropicales y subtropicales de maíz de México por selección masal visual. Rendimiento, altura de planta y precocidad. Rev. Fitotec. Mex. 25(4):435-441. [ Links ]
Pérez, C. A.; Molina, G. J. D.; Martínez, G. A.; García, M. P. y Reyes, L. D. 2007. Selección masal para la adaptación a clima templado de razas tropicales y sub-tropicales de maíz de México. Bioagro. 19(3):133-141. [ Links ]
Rincón, T. J. A.; Castro, N. S.; López, S. J. A.; Huerta, A. J.; Trejo, L. C. y Briones, E. F. 2006. Temperatura alta y estrés hídrico durante la floración en poblaciones de maíz tropical. Bot. Exp. 75:31-40. [ Links ]
Salinas, M. Y. y Vázquez, C. G. 2006. Metodologías de análisis de la calidad nixtamalero-tortillera en maíz. Instituto Nacional de Investigaciones Forestales, Agrícolas y Pecuarias (INIFAP). Campo Experimental Valle de México. Chapingo, Estado de México. Folleto técnico núm. 23. México. 91 p. [ Links ]
Salinas, M. Y.; Gómez, M. N. O.; Cervantes, M. J. E.; Sierra, M. M.; Palafox, C. A.; Betanzos, M. E. y Coutiño, E. B. 2010. Calidad nixtamalera y tortillera en maíces del trópico húmedo y sub-húmedo de México. Rev. Mex. Cienc. Agríc. 1(4):509-523. [ Links ]
Salinas, M. Y.; Aragón, C. F.; Ybarra, M. C.; Aguilar, V. J.; Altunar, L. B. y Sosa, M. E. 2013. Caracterización física y composición química de razas de maíz de grano azul/morado de las regiones tropicales y subtropicales de Oaxaca. Rev. Fitotec. Mex. 36(1):23-31. [ Links ]
SE (Secretaría de Economía). 2002. Norma mexicana para maíces destinados al proceso de nixtamalización, NMX-FF-034/1-2002-SCFI-2002. Productos alimenticios no industrializados para consumo humano cereales maíz blanco para proceso alcalino para tortillas de maíz y productos de maíz nixtamalizado-especificaciones y métodos de prueba. Especificaciones y métodos de prueba. Secretaría de Economía (SE). Dirección General de Normas. México, D. F. 18 p. [ Links ]
SE (Secretaria de economía). 2012. Análisis de la cadena de valor de la tortilla de maíz: estado actual y factores de competencia local. SE-Departamento General de Industrias Básicas. Disponible en: http://www.economia.gob.mx/files/comunidadnegocios/industria_comercio/informacionsectorial/20120411_analisis_cadena_valor_maiz_tortilla.pdf. [ Links ]
Statistical Analysis System (SAS). 2002. The SAS System for Windows 9.0. User’s guide. Cary, N. C. USA. 584 p. [ Links ]
Tanaka, A. and Yamaguchi, J. 1972. Dry matter production, yield components and grain yield of the maize plant. J. Fac. Agric. 57(1):71-132. [ Links ]
Torres, F. J. L.; Morales, R. E. J.; González, H. A.; Laguna, C. A. y Córdova, O. H. 2011. Respuesta de híbridos trilineales y probadores de maíz en valles altos del centro de México. Rev. Mex. Cienc. Agríc. 2(6):829-844. [ Links ]
Vázquez, C. G.; García, L. S.; Salinas, M. Y.; Bergvinson, D. J. and Palacios, R. N. 2011. Grain and tortilla quality in landraces and improved maize grown in the highlands of Mexico. Plant Foods for Human Nutrition. 66:203-208. [ Links ]
Vázquez, C. M. G.; Santiago, R. D.; Salinas, M. Y.; Rojas, M. I.; Arellano, V. J. L.; Velázquez, C. G. A. y Espinosa, C. A. 2012. Interacción genotipo-ambiente del rendimiento y calidad de grano y tortilla de híbridos de maíz en Valles Altos de Tlaxcala, México. Rev. Fitotec. Mex. 35(3):229-237. [ Links ]
Vázquez, C. M. G.; Arellano, V. J. L. y Santiago, R. D. 2015. Rendimiento y calidad de grano y tortilla de maíces híbridos de Valles Altos de México crecidos en riego y temporal. Rev. Fitotec. Mex. 38(1):75-83. [ Links ]
Wen, W.; Franco, J.; Chávez, T. V. H; Yan, J. and Taba, S. 2012. Genetic characterization of a core set of a tropical maize race Tuxpeño for further use in maize improvement. PLoS ONE. 7(3):e32626. [ Links ]
Zepeda, B. R.; Carballo, C. A. y Hernández, A. C. 2009. Interacción genotipo-ambiente en la estructura y calidad del nixtamal-tortilla del grano en híbridos de maíz. Agrociencia. 43:695-706. [ Links ]
Zobel, W. R.; Wright, M. J. and Gauch, J. H. G. 1988. Statistical analysis of a yield trial. Agron. J. 80(3):388-393.2 [ Links ]
Received: March 2017; Accepted: June 2017