Introduction
Nowadays, the valorization of aromatic and medicinal plants is having more and more interest. Indeed, these plants are resource of many active compounds essentially secondary metabolites, characterized by biological properties as antioxidant, antimicrobial, anti-inflammatory, and antitumor activities. In order to preserve health and to avoid the undesirable effects of chemical drugs and products, medicinal plants are increasingly used in modern medicine, in cosmetic and pharmaceutical industry [1]. Several studies revealed that the variability of medicinal plants and their proprieties are related to the geographical parameters. In fact, species growing under diverse environmental conditions and bioclimatic areas show significant variances in both the production and the accumulation of primary and secondary metabolites [2,3]. Influenced by environmental factors, many groups of secondary metabolites serve as a chemical interface between the plant and its environment. In fact, this chemical interaction is basically mediated by the biosynthesis of secondary metabolites, which exert their biological roles. Such chemical interactions frequently consist of variations in the production of plant metabolites [3,4]. For this reason, the study of these variations is very important in the chemical characterization of plants that belong to the same species and have been collected from diverse regions and this is when the different geographical origins of a plant material is taken into account [5,6]. Some factors can be the essential sources of distinction of metabolites for plant species. These characteristics involve long term acclimation or local adaptation, seasonal differences related to phenology or environmental changes in the biotic and abiotic factors, geographical differences, or different environmental conditions of the growth location of species [7,8].
Artemisia is one of the largest genera of the family Asteraceae. Artemisia species are essentially dispersed in temperate regions, occupying arid and semiarid environmental zone. On account of the considerable number of species, ecological and economic values, they have been the subject of numerous reviews. Many species have been applied as traditional remedies for some therapeutics. Previous researches revealed that a large number of Artemisia species contain many classes of secondary metabolites as phenolic acids, flavonoids, coumarins, sterols and terpenoids [9,10].
Artemisia absinthium L. commonly known as wormwood, is a medicinal and aromatic plant belonging to the Asteraceae family and the Artemisia genus. The aerial parts of A. absinthium possess an extended history of use in folk medicine. It is a wild growing plant in Europe, North America, Asia and widely dispersed in north of Iran [11]. In previous studies, it has been reported that A. absinthium possesses many pharmacological properties, mainly antibacterial [12], anti-parasitic [13], antiplasmodial [14], antidiabetic [15], hepatoprotective [16], and neuroprotective [17] properties. Wormwood is also known by enhancing the cognitive ability as evidenced by its nicotinic and muscarinic receptor activity in homogenates of human cerebral cortical membranes [18]. It is worth to note that the ethnopharmacological literature has supported the use of A. absinthium for muscle complications, antiseptic activity and relieving the signs of depression [19]. The ointment of A. absinthium can be used to reduce muscle and joint stiffness or pain and can also help heal bruises. The results of a study conducted by Benkhaled et al., [20] showed that the ointment with 10% of A. absinthium essential oil (EO) enhances the skin wound healing process. Further, the methanolic extract of A. absinthium has been reported to protect the liver against chemical toxins [21,22]. Also, the extracts of this medicinal plant have demonstrated a strong antiradical and antioxidant activity. Free radical scavenging activity of A. absinthium extracts have been also described in both in vitro and in vivo studies [23-25]. Several reviews have described the composition and the biological proprieties of A. absinthium EO and extracts. A. absinthium L. is used within the gastrointestinal treatment in the traditional Persian medicine [26]. This gastroprotective effect is evaluated also by Kaoudoune et al. [27]. In fact, they found that the antiulcer and antioxidant properties of A. absinthium aqueous extract were promising.
Previous studies have point out that the stimulant property of wormwood is initiated by bioactive substances such as artabsin (sesquiterpene lactone) and absinthin (dimer of sesquiterpene lactone) present in plant extracts [11]. In fact, the EO extracted from Iranian wormwood was characterized by the predominance of β-pinene and β-thujone [28]. Also, from the same perspective, the EO of Artemisia a. growing in Morocco is principally constituted by α-thujone (39.69%), sabinyl acetate (10.96%), and β-thujone (7.25%) [29]. Besides, research conducted by Martín et al. [30] indicated that Z-epoxyocimene, chrysanthenol, and chrysanthenyl acetate were the dominant compounds of wormwood found in the supercritical fluid extracts (SFE) similarly in the hydrodistilled EOs.
The purpose of this study was to underline the variability of wormwood EO and methanolic extracts and their biological activities as affected by the collection site.
Experimental
Materials and methods
Chemical reagents
All solvents used in the experiments were purchased from Merck (Darmstadt, Germany). Folin-Ciocalteu reagent, sodium hydroxide de (NaOH), sodium carbonate anhydrous (Na2CO3), sodium nitrite (NaNO2), disodium hydrogen phosphate (Na2HPO4), sodium monobasic phosphate anhydrous (NaH2PO4H2O), aluminum chloride hexahydrate (AlCl3, 6H2O), 2,2-diphenyl-1-picrylhydrazyl (DPPH), trichloroacetic acid (TCA), iron (III) chloride (FeCl3), potassium ferricyanide (K3Fe (CN)6) and butylated hydroxytoluene (BHT) were purchased from Sigma-Aldrich (Steinheim, Germany). Sulfuric acid (H2SO4) and Mueller Hinton broth were purchased from Merck (Darmstadt, Germany). Hydrochloric acid (HCl 37%) was provided from VWR chemicals (France). Deionized water was used to prepare all solutions and these solutions were stored at 4 °C.
Plant sampling
Aerial parts of Artemisia absinthium were randomly collected in February 2017 from five different regions of Tunisia (Bizerte, Zaghouan, Kasserine, Gabes and Tozeur) (Table 1) and identified by Professor Abderrazek Smaoui (Borj-Cedria Biotechnology Center, Tunisia). The choice of these sites was based on the geographical differences of the studied species. A voucher specimen (Aa 2540-17) was deposited in the herbarium of the Laboratory of Aromatic and Medicinal Plants in the Center of Biotechnology in Borj Cedria. The plant material was air-dried for two weeks at room temperature (20-25 °C) and then ground to fine powder by an electric mill and conserved at room temperature (∼25 °C) in darkness for different uses.
Table 1 Geographical and bioclimatic collection sites parameters.
Region | Longitude | Latitude | Elevation (m) | Bioclimatic floor |
Bizerte | 9°51’57.31’’E | 37°16’01.57’’ N | 7 | Humid |
Zaghouan | 10°08’59.79’’E | 36°24’01.90’’N | 184 | Semi-arid medium |
Kasserine | 8°49’57.16’’E | 35°10’00.59’’N | 709 | Lower semi-arid |
Gabes | 10°06’26.20’’E | 33°51’50.81’’N | 21 | Lower arid |
Tozeur | 8°07’59.39’’ E | 33°55’02.44’’N | 47 | Saharan |
E: East, N: North
Essential oil extraction
One hundred gram of aerial parts (leaves and stems) of A. Absintium was subjected to hydrodistillation for 3h with 750 mL distilled water using a Clevenger-type apparatus. The obtained EO was collected and dried over anhydrous sodium sulfate and stored in sealed glass vials in a refrigerator at 4 °C prior to analysis. Yield based on dried weight of the sample was calculated as well as the retention index (IR).
Chromatographic analyses of essential oil
The gas chromatography analysis of the volatile oil was performed using a HP 6890-series equipped with Flame ionization detectors (FID), HP-5 (BP-1) (5 % phenyl + 95 % dimethylpolysiloxane) 30 m × 0.25 mm ID, 0.25 μm film thickness fused capillary column. The carrier gas was nitrogen (1/min). The oven temperature program was 5 min isothermal at 50 °C, then 50-280 °C at rate of 5 °C/min and held isothermal at 280 °C for 1 min. The injection port and the detector temperature were 250 °C and 300 °C, respectively. One micro-liter of 1 % solution (diluted in hexane) was injected.
Gas chromatography-mass spectrometry
The analysis of the volatile constituents was run on a Hewlett-Packard GC-MS system (GC: 5890-series II; Mass Spectrometer: 5972). The fused-silica HP-5 MS capillary column (30 m × 0.25 mm ID, film thickness of 0.25 μm) was directly coupled to the MS. The carrier gas was the helium at a flow rate of 1 mL/min. Oven temperature was programmed (80 °C for 1.2 min, then 80-175 °C at rate of 30 °C/min and 175-225 °C at rate of 45 °C/min) and subsequently, held isothermal at 275°C for 10 min. Injector port: 250 °C, detector: 280 °C, split ratio 60:1. Volume injected: 1 μL of 1 % solution (diluted in hexane): HP 5972 recording at 70 eV; scan time and mass range were 1s and 50-550 m/z, respectively. The components of the EO were identified by comparison of their mass spectra with those in the Wiley 275 GC-MS library and those in the literature [31], as well as by comparison of their retention indices with literature data [32]. Retention indices of the recognized compounds were determined relative to the retention times of a series of C9-C28 n-alkanes on the HP5 -MS column. The GC/MS analysis was performed in triplicate.
Extract preparation
Extracts were obtained by magnetic stirring of 3 g dry powder in 30 mL methanol (80 % (v/v)) for 30 min as described by Mau et al. [33]. The mixtures were kept for 24 h at 4 °C and filtered through Whatman No. 4 filter paper. Extracts were stored in dark bottles at 4 °C until analysis.
Colorimetric quantification of antioxidants
Total phenolic content
Colorimetric quantification of total polyphenols of aerial parts of A. absinthium was determined, as described by Dewanto et al. [34]. An aliquot (0.125 mL) of appropriately diluted sample extract was mixed with 0.5 mL distilled water and 0.125 mL of Folin-Ciocalteu reagent. After 3 min, 1.25 mL of Na2CO3 solution (7%) were added and the final volume was made up to 3 mL with distilled water. The absorbance of the resulting solution was measured at 760 nm, after incubation for 90 min. The phenols contents were expressed in terms of milligrams gallic acid equivalent per gram of dry residue (mg GAE/g DW). Triplicate measurements were taken for all samples.
Total flavonoid content
Total flavonoids contents were measured according to Dewanto et al. [34]. An aliquot of suitable diluted samples was added to 0.075 mL of NaNO2 and mixed for 6 min, before adding 0.15 mL of a freshly prepared AlCl3 (10 %). After 5 min, 0.5 mL of NaOH (1 M) solution was added. The final volume was adjusted to 2.5 mL with distilled water and thoroughly mixed. Absorbance of the mixture was measured at 510 nm. Total flavonoid content was expressed as mg quercetin equivalent per gram of dry weight (mg QE/g DW). All samples were analyzed in triplicate.
Total condensed tannin content
The analysis of condensed tannins was realized according to the protocol of Sun et al. [35]. Three milliliters of 4 % methanolic vanillin solution and 1.5 mL of concentrated HCl (37 %) were added to 0.05 mL of suitably diluted sample. After 15 min of incubation, the absorbance was measured at 500 nm. The amount of total condensed tannins was expressed as mg of catechin equivalent per g dry weight (mg of CE/g of DW).
RP-HPLC analysis of phenolic compounds
The separation of phenolics was performed with an Agilent 1260 series RP-HPLC system equipped with an online degasser (G 1322A), quaternary pump (G 1311A), a thermostatic autosampler (G 1313A), column heater (G 1316A), and diode array detector (G 1315A). Instrument control and data analysis was carried out using Agilent HPLC Chemstation 10.1 editon through Windows 2000. The separation was carried out on a reverse phase ODS C18 column (4.6 mm, 250 x 4.6 mm, Hypersil) used as stationary phase at ambient temperature. The mobile phase consisted of methanol as solvent A and water with sulfuric acid as solvent B (0.1 mL/100 mL water). The flow rate was kept at 0.4 mL/min. The gradient program was as follows: 10-20 % A/90-80 % B 0-5 min, 20-30 % A/80-70 % B 5-10 min, 30-50 % A/ 70-50 % B 10-15 min, 50-70 % A/50-30 % B15-20 min, 70-90 % A/30-10 % B 20-25 min, 90 % A/10 % B 25-30 min, and 100 % A 30-35 min. The injection volume was 20 µL and peaks were monitored at 280 nm. Peak identification was obtained by comparing the retention time and the UV spectra of the fraction phenolics chromatogram with those of pure standards in the same operating conditions which were purchased from Sigma (St. Louis, MO, USA).
Assessment of antioxidant activities
Evaluation of total antioxidant capacity
Total antioxidant capacity based on the reduction of Mo (VI) to Mo (V) by the extract. An aliquot (0.1 mL) of fractions extracts was combined to 1 mL of reagent solution (0.6 M sulfuric acid (H2SO4), 28 mM sodium phosphate (NaH2PO4) and 4 mM ammonium heptamolybdate [(NH4)6MO7O24, 4H2O)]. The tubes were incubated in a thermal block at 95 °C for 90 min before measurement at 695 nm versus a blank [36]. The antioxidant capacity was expressed as mg gallic acid equivalent per gram dry weight (mg GAE/g DW). Triplicate measurements were taken for all samples.
DPPH radical-scavenging activity
The free radical scavenging activity (RSA) of the extracts was measured using the DPPH (2,2-diphenyl-1-picrylhydrazyl) method based on measurement of hydrogen donating or radical-scavenging ability using the stable DPPH method [37]. One mL of the methanolic extract solution with different concentrations (25, 50, 1000 and 250 μg/ml) was mixed with 0.25 mL of methanolic solution of DPPH (0.2 mmol/L) and allowed to react in the dark for 30 min. The antiradical activity was expressed as IC50 (µg/mL), the extract dose required to cause a 50 % inhibition. Radical-scavenging activity (RSA) was estimated as RSA % = [(A0 - A1)/A0] x100, where A0 is the absorbance of the control reaction and A1 is the absorbance of the test extract.
Determination of reducing power
The ferric reducing antioxidant power assay was evaluated by the method of Oyaizu [38]. Briefly, 1 mL of the methanolic extract solution with different concentrations (250, 500, 1000 and 1500 μg/mL) was mixed with 2.5 mL of sodium phosphate buffer (0.2 mol/L, pH 6.6) and 2.5 mL of 1% potassium ferricyanide (K3Fe(CN)6). After incubation at 50 °C for 20 min, 2.5 mL of 10 % trichloroacetic acid (TCA) were added. After a rest of 10 min at room temperature, the upper layer (2.5 mL) was mixed with distilled water (2.5 mL) and 0.1% ferric chloride (FeCl3) (0.5 mL). The absorbance was read at 700 nm. A higher absorbance indicates a higher reducing power. The results are expressed in EC50 (mg/mL) which is the effective concentration giving an absorbance of 0.5 for reducing power. The EC50 value is obtained by interpolation of the linear regression line.
Antibacterial activities
The antibacterial activity was assessed by the agar disk diffusion assay as described by Bagamboula et al. [39] against six human pathogenic bacteria strain: Gram-positive bacteria including Bacillus licheniformis (ATCC 8480), Enterococcus hirae (ATCC 10541), Staphylococcus aureus (ATCC 6538) and Gram-negative bacteria including Serratia marcescens (ATCC 13880), Pseudomonas aeruginosa (ATCC27853) and Echerichia coli (ATCC 8739). The bacterial strains were first grown on Muller Hinton medium at 37 °C for 24 h prior to seeding onto the nutrient agar. One or several colonies of the indicator bacteria were transferred into API suspension medium (BioMérieux) and adjusted to the 0.5 McFarland turbidity standard with a Densimat (BioMérieux). Sterile filter paper discs (6 mm in diameter) were placed on the cultured plates and 10 µL of wormwood EOs were dropped per disc. Positive control discs of streptomycin were included in each assay (1 mg/mL). The antibacterial activity was assessed by the appearance or not of an inhibition zone of growth around the discs after 24 h of incubation at 37 °C. Three replicates were conducted for each test.
Statistical analysis
Data were expressed as mean ± standard error. The means were compared using the one-way and multivariate analysis of variance (ANOVA) followed by Duncan’s multiple range tests. The differences between individual means were deemed to be significant at P < 0.05. Pearson’s correlation test was conducted to determine the linear correlation among the variables. A hierarchical component analysis (HCA) was performed in order to discriminate between different regions of plant collection on the basis of their EOs and phenolic composition. All analyses were performed by the ‘‘Statistica v 5.1’’ software.
Results and Discussion
Characterization by Elemental and Mass Spectral Studies
Essential oil yield
The hydrodistillation of A. absinthium aerial parts collected from five different regions gave dark blue colored EOs with a strong odor. The obtained yields of EO significantly varied (P < 0.05) depending on the region factor (Fig. 1). The higher EO yields were observed in Gabes (0.61 ± 0.02 %) and Tozeur (0.60 ± 0.05 %) regions, followed by Bizerte (0.45 ± 0.03 %), Zaghouan (0.40 ± 0.04 %) and Kasserine (0.37 ± 0.01 %) ones. The highest EO yield (1.46 %) was determined by Msaada et al. [24] in the case of Tunisian A. absinthium at Bou Salem region. Lopez-Lutz et al. [40] reported that the EO yield of A. absinthium was 0.50 % at the Central prairies of Alberta, West of Canada. However, Turkish A. absinthium EO yielded 0.67 % [41]. These authors demonstrated the effect of the environmental and geographical characteristics of the collection region on the EO yields.
Essential oil composition
The results of GC-MS analysis are presented in Table 2. A total of 35 compounds were identified representing 91.63 ± 8.15 %, 89.22 ± 4.68 %, 95.33 ± 8.45 %, 90.73 ± 7.83 % and 98.21 ± 8.57 % of total volatiles in the regions of Zaghouan, Bizerte, Gabes, Kasserine and Tozeur, respectively. As can be seen from Table 2, these different compounds significantly varied from region to another, and they were highly (P ≤ 0.001) affected by the regional factor. The major contribution was attributed to the oxygenated monoterpene fraction with 57.73 ± 6.35 % in Zaghouan, 51.60 ± 3.59 % in Bizerte, 63.12 ± 5.97 % in Gabes, 52.93 ± 4.28 % in Kasserine and 53.66 ± 5.03 % in Tozeur. Camphor was the main compound with a maximum level (49.70 ± 2.34 %) in Gabes, followed by Kasserine (40.39 ± 1.07 %), Zaghouan (39.51 ± 0.95 %), Tozeur (35.37 ± 0.73 %) and Bizerte (33.63 ± 0.68 %) regions. These results were confirmed by Riahi et al. [25] who reported that camphor was the major component of A. absinthium EOs in Kasserine (33.29 %), Kef (28.94 %) and Ghar Dimaou (50.37 %) regions. Vieira et al. [42] reported that camphor (19 %), (E)-caryophyllene (9.3 %), eucalyptol (6.8 %), germacrene D (6.7 %) and α- cadinol (6.5 %) were the major chemical constituents of A. absinthium EO from Brazil. Several studies mentioned that α-thujone and β-thujone were the major compounds in A. absinthium EOs from Greece, Spain, Ukraine, France, and Italy with percentages ranging from 4.5 to 38.7 % [43]. A study done in Canada showed that the main components of A. absinthium EO were trans-sabinyl acetate (26.4 %), myrcene (10.8 %) and trans-thujone (10.1 %) [40].
Table 2 Essential oil composition (%, w/w) of Artemisia absinthium L. collected from different regions of Tunisia.
Compound† | RRI | Collection region | P | ||||
---|---|---|---|---|---|---|---|
Zaghouan | Bizerte | Gabes | Kasserine | Tozeur | |||
α-Thujene | 930 | 0.19 ± 0.02a | 0.12 ± 0.01c | 0.12 ± 0.01c | 0.15 ± 0.01b | tr | 0.000*** |
α-Pinene | 940 | 2.12 ± 0.09a | 1.33 ± 0.06c | 0.88 ± 0.03e | 1.54 ± 0.05b | 1.05 ± 0.11d | 0.000*** |
α-Fenchene | 941 | tr | tr | tr | 0.53 ± 0.02 | tr | 0.000*** |
Sabinene | 973 | 0.06 ± 0.01 | nd | nd | nd | tr | 0.000*** |
Myrcene | 987 | 0.05 ± 0.01 | tr | tr | tr | tr | 0.623NS |
α-Phellandrene | 1002 | 0.30 ± 0.02a | 0.07 ± 0.01d | 0.13 ± 0.01c | 0.18 ± 0.01b | tr | 0.000*** |
Δ-3-Carene | 1010 | 0.21 ± 0.02b | 0.14 ± 0.01c | nd | 1.01 ± 0.01a | tr | 0.453NS |
α-Terpinene | 1013 | 0.84 ± 0.02a | 0.63 ± 0.03b | 0.28 ± 0.02e | 0.59 ± 0.09c | 0.38 ± 0.05d | 0.022* |
p-Cymene | 1015 | 0.58 ± 0.02a | 0.09 ± 0.01e | 0.43 ± 0.04b | 0.28 ± 0.01d | 0.37 ± 0.03c | 0.000*** |
1,8 Cineole | 1024 | 0.02 ± 0.00 | tr | tr | tr | tr | 0.625NS |
Limonene | 1025 | 0.82 ± 0.09a | 0.62 ± 0.06b | 0.50 ± 0.02d | 0.53 ± 0.03c | 0.37 ± 0.04e | 0.000*** |
Camphene | 1050 | 3.04 ± 0.12a | 1.91 ± 0.09c | 1.21 ± 0.03e | 1.97 ± 0.06b | 1.37 ± 0.14d | 0.000*** |
γ-Terpinene | 1051 | 2.06 ± 0.03a | 1.24 ± 0.05d | 1.05 ± 0.05e | 1.58 ± 0.04b | 1.30 ± 0.10c | 0.000*** |
trans-Sabinene hydrate | 1053 | 3.59 ± 0.09d | 3.43 ± 0.05e | 7.61 ± 0.07a | 6.40 ± 0.11b | 5.09 ± 0.06c | 0.000*** |
cis Sabinene hydrate | 1082 | nd | nd | 0.57 ± 0.07a | 0.48 ± 0.03b | 0.31 ± 0.02c | 0.000*** |
α-Terpinolene | 1082 | 0.28 ± 0.02c | 0.06 ± 0.01d | tr | 0.31 ± 0.02b | 0.62 ± 0.07a | 0.377NS |
Linalool | 1086 | 2.72 ± 0.05b | 3.82 ± 0.10a | 2.07 ± 0.16c | 1.62 ± 0.08e | 1.69 ± 0.01d | 0.000*** |
Camphor | 1123 | 39.51 ± 0.95c | 33.63 ± 0.68e | 49.70 ± 2.34a | 40.39 ± 1.07b | 35.37 ± 0.73d | 0.000*** |
Terpinene-4-ol | 1176 | 3.34 ± 0.07c | 3.31 ± 0.02c | 3.06 ± 0.11d | 3.68 ± 0.09b | 3.78 ± 0.08a | 0.000*** |
α-Terpineol | 1176 | 0.11 ± 0.21c | 0.28 ± 0.02b | 0.37 ± 0.07a | tr | tr | 0.003** |
α-Fenchyl acetate | 1205 | 7.82 ± 0.26b | 6.87 ± 0.04d | 7.92 ± 0.24a | tr | 6.92 ± 0.18c | 0.000*** |
Linalyl acetate | 1239 | 0.02 ± 0.00 | nd | nd | nd | nd | 0.624NS |
Bornyl acetate | 1270 | nd | nd | nd | 5.33 ± 0.10b | 5.80 ± 0.68a | 0.000*** |
Neryl acetate | 1342 | 0.60 ± 0.02a | 0.26 ± 0.04d | tr | 0.36 ± 0.05c | 0.50 ± 0.03b | 0.316 |
Geranyl acetate | 1362 | 0.33 ± 0.07b | 0.04 ± 0.01c | tr | 0.32 ± 0.02b | 0.66 ± 0.08a | 0.027* |
β-Copaene | 1430 | 0.58 ± 0.04 | nd | nd | nd | nd | 0.624NS |
α-Humulene | 1455 | 0.10 ± 0.01 | nd | nd | nd | nd | 0.170NS |
α-Farnesene | 1460 | 0.03 ± 0.00b | nd | nd | 0.10 ± 0.01a | nd | 0.003** |
𝛾-Curcumene | 1473 | 0.75 ± 0.06e | 1.09 ± 0.15c | 1.24 ± 0.06a | 0.78 ± 0.03d | 1.16 ± 0.03b | 0.965NS |
Germacrene D | 1476 | 1.12 ± 0.10e | 2.17 ± 0.10a | 1.46 ± 0.07d | 1.93 ± 0.03c | 2.13 ± 0.10b | 0.123NS |
α-Calacorene | 1527 | 0.08 ± 0.01b | tr | tr | tr | 0.23 ± 0.03a | 0.012* |
Trans-Caryophyllene | 1578 | 0.73 ± 0.05a | 0.18 ± 0. 07e | 0.61 ± 0.08c | 0.70 ± 0.05b | 0.59 ± 0.03d | 0.000*** |
dihydro Cadalene | 1659 | 2.66 ± 0.16c | 4.15 ± 0.08a | 2.20 ±0.07d | 3.69 ± 0.19b | 1.64 ± 0.55e | 0.000*** |
Chamazulene | 1719 | 13.78 ± 0.45d | 21.1 ± 0.53b | 12.51 ± 0.92e | 14.15 ± 0.19c | 25.41 ± 0.61a | 0.000*** |
Chemical classes | |||||||
Monoterpene hydrocarbons | 17.36 ± 1.65b | 12.32 ± 1.20d | 14.19 ± 1.25c | 17.88 ± 1.28a | 12.35 ± 1.04d | 0.000*** | |
Oxygenated Monoterpene | 57.73 ± 6.35b | 51.60 ± 3.59d | 63.12 ± 5.97a | 52.93 ± 4.28e | 53.66 ± 5.03c | 0.000*** | |
Sesquiterpene Hydrocarbons | 19.75 ± 1.56c | 28.69 ± 2.04d | 18.02 ± 1.08e | 21.25 ± 2.22b | 30.93 ± 3.19a | 0.000*** | |
Oxygenated sesquiterpene | 0.41 ± 0.03c | 0.04 ± 0.01d | tr | 5.65 ± 0.41b | 6.69 ± 0.59a | 0.000*** | |
Total identified | 91.63 ± 8.15c | 89.22 ± 4.68e | 95.33 ± 8.45b | 90.73 ± 7.83d | 98.21 ± 8.57a | 0.000*** |
RRI: relative retention index. †Compounds in order of elution on HP5-MS; values of volatile essential oil percentages are the average of three determinations (n=3). These values with different letters (a-e) are significantly different at P < 0.05. tr: trace, nd: not detected. ∗∗P< 0.01, ∗∗∗P< 0.001. P: propabolity.
In our research, sesquiterpene hydrocarbons fraction which is represented by β-copaene, α-humulene, ar-curcumene, germacrene-D, trans-caryophyllene, dihydro cadalene and chamazulene ranged from 18.02 ± 1.08 to 30.93 ± 3.19 % of total volatile compounds.
As shown in the Table 2, chamazulene was detected with significant percentages in the regions of Tozeur (25.41 ± 0.61 %) and Gabes (12.51 ± 0.92 %). The dark blue color of A. absinthium essential oil is due to the presence of the sesquiterpenoid molecule chamazulene considered as natural colorant. Chamazulene is biosynthesized by carboxylation of the sesquiterpene matricin [44]. Previous studies have shown that chamazulene was the major component of wormwood EO with a maximum percentage (39.93 ± 4.56 %) in Jérissa region [24].
Among the identified compounds, α-fenchyl acetate and trans-sabinene hydrate were present in considerable levels in the different EOs. Indeed, α-fenchyl acetate had 7.82 ± 0.26 % in Zaghouan, 6.87 ± 0.04 % in Bizerte, 7.92 ± 0.24 % in Gabes and 6.92 ± 0.18 % in Tozeur. For trans sabinene hydrate, the maximum level was observed in Gabes region (7.61 ± 0.07 %).
It should be mentioned that the monoterpene hydrocarbon fraction was represented by considerable percentages having 17.36 ± 1.65% in Zaghouan, 12.32 ± 1.20 % in Bizerte, 14.19 ± 1.25 % in Gabes, 17.88 ± 1.28 % in Kasserine and 12.35 ± 1.04 % in Tozeur, respectively. As shown in Table 2, the oxygenated sesquiterpene fraction was highly affected by the region factor ranging from 0.04 ± 0.01 % in Bizerte to 6.69 ± 0.59 % in Tozeur.
HCA was carried out based on the EO composition to discriminate between the five different collection regions of wormwood (Fig. 2). The dendrogram generated by HCA showed the existence of two main groups. The first group contained only Gabes which was characterized by the highest level of camphor (49.70 ± 2.34 %) and the lowest level of chamazulene (12.51 ± 0.92 %). The second group represented the other regions Bizerte, Kasserine, Tozeur and Zaghouan regions. This group could be divided in three sub-groups. Bizerte and Tozeur were found in the same subgroup having a similar chemical composition. Kasserine and Zaghouan constituted each one a subgroup. In fact, the highest level of chamazulene was detected in Tozeur (25.41 ± 0.61 %) but the lowest level of camphor in Bizerte (33.63 ± 0.68 %).
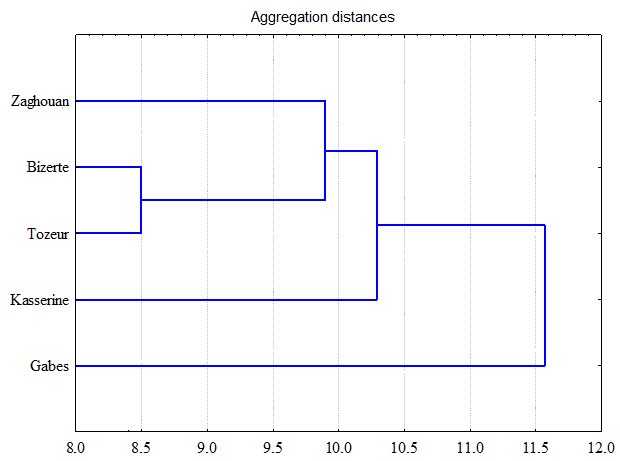
Fig. 2 Two‐dimensional dendrogram obtained by the cluster analysis of different regions based on the essential oil’s composition of Artemisia absinthium
To sum up, A. absinthium collected from the five different Tunisian regions showed an interesting chemical composition where camphor and chamazulene were the most important compounds characterized by many biological activities (anti-inflammatory, antioxidant, antimicrobial activities…).
Total polyphenol, flavonoid and condensed tannin contents
The total polyphenol, flavonoid and condensed tannin contents of wormwood extracts are presented in the Fig. 3. The contents of these components, determined by Folin-Ciocalteu method, significantly varied (P ≤ 0.05) from one region to another. The highest total polyphenol content was observed in Zaghouan (26.80 ± 0.00 mg GAE/g DW), followed by Tozeur (26.53 ± 0.00 mg GAE/g DW), Gabes (20.66 ± 0.00 mg GAE/g DW), Bizerte (20.40 ± 0.00 mg GAE/g DW) and Kasserine (6.93 ± 0.00 mg GAE/g DW) regions. The highest total flavonoid contents was detected in Zaghouan region (6.84 ± 0.02 mg QE/g DW). For total condensed tannins, the maximum content was observed in the region of Tozeur (11.62 ± 0.01 mg CE/g DW). Mahmoudi et al. [45] found that the total polyphenol content of A. absinthium collected from Iran was 194.9 ± 9.7 mg GAE/g DW. In Turkey, the total polyphenol content of the ethanolic extract from A. absinthium was about 33.33 ± 1.48 mg GAE/g [46]. Moreover, Sidaoui et al. [47] reported that the total polyphenol content of Tunisian A. absinthium aerial parts was 11.56 ± 1.20 mg GAE/g. The total flavonoid content of A. absinthium collected from South Korea was 41.21 ± 0.04 mg QE/g DW [48].
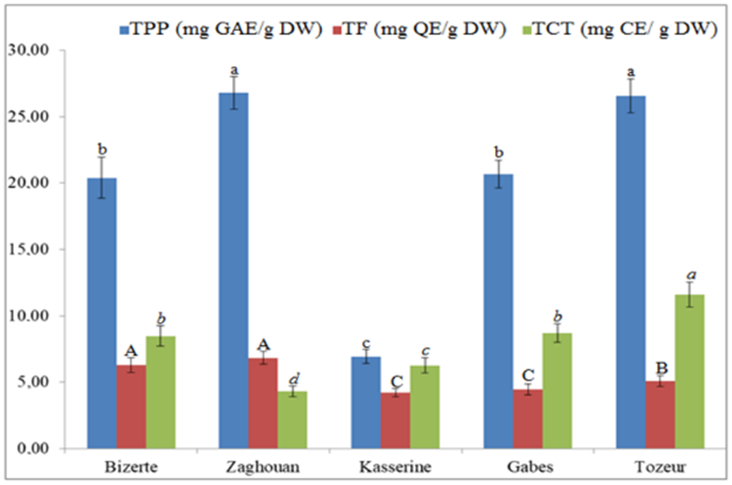
GAE gallic acid equivalents; QE: quercitin equivalents; CE: catechin equivalents. The letters [small letters a-c, small italic letters a-d and capital letters (A-C)] indicate significant differences (P < 0.05)
Fig. 3 Total polyphenols (TPP), total flavonoids (TF), and total condensed tannin (TCT) contents of different regions.
Identification of phenolic compounds by RP-HPLC
The results of RP-HPLC analysis of the five methanolic extracts of A. absinthium are summarized in Table 3. The identified compounds were highly influenced (P < 0,001) by the region factor. Phenolic acids (chlorogenic hemihydrate, caffeic, sinapic, syringic, ferrulic, trans-hydroxycinnamic and ellagic acids) represented 7.22 ± 0.25 % in Bizerte, 9.53 ± 1.11 % in Zaghouan, 4.33 ± 0.73 % in Kasserine, 3.21 ± 0.11 % in Gabes and 9.37 ± 1.95 % in Tozeur.
Table 3 Phenolic composition of the methanolic extracts of wormwood aerial parts.
Compound | Collection region | P | ||||
---|---|---|---|---|---|---|
Bizerte | Zaghouan | Kasserine | Gabes | Tozeur | ||
Epigallocatechin | 0.54 ± 0.04d | 0.59 ± 0.05b | 0.56 ± 0.04c | 0.61 ± 0.05a | nd | 0.017* |
Chlorogenic acid | 0.72 ± 0.06d | 0.79 ± 0.08c | 1.15 ± 0.12b | 0.49 ± 0.05e | 1.27 ± 0.11a | 0.000*** |
Epicatechin 3-O-gallate | 0.25 ± 0.02d | 0.27 ± 0.03c | 0.48 ± 0.04b | tr | 0.63 ± 0.07a | 0.073NS |
Caffeic acid | 0.72 ± 0.06d | 0.79 ± 0.08c | 0.97 ± 0.08a | nd | 0.92 ± 0.08b | 0.000*** |
Syringic acid | 1.14 ± 0.10c | 1.25 ± 0.11b | 1.27 ± 0.13a | 0.51 ± 0.05e | 1.09 ± 0.09d | 0.000*** |
Sinapic acid | 0.94 ± 0.08d | 2.53 ± 0.21a | 0.49 ± 0.05e | 1.22 ± 0.14b | 1.07 ± 0.11c | 0.000*** |
Ferulic acid | 0.40 ± 0.03e | 1.40 ± 0.12b | 0.45 ± 0.03d | 0.99 ± 0.08c | 1.32 ± 0.11a | 0.096NS |
trans hydroxycinnamic acid | 3.30 ± 0.21b | 2.77 ± 0.22c | nd | nd | 3.70 ± 0.34a | 0.000*** |
Coumarin | tr | tr | 3.83 ± 0.35 | tr | tr | 0.000*** |
Isorhamnetin 3-O-glucoside | 2.98 ± 0.25c | 4.57 ± 0.39a | 2.18 ± 0.20d | 0.95 ± 0.08e | 3.32 ± 0.31b | 0.000*** |
Rutin | 7.63 ± 0.88d | 10.29 ± 1.25c | 12.88 ± 1.31a | 1.05 ± 0.11e | 12.00 ± 1.35b | 0.000*** |
Isoquercetin | 0.67 ± 0.05c | 4.93 ± 0.55a | 0.25 ± 0.02d | tr | 0.71 ± 0.06b | 0.398NS |
Ellagic acid | 0.62 ± 0.05a | 0.40 ± 0.03c | nd | nd | 0.56 ± 0.06b | 0.000*** |
Quercetin | 41.00 ± 3.25a | 31.35 ± 3.02d | 40.26 ± 4.29b | 38.84 ± 3.27c | 30.16 ± 3.44e | 0.036* |
Naringenin | 0.59 ± 0.06e | 1.97 ± 0.18a | 0.98 ± 0.08d | 1.19 ± 0.12c | 1.46 ± 0.13b | 0.034* |
Luteolin | 0.87 ± 0.07c | 1.58 ± 0.14a | 0.71 ± 0.06e | 0.76 ± 0.07d | 1.36 ± 0.12b | 0.002** |
Kaempferol | 6.04 ± 0.55e | 6.96 ± 0.71c | 7.10 ± 0.78b | 6.40 ± 0.62d | 7.62 ± 0.71a | 0.314NS |
Isorhamnetin | 31.58 ± 3.22c | 27.28 ± 2.55d | 25.81 ± 2.65e | 46.62 ± 4.25a | 32.41 ± 3.31b | 0.011* |
Total identified | 100.00 ± 9.56a | 100.00 ± 8.65a | 100.00 ± 9.54a | 100.00 ± 7.54a | 100.00 ± 8.56a | 0.000*** |
Values of phenolic compounds percentages are expressed as mean ± standard deviation (n = 3). Phenolic compounds percentages with small letters (a-e) are significantly different at P < 0.05. tr: trace, nd: not detected. ∗∗P< 0.01, ∗∗∗P< 0.001. NS: not significant. P: Probability. *** P< 0.001.
The chromatographic analysis of wormwood extracts showed that flavonoids represented the major fraction, four flavonoids were identified flavonols (quercetin, kaempferol, rutin and isorhamnetin), flavanol (epigallocatechin), flavanone (naringenin), flavone (luteolin) and coumarin where the percentages were highly influenced by the regional factor. Quercetin was the predominant compound in Bizerte (41.00 ± 3.25 %), Zaghouan (31.35 ± 3.02 %) and Kasserine (40.26 ± 4.29 %) regions. Quercetin is an important flavonol present in a number of plants which is characterized by its anti-inflammatory, antihypertensive, vasodilator effects, anti-obesity activities [48].
Isorhamnetin dominated in the region of Gabes (46.62 ± 4.25 %) and Tozeur (32.41 ± 3.31 %). This compound is the methylated metabolite of quercetin and it has been identified in different medicinal plants and fruits known for its interesting biological effects on human health such as the inhibition of the proliferation of a number of cancer cells [49]. The research conducted by Kim et al. [49] demonstrated the direct role of isorhamnetin on reducing tumor growth of the skin cancer.
As shown in Table 3, rutin was highly affected (P < 0.001) by the region factor and presented 12.88 ± 1.31 % in Kasserine. Kaempferol was detected in the five extracts of A. absinthium and the percentages were ranging from 6.04 ± 0.55 % in the region of Bizerte to 7.62 ± 0.71 % in the region of Tozeur. It should be mentioned that coumarin was exclusively detected in the extract of Kasserine region with a proportion of 3.83 ± 0.35 %. Isorhamnetin-3-glucoside and epicatechin 3-O-gallate were also found in the extracts of A. absinthium.
Msaada et al. [24] reported that the phenolic acids represented the major fraction in the methanolic extracts of Tunisian wormwood with 58.33 ± 6.21 % in Jerissa region. Lee et al. [50] showed that the salicylic acid was the dominant phenolic compound of A. absinthium leaf extract from South Korea. Craciunescu et al. [51] mentioned that quercetin, luteolin, apigenin and caffeic acid were the major phenolic compounds in A. absinthium growing in Romania.
The richness of the medicinal plants in polyphenols shows its importance in the agri-food, cosmetic and pharmaceutical fields as natural antioxidants. Indeed, the use of natural antioxidants instead of synthetic antioxidants (as butylated hydroxyanisole (BHA) and butylated hydroxytoluene (BHT) is more defended nowadays by the food industry [52]. Furthermore, Bubols et al. [53] mentioned that the flavonoids represent a vast array of active compounds with beneficial human health effects. Antioxidant and antiproliferative properties stand out as the main actions of flavonoids.
HCA was caried out in order to determine the relationship between the studied regions based on the phenolic compounds identified in wormwood extracts (Fig. 4). The dendrogram generated by HCA showed the presence of two groups. The first one composed of Gabes which was marked by the highest level of isorhamnetin (46.62 ± 4.25 %). The second group was divided in two subgroups where the first one contained Bizerte and Kasserine regions and the second one composed of Zaghouan and Tozeur regions. This subdivision could be due to a similar phenolic composition of the extracts between the regions in the same subgroup. For example, comparable levels of quercetin were found in Bizerte (41.00 ± 3.25 %) and Kasserine (40.26 ± 4.29 %) regions as well as in Tozeur (31.35 ± 3.02 %) and Zaghouan (30.16 ± 3.44 %) regions. This similarity of the phenolic composition of extracts between regions could be due to some environmental factors such as soil nature, rainfall, temperature, and genetic ones.
Antioxidant activity of the methanolic extracts
Total antioxidant capacity
The total antioxidant capacity test (TAC) was employed to estimate the antioxidant activity of the methanolic extracts from A. absinthium. Table 4 showed that the methanolic extract of Tozeur region has the best total antioxidant capacity in the order of 85.24 ± 0,07 mg GAE/g DW, followed by Gabes (42 ± 0.01 mg GAE/g DW), Bizerte (35.11 ± 0.021 mg GAE/g DW), Kasserine (33.82 ± 0.011 mg GAE/g DW) and Zaghouan (21.38 ± 0.012 mg GAE/g DW). Lee et al. [50] mentioned that wormwood leaves growing in South Korea presented a higher total antioxidant capacity reaching 168.28 ± 0.01 mg α-tocopherol/g DW for the methanolic extract and 187.48 ± 0.09 mg α-tocopherol/g DW for the ethyl acetate extract. A strong positive correlation between TAC and condensed tannins (r = 0.886) was observed.
Table 4 Total antioxidant capacity (TAC) (mg GAE/g DW) and reducing power (EC50 in µg/mL), DPPH (IC50 in μg/mL) of Artemisia absinthium extracts.
Bizerte | Zaghouan | Kasserine | Gabes | Tozeur | P | |
---|---|---|---|---|---|---|
TAC (mg GAE/gDW) | 35.11±0.021c | 21.38±0.012e | 33.82 ± 0.011d | 42.01 ±0.028b | 85.24± 0.069a | 0.000*** |
DPPH (IC50=µg/ml) | 52.828±4.77d | 31.459±1.41e | 56.014±3.68b | 107.659±3.47a | 54.630±3.23c | 0.000*** |
PR (EC50=µg/mL) | 788.66±5.63d | 922.52±3.85b | 1366.45±29.59a | 832.67±20.38c | 654.05±14.51e | 0.000*** |
BHT | 10.77 ± 2.98 | |||||
Ascorbic acid | 37.30 |
IC50 and EC50 values represent the mean of three replicates (n=3); letters (a-e) are significantly different at P < 0.05. ∗∗∗ significant at P< 0.001 %; P: probability.
DPPH-radical scavenging activity
DPPH radical scavenging activity is the fast technique to evaluate the antioxidant activity. The dose-dependent curve of the five wormwood extracts at different concentrations (25, 50, 1000 and 250 μg/ml) are given in Fig. 5. As can be seen in Table 4, the antiradical activity of the methanolic extracts from A. absinthium was highly influenced by the region factor. DPPH effect of the five wormwood extracts increased in the order of Gabes ˃ Kasserine ˃ Tozeur ˃ Bizerte ˃ Zaghouan, respectively. The methanolic extract of Zaghouan had an IC50 of 31.46 ± 1.42 µg/mL which was higher than that of BHT (IC50 = 10.77 ± 2.98 µg/mL). Msaada et al. [24] reported that DPPH activity of Tunisian A. absinthium extracts was highly affected by the region factor. In fact, the IC50 values varied from 9.38 ± 0.82 to 44.26 ± 1.92 µg/mL according to the sampling site. Moreover, the ethanolic extract of Romanian wormwood had a higher DPPH activity compared to our study with IC50 = 0.57 ± 0.05 mg/mL [51]. Mahmoudi et al. [45], reported an anti-radical activity lower than our results with an IC50 of 612 ± 30.6 µg/mL of the methanolic extract from Iranian wormwood aerial parts at the flowering stage. However, Riahi et al. [25] reported a DPPH scavenging power for Tunisian wormwood extract more important than that of our work (IC50 = 11.65 ± 0.95 µg/mL).
Ferric-reducing antioxidant power
In ferric reducing power assay, the dose-dependent curve of wormwood extracts at different concentrations (250, 500, 1000 and 1500 μg/mL) are given in Fig. 6. The ferric reducing power of wormwood extracts was significantly influenced (P < 0.05) by the region factor. The EC50 values varied from 654.05 ± 14.51 µg/mL to 1366.45 ± 29.59 µg/mL (Table 4). The aerial parts of A. absinthium collected from Tozeur showed the best reducing power with EC50 = 654.05 ± 14.51 µg/mL. The ferric reducing power of A. absinthium aerial parts extracts was found to increase in the order: Kasserine ˃ Zaghouan ˃ Gabes ˃ Bizerte ˃Tozeur. A strong negative correlation between ferric reducing power assay (FRAP) and total contents of phenolic compounds (r = - 0.8618) was found. These results suggested that flavonoids played a significant role in the antioxidant activity. Msaada et al. [24] reported that the methanolic extracts of wormwood, growing in Boukornine, showed an important ferric reducing power with EC50 = 2.16 ± 0.05 µg/mL.
Antibacterial activity of the essential oil
The evaluation of antibacterial activity of the five EOs of A. absinthium against the different bacteria strains was carried by the agar disc-diffusion method based on the determination of the inhibition zone (IZ). As it is seen in Table 5, IZ was highly affected by the region factor (P < 0.001).
Table 5 ANOVA analysis and antibacterial activity (IZ in mm) of the different essential oil of Artemisia absinthium collected from five different regions of Tunisia.
Bacteria strain | Collection region | d.l | F | P | Streptomycin (1 mg/mL) | ||||
---|---|---|---|---|---|---|---|---|---|
Bizerte | Zaghouan | Kasserine | Gabes | Tozeur | |||||
Gram-negative | |||||||||
Pseudomonas aeruginosa | 8.00 ± 1.13b | na | 8.30 ± 0.65a | na | na | 4 | 225.25 | 0.000*** | 17.66 ± 0.65 |
Escherichia coli | 28.70 ± 2.61b | 17.30 ± 2.85e | 31.00 ± 1.96a | 27.30 ± 2.36c | 24.70 ± 0.65d | 4 | 21.44 | 0.000*** | 18.33 ± 1.3 |
Serratia marcescens | 17.00 ± 0.00c | 19.70 ± 0.65a | 16.30 ± 0.65d | 18.00 ± 1.13b | 14.00 ± 1.13e | 4 | 24.68 | 0.000*** | 14.00 ± 0.01 |
Gram-positive | |||||||||
Bacillus licheniformis | 20.70 ± 2.36c | 25.00 ± 1.13b | 19.30 ± 1.31d | 26.30 ± 1.73a | 11.30 ± 1.31e | 4 | 50.69 | 0.000*** | 16.00 ± 0.02 |
Staphylococcus aureus | 23.30 ± 3.27a | 17.30 ± 2.36d | 20.30 ± 1.73c | 21.70 ± 3.27b | 10.00 ± 1.23e | 4 | 17.73 | 0.000*** | 15.00 ± 0.05 |
Enterococcus hirae | 20.30 ± 2.85b | 23.30 ± 3.27a | 13.00 ± 1.96c | 20.00 ± 1.58b | 20.00 ± 1.48b | 4 | 12.31 | 0.000*** | 13.00 ± 1.13 |
na: not active. Values of inhibition zones represent the average of three replicates (n=3). Letters (a-e) indicate significant differences at P< 0.05.
*** Significant effect at % 0.001.
For Gram-negative bacteria strains E. coli and S. marcescens, they were sensitive to the five EOs. However, P. aeruginosa was relatively resistant. Indeed, the highest activity was observed against E. coli strain with IZ = 31 ± 1.96 mm for A. absinthium EO of Kasserine region. This strain also presented a sensitivity towards A. absinthium EOs of Bizerte (IZ = 28.70 ± 2.61 mm), Gabes (IZ = 27.30 ± 2.36 mm) and Tozeur (IZ = 24.7 ± 0.65 mm) regions. The positive control streptomycin had an IZ = 18.33 ± 1.3 mm. However, A. absinthium EO of Zaghouan region was less active (IZ = 17.3 ± 2.85 mm). In agreement with our results, the EO of wormwood aerial parts collected from North Khorasan in Iran had a strong antibacterial activity against E. coli (IZ = 30 ± 0.11 mm). S. marcescens strain showed a higher sensitivity for A. absinthium EO of Zaghouan (IZ = 19.70 ±0.65 mm), Gabes (IZ = 18.00 ±1.13) and Bizerte (IZ = 17.00 ± 0.00 mm) regions. Nevertheless, A. absinthium EOs of Kasserine (IZ = 16.30 ± 0.65 mm) and Tozeur (IZ = 14.00 ±1.13 mm) were less active. The positive control streptomycin had an IZ = 14.00 ± 0.01 mm. The antibacterial test showed a normal development of P. aeruginosa strain. However, a low antibacterial activity was detected for the EOs of Bizerte (IZ = 8.00 ± 1.13 mm) and Kasserine regions (IZ = 8.30 ± 0.65 mm). The positive control streptomycin had an IZ = 17.66 ± 0.65 mm.
Concerning Gram-positive bacteria strains, Gabes EO exhibited the highest antibacterial activity against B. licheniformis (IZ = 26.30 ± 1.73 mm), followed by Zaghouan (IZ = 25.00 ± 1.13 mm) and Bizerte (IZ = 20.70 ± 2.36 mm) regions. The EOs of these regions were more powerful than the positive control streptomycin (IZ = 16.00 ± 0.02 mm). As shown in Table 5, A. absinthium EO of Bizerte region was the most active against S. aureus with IZ = 23.30 ± 3.27 mm. A. absinthium EOs of Gabes, Kasserine and Zaghouan regions showed a moderate antibacterial activity with IZ = 21.70 ± 3.27 mm, 20.30 ± 1.73 mm and 17.30 ± 2.36 mm, respectively. IZ of the positive control streptomycin was 15.00 ± 0.05 mm. For E. hirae, the highest antibacterial activity was observed with A. absinthium EO of Zaghouan region with IZ = 23.30 ± 3.27 mm which was greater than that of the positive control (IZ = 13.00 ± 1.13 mm). Msaada et al. [24] mentioned that IZ of Tunisian A. absinthium EO against S. aureus varied from 18.00 ± 1.13 to 25.00 ± 1.13 mm. Moghaddam et al. [54] reported that IZ of Iranian A. absinthium EO was around 32 ± 0.016 for S. aureus and 30 ± 0.11 mm for E. coli. It has also been demonstrated that Tunisian A. absinthium EO of Kasserine region was active against E. coli (IZ = 14 mm) and P. aeruginosa (IZ = 18 mm) [25]. However, Juteau et al. [54] mentioned that French A. absinthium EO had no antibacterial activity against E. hirae. Accordingly, to Kheyar et al. [56] and Saada et al. [57], the variability of the antibacterial activity of the EOs against the two types Gram-positive and Gram-negative bacteria strains is due to a difference in the ability of penetration of the active compounds present in EOs. In fact, it is difficult to attribute the antibacterial activity of a complex mixture to a single or constituent; also, possible synergistic and antagonistic effect of compounds in the EOs should be taken into consideration [40].
It has been conclusively shown that A. absinthium EOs were active against both Gram-positive and Gram-negative bacterial strains. In our present research, it could be deduced that the antibacterial effect of A. absinthium EO was region dependent. Indeed, the variability of the chemical composition and the different geographical parameters of the studied regions had an important role on the antibacterial activity of the EOs.
Conclusion
In conclusion, the present study showed that A. absinthium is characterized by an important antioxidant activity thanks to its richness in bioactive components which were highly influenced by the regional factor. As it was reported, considerable qualitative and quantitative differences existed between wormwood samples. It’s noteworthy to mention that this research is the first to evaluate the antibacterial activity of EO extracted from Tunisian wormwood against B. licheniformis, S. marcescens and E. hirae bacteria strains. Indeed, A. absinthium EO presented an interesting antibacterial effect against both Gram-positive and Gram-negative bacteria. According to our findings, A. absinthium EO presented a promising potential application for industries and could be used in several treatments particularly those of bacterial infections.