Introduction
Cobalt aluminate (CoAl2O4), known as Thenard's blue, is a normally spinel-type oxide that has the general formula AB2O4, where A represents a divalent cation in a tetrahedral site, and B is a trivalent cation located in an octahedral site. This compound has been applied as a catalyst [1], an electrode in photo-electrochemical cells [2] and as a ceramic [3,4], because of its high thermal stability, high catalytic activity and good electronic and optical properties.
Several synthesis methods have been used for the preparation of cobalt aluminate, including combustion [5], the use of thermal complex precursors [6], sol-gel methods [7], co-precipitation [8], sonochemical decomposition [9], and the use of inverse micro-emulsions [10]. However, the use of colloidal methods for the preparation of cobalt aluminate has not been reported previously. Colloidal methods involve simple processes, and allow the preparation of a wide variety of inorganic composites such as hydrous sulfides, phosphates, hydroxides, and oxides [11,12]. These methods also offer advantages in terms of the higher purity and compositional homogeneity of the final products and they do not require expensive instruments or sophisticated procedures to generate nanostructured materials. Recently, CoSb2O6 and ZnSb2O6 with a trirutile-type structure were synthesized using a colloidal method [13-15]. In these studies, the colloidal method allowed unique microstructural morphologies to be obtained: microcolumns and microspheres were obtained for CoSb2O6, and microtubes and microwires were obtained for ZnSb2O6. The form of these microstructures depended largely on the type of surfactant used in the synthesis; ethylenediamine and n-dodecylamine were used for CoSb2O6, while PVP and n-dodecylamine were used in the preparation of ZnSb2O6. This synthetic method enabled the production of nanoparticles of these compounds; such nanoparticles are suitable for gas sensor applications, because of their large specific surface area. The ability to produce nanosized powders of a material makes it very attractive for various applications; the properties of the material could depend on the particle size.
The goal of this work was to achieve the synthesis of nanoparticles of CoAl2O4 using a simple colloidal method in a non-aqueous environment, using the organic compound dodecylamine as a surfactant agent. Also, the effect of the dodecylamine concentration on the microstructure of the CoAl2O4 was investigated.
Materials and methods
Synthesis
Cobalt aluminate with a spinel-type structure was synthesized using a colloidal method, following the method reported by Michel et al. [13]. The synthesis of CoAl2O4 was carried out in two separate processes performed at room temperature. In the first, 1.45 g of cobalt nitrate hexahydrate (Mallinckrodt, USA, 99 %), 3.75 g of aluminum nitrate nonahydrate (Sigma Aldrich, México, 98 %), and 1 g of dodecylamine (Sigma Aldrich, México, 98 %), were separately dissolved in 5 mL of ethyl alcohol (Golden Bell, México, 98 %). Then, the cobalt nitrate solution was added to the dodecylamine solution under vigorous stirring. The aluminum nitrate solution was then added slowly, producing a transparent red colloidal solution with pH = 2. The resulting colloidal solution was subjected to an agitation process for 24 h. Solvent evaporation was then performed using a microwave oven (General Electric JES769WK, 700 W) at low power (140 W), leaving a solid precursor material. The resulting precursor was dried at 200 °C for 8 h using a furnace (Novatech muffle); finally, the powders were calcined at 800 °C for 5 h. In the second synthesis, the same process was followed but instead of 1 g, 2 g of dodecylamine was used. Figure 1 shows a flowchart for the synthesis of the CoAl2O4 powders.
Characterization
X-ray diffraction measurements were performed using a Siemens D500 diffractometer (radiation Cu Ka). The θ/2θ diffraction pattern was recorded from 20 to 70 degrees, in 0.02 ° increments. The microstructure of the powders was observed by SEM, using a JEOL JSM 6390LV microscope. The nanostructure was identified by TEM, using a JEOL JEM 1010 microscope. The UV-vis spectra were recorded using a Perkin-Elmer/Lambda2 spectrometer (λ = 200 to 1000 nm). For this analysis, a small amount of powder was ground in an agate mortar and then suspended in ethyl alcohol. The Raman spectra from 100 to 1000 cm-1 were measured at room temperature using a Renishaw 1000B Raman spectrometer (laser diode, λ = 830 nm).
Results and discussion
Figure 2 shows the XRD patterns for the CoAl2O4 powder calcined at 800 °C, for 1 g and 2 g of dodecylamine. In these patterns the main phase was the cubic CoAl2O4 spinel phase, which was identified using the JCPDF #44-0160 file. The spinel phase can be confirmed by the incipient presence of the (331) plane situated at 2θ = 49.2 °, and by the visual observation of its blue color, which is characteristic of cobalt aluminate. The average crystal sizes, which were calculated using Scherrer's formula, using the XRD (311) plane, were ~ 19 and 24 nm for 1 and 2 g of dodecylamine, respectively.

Figure 2 XRD patterns for the CoAl2O4 powders obtained using 1 and 2 g of dodecylamine, and subsequent calcination at 800 °C.
The surface microstructure of the CoAl2O4 powders calcined at 800 °C was observed using SEM. Figure 3 shows micrographs of the powders prepared using 1 g of dodecylamine; the powders were formed from semispherical particles with sizes between 1 and 9 µm, with an average size of 3.7 µm (Figure 3A). The powders prepared using 2 g of dodecylamine were formed from thin laminas with a uniform thickness (Figure 3B). The length of the laminas was between 10 and 60 μm and the width of the laminas was in the range of 5 - 13 µm
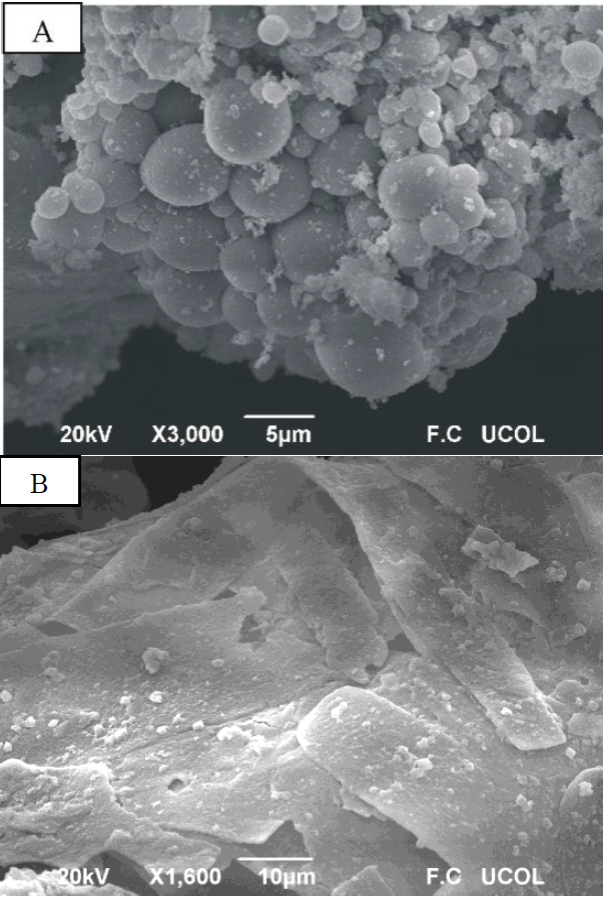
Figure 3 Surface morphology observed using SEM for the CoAl2O4 powders synthesized using 1 g of dodecylamine (A) and 2 g of dodecylamine (B); the powders were calcined at 800 °C.
The mechanism of formation of colloidal particles was studied by LaMer and Dinegar [16]. This mechanism was established to explain the variation of the concentration of the reagents with the time. According to this mechanism the concentration of the reagents in the colloidal solution increased quickly. Then the concentration of reagents rising about the supersaturation limit for a short period of time and a rapid nucleation process occurs with the formation of large number of crystal nuclei. This leads to a decrease of the concentration. Later, the process of growth of the particles is developed by diffusion. In our case, the final colloidal solution does not present the supersaturation although the nucleation process may occur when the aluminum nitrate solution was added to the cobalt and dodecylamine solution; meanwhile, the process of growth was developed during the stirring of the colloidal solution. The amount of dodecylamine in the colloidal solution could affect the particle growth due to the suturing of nanocrystal surfaces [17] and hence results in the formation of CoAl2O4 nanoparticles with different sizes and morphologies. In this work, semispherical particles and laminas of CoAl2O4 were obtained when 1 and 2 g of dodecylamine were used, respectively.
Figure 4 shows TEM images of the powders prepared using 1 and 2 g of dodecylamine calcined at 800 °C. When 1 g of dodecylamine was used, the obtained material consisted of agglomerates of nanoparticles, as shown in Figure 4A. The particle sizes were in the range of 5 - 45 nm, with an average size of 17 nm (Figure 4B). When 2 g of dodecylamine was used, fine agglomerates of nanoparticles with an average size of 13 nm were observed (Figure 4C). The sizes of the CoAl2O4 nanoparticles were in the ranged from 6 to 24 nm (Figure 4D). However, several particles with a size of approximately 50 nm were also observed. The TEM images revealed that the microstructures observed in the SEM images were formed from nanosized particles.
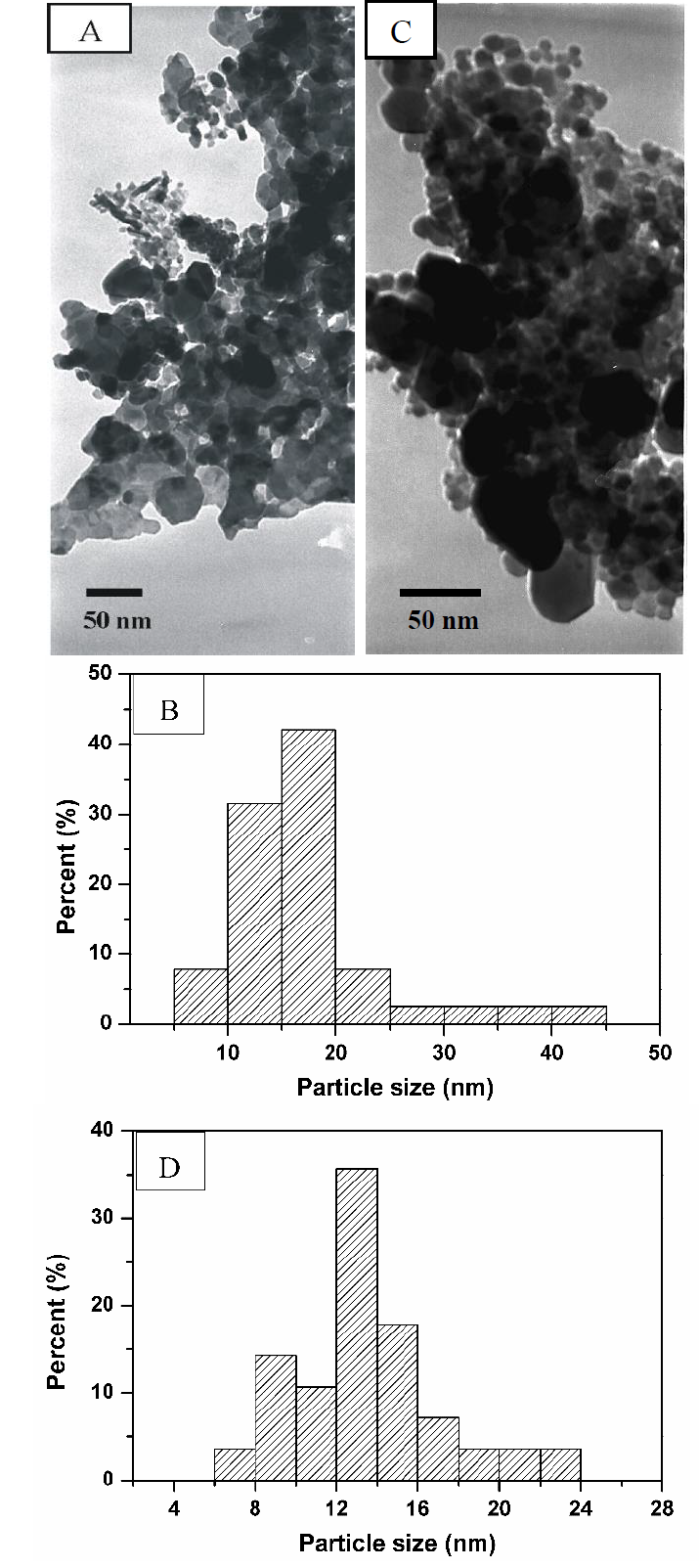
Figure 4 TEM images and particle size distributions of CoAl2O4 prepared using 1 g of dodecylamine (A,B) and 2 g of dodecylamine (C,D).
UV-vis spectra from CoAl2O4 powders prepared using 1 and 2 g of dodecylamine are show in Figure 5. In the samples (both calcined at 800 °C), three absorption bands were observed in the visible region, at approximately 548, 591, and 639 nm. These absorption bands are characteristic of the CoAl2O4 blue-phase, and had their origin in the spin transitions allowed for the Co2+ tetrahedron (3d7 configuration); these transitions were assigned to 4A2(F) → 4T1(P) [18]. Rangappa et al. [19] reported a similar spectrum when they prepared nano-CoAl2O4 pigment using using a supercritical water method involving organic binding. The same behavior was reported by Feldmann [4].
According to the group theory for oxides with a spinel structure, five active Raman vibration modes can be expected: A1g + Eg + 3Fg [20]. However, previous studies have shown that most of these five vibration modes can arise owing to a reduction in the crystal symmetry of the spinel structure [21]. Figure 6 shows the Raman spectra for the CoAl2O4 spinel (blue powder) synthesized using 1 and 2 g of dodecylamine. For the powders synthesized using 1 g of dodecylamine, seven bands located at 194, 417, 485, 519, 618, 686, and 770 cm-1 were observed; bands located at 196, 411, 483, 523, 620, 690, and 756 cm1 were observed for the powders synthesized using 2 g of dodecylamine. The low intensity bands located around 417 and 770 cm-1, and 411 and 756 cm-1, for the samples prepared using 1 and 2 g of dodecylamine, respectively, are consistent with those reported by Jongsomith [22] and Kock [23] for the formation of CoAl2O4, and with the vibration bands observed for other aluminates such as MgAl2O4 or ZnAl2O4, which are located near 410 and 755 cm-1 [24,25]. The slight shift observed in the bands was owed to the atomic mass of Co. Based on previous studies made by Stanojevic [21] and Julien [26] on ZnCr2O4 and LiMn2O4 spinel oxides, it was possible to assign the active Raman vibration modes for the CoAl2O4 as follows: one of the most intense peaks, located at 196 cm-1, is assigned to the Co-O deformation vibration with symmetry F2g. Another intense peak observed at 483 cm-1 is related to the combination of the Al-O and Co-O symmetrical stretching vibrations with symmetry Eg. Mean intensity peaks located at 523 and 620 cm-1 are generated by asymmetric and symmetric Al-O stretching vibrations, respectively, which corresponded to an F2g symmetry. The highly symmetric A1g vibration, which is related to the contraction of the Al-O linkage, generated a very intense peak at 690 cm-1. Therefore, the Raman spectroscopy studies confirmed the formation of the CoAl2O4 spinel phase as a result of the method presented in this work.
Conclusions
Cobalt aluminate with a spinel type structure was synthesized by a colloidal method, using dodecylamine as a surfactant. Using this method, a pure CoAl2O4 phase was obtained at 800 °C with a cubic spinel phase, as identified by DRX analysis. The spinel phase was confirmed by the presence of the (331) plane at 2θ = 49.2 °. SEM analysis revealed that the size and morphology of the cobalt aluminate particles were strongly influenced by the amount of dodecylamine used in the synthesis. When 1 g of dodecylamine was used, nanostructured semispherical particles (~17 nm) were produced, while nanostructured laminas (~13 nm) were obtained when 2 g of dodecylamine was used. The formation of the cobaltate blue phase was confirmed using UV-Vis and Raman spectroscopy. We expect that the colloidal method presented could be applied for the preparation of other oxides, because this method gives good control over the stoichiometry, provides unique morphological characteristics, and shows good repeatability for the formation of a pure CoAl2O4 phase.