1 Introduction
Antioxidants play a vital role in food systems and in the human body because they reduce the oxidative processes. In food systems, antioxidants are useful in retarding lipid peroxidation, and secondary lipid peroxidation product formation; therefore they help maintaining the flavor, the texture, and in some cases, the color of the food product during storage (Elias et al., 2008).
The consumption of antioxidants and/or diets enriched with these, seems to prevent or at least reduce the deterioration of the organism caused by excessive oxidative damage (Martínez et al., 2015). Several in vitro studies have been done for evaluating the Total Antioxidant Capacity (TAC) of food products. However, there is no official standardized method; therefore it is recommended for each evaluation to be made in the context of several oxidation conditions and different measurement methods (Zulueta et al., 2009). The methods for measuring antioxidant capacity are basically classified into two groups depending on the reaction mechanism: methods based on Hydrogen Atom Transfer (HAT) and methods based on Electron Transfer (ET) (Huang et al., 2005). Most of the HAT-based assays apply a competitive scheme in which the antioxidant and the substrate compete for thermally generated peroxyl radicals through the decomposition of azo- compounds. On the other hand ET-based assays measure the capacity of an antioxidant through the reduction of an oxidant; the latter changes color when reduced. The degree of colour change is correlated with the sample's antioxidant concentrations. Trolox Equivalent Antioxidant Capacity (TEAC) and Oxygen Radical Antioxidant Capacity (ORAC) assays are the most popularly used ET and HAT methods respectively (Zulueta et al., 2009).
Traditionally, dietary protein is regarded as a source of energy and essential amino acids; however, proteins and protein hydrolysates derived from different food sources have also shown biofunctional activities (Gómez et al., 2013; Martínez et al., 2015; Reyes et al., 2015). There has been an increasing interest in recent literature to identify, characterize, and assess the antioxidant potential of both, protein and peptides, as well as a general concern about their possible applications as functional foods (Samaranayaka and Li-Chan 2011).
A feasible and economical way to produce protein hydrolysates from an environmental point of view is by using waste materials from food plants, and farms in general. For example, animal blood produced during the slaughter is one of these cases; in addition of being a valuable source of protein (Del Hoyo et al., 2008), it has been found to produce several bioactive peptides (Catiau et al., 2011; Gómez et al., 2013; Gómez and Zapata 2014; Liu et al., 2010; Salgado et al., 2011).
The goals of this work were: 1) To study the effect of the DH on the total antioxidant capacity of bovine plasma hydrolysates by using two measurement methods (ORAC and TEAC) based on different reaction mechanisms and 2) To identify the peptide fractions showing the greatest antioxidant activity. A final issue was to establish the correlation between the TEAC method and the ORAC method.
2. Materials and methods
2.1 Reactants and materials
Liquid plasma was obtained from a commercial supplier in Medellín, Colombia by taking samples of three different production batches. It was then stored at -20°C before use. As for the enzymatic hydrolysis, Alcalase 2.4 L alimentary degree was used (Novo Nordisk Co., Dinamarca); its activity (2.45 ± 0.07 AU/g) was verified with the modified method of Takami et al., (1989). In addition Trolox (6-hydroxy-2,5,7,8-tetramethylchroman-2- carboxylic acid) Sigma-Aldrich (St. Louis, USA), a hydrophilic analogue of vitamin E, 2,2'-azinobis(3-ethylbenzthiazoline-6-sulfonic acid) (ABTS), 2,2'- azobis (2-amidinopropane) dihydrochloride (AAPH), and fluorescein sodium salt, from Sigma-Aldrich (St. Louis, USA) were purchased. All other chemicals and reagents used were of analytical grade.
2.2 Bovine plasma hydrolysis
The hydrolysis was carried out in a glass reactor for 2 h, as it has been shown that hydrolysates with higher antioxidant activity are obtained at that time (Gómez et al., 2013). 800 mL of bovine plasma solution (8 mg protein/mL) was hydrolyzed with Alcalase 2.4 L at 60 °C and pH 8.0. The enzyme to substrate ratio (E/S) was 8:100 (g/g). The pH and temperature control were done with a glass-combined electrode connected to a Metrohm Titrando 842 operated by a PC (software Tiamo 1.2.1). The reaction system was maintained in permanent agitation (200 rpm) using a magnetic agitator 801 (Metrohm, Switzerland). The reaction was monitored along with the determination of the DH; a pH-stat method was used consisting of keeping the pH of the system constant by adding a base into it. The purpose was to neutralize the protons released in the hydrolysis reaction (Adler-Nissen 1986).
The base consumption can be related to the DH as shown in Eq. (1) (Marquez and Vazquez 1999).
Where htot is the total number of peptide bonds present in the native protein, B is the consumed volume of base in L, MP is the protein mass in kg, NB is the concentration of base and α is the degree of dissociation of the amino acid groups released in the reaction; this value is given in function of the pH and the temperature of reaction, according to Eqs. (2)-(3) respectively. For this experiment a htot of 8.3 mol/kg was used as previously reported for blood proteins (Adler-Nissen 1986).
The degree of dissociation α was found as shown in Eq. (2) (Forghani et al., 2012):
The pK values at temperature (Kelvin) were calculated from Eq. (3) (Steinhardt et al., 1964):
After hydrolysis, the solution was heated up to 85 °C for 10 min to inactivate the enzyme. The hydrolysate was later centrifuged at 3420 g for 20 min in order to precipitate the non-hydrolyzed fraction.
2.3 TEAC assay
This method was used as described by Re et al., (1999), based on the capacity of a sample to inhibit the ABTS radical (ABTS·+) compared with a standard antioxidant reference (Trolox®). The ABTS·+ radical was generated by chemical reaction of potassium persulfate (2.45 mM) with ABTS (7 mM). The working solution was prepared by taking a volume of the previous solution and diluting it in a phosphate buffer solution (PBS) (5 mM, pH 7.4) until the absorbance at λ= 730 nm was 0.70 ± 0.02.
One milliliter of the ABTS·+ radical was mixed with 100 mL of the sample or standard and it was left in a dark place at 30 °C for 30 min; afterwards, the absorbance (730 nm) was measured. The Trolox calibration curve was prepared for a concentration range of 0-250 μM, and the absorbance value obtained for the samples was interpolated to calculate the concentration in trolox equivalents (μM TE); the results were reported as micromoles of Trolox equivalents per gram of protein (μmol TE/g of protein).
2.4 ORAC assay
The ORAC method was used with fluorescein (FL) as the "fluorescent probe" as described by Ou et al., (2002). The samples were diluted in PBS (10 mM, pH 7.4). A mixture of 150 μL of fluorescent probe FL (1 μM) and 25 μL of the samples were pre-incubated for 30 min at 37°C. Then, 25 μL of an AAPH solution in PBS (250 mM) was added. Fluorescence intensity was measured every 2 min during 120 min with excitation and emission wavelengths set at 485 nm and 520 nm, respectively. The Trolox calibration curve was prepared for a concentration range of 0-200 μM; the area under curve (AUC) for the samples was interpolated to calculate the concentration in trolox equivalents (μM TE); the results were reported as μmol TE/g of protein.
2.5 Purification of peptides with antioxidant activity
BPH with DH of 19.1%, which exhibited a higher antioxidant activity, was fractioned by ultrafiltration (Amicon ultra-4, Millipore, USA) with membranes of two different molecular weight cut-offs (3 kDa and 10 kDa). Three fractions (<3 kDa, 3-10 kDa, and >10 kDa) were separated and stored at -20°C. The antioxidant activity of these fractions was determined once again.
The fraction obtained from ultrafiltration with the greatest antioxidant activity, was fractioned by anion- exchange chromatography on a DEAE-Sephadex support (20 x 100 mm), balanced with PBS 0.01 M (pH 7.8) and eluted with a linear gradient of NaCl from 0 to 1 M. Eluted peaks were detected by ultraviolet absorbance at 280 nm. The antioxidant activity of chromatographic peaks was determined once more.
The peak obtained in the anion exchange chromatography that showed the greatest antioxidant capacity was separated by Reversed-Phase High Performance Liquid Chromatography (RP-HPLC) on an octadesilsilic column (C18) (250 x 10 mm; 5 μm; Restek, Pennsylvania, USA), at a flow rate of 2 mL/min. Trifluoracetic acid (TFA) 0.1% was used as solvent A, and acetonitrile/TFA 0.1% (99:1 v/v) as solvent B. The sample was eluted by using a gradient of B as follows: 0-5 min, 0% B; 5-20 min, 0-15% B; 20-50 min, 15-35% B and 50-53 min, 35- 70% B. The eluted peaks were detected by UV at an absorbance of 280 nm, and the antioxidant activity of chromatographic peaks was determined again.
2.6 Analysis of the antioxidant peptides by mass spectrometry
The chromatographic peak obtained from RP-HPLC with the greatest antioxidant activity was mixed in equal parts with a saturated solution of α- cianodihoxicinamic acid (α-CHCA), dissolved in acetonitrile at 50% in water with 0.1% of TFA. Some drops of 1 μL of these mixtures were placed on a metallic plate for ionization MALDI; next, they were left to dry at room temperature. An external calibration for modes MS and MS/MS was made through a mixture of CalMix-5 (ABSciex) standards. The spectra were obtained by using a total of 1625 shots/spectrum and a laser intensity of 3000. The most representative precursors were fragmented through MALDI-TOF-TOF and the resulting spectra were analyzed by the ProteinPilot 4.0.8 (ABSciex) software, comparing them against the UniProt/SwissProt (20120518) data base; the purpose was to obtain the most probable peptide sequences.
Additionally, peptide fragmentation was manually done through nESI-MS/MS by direct infusion. The sample (10 μL) was put into a metalized capillary tube (Proxeon), and was then subjected to 1200 volts, before it was analyzed in a QTrap 3200 instrument (Applied Biosystems, EEUU) equipped with a nano- spray source.
2.7 Statistical analysis
All the experiments were carried out in triplicate; the data are presented as mean ± standard deviation. The regression analyses between the antioxidant capacity of a sample and DH, and between TEAC and ORAC assays were calculated by Stagraphics® Centurion XV statistical software (StatPoint Technologies, Inc. Virginia, USA), the significance was established at p < 0.05. Significant differences (p < 0.05) between means were identified by using a LSD test.
3 Results and discussion
3.1 Effect of DH on antioxidant activity of BPH
The hydrolysis was performed according to the described procedure. After two hours of reaction, the DH reached a value of 19.1 ± 0.23%. It was determined according to equation 1, with α and pK values were calculated using equations 2 and 3 respectively. The hydrolysis behavior in time is shown in Table 1, where there is a typical trend of enzymatic hydrolysis of food proteins; it shows a high speed hydrolysis at the beginning of the reaction and a progressive decrease at the same time (Figueroa et al., 2013; Lamsal et al., 2007).
Table 1 Comparison between ORAC and TEAC values of the PBH with different DH.

a-f Means in the same column with different superscript show significantly statistic differences (p < 0.05)
The BPH antioxidant activity with different DH shown in Table 1 reached values above those reported for other proteinaceous hydrolysates (Nalinanon et al., 2011) and even greater than the antioxidant activity reported for some extracts of fruits and vegetables (Floegel et al., 2011; Romero et al., 2015; Silva et al., 2007). Thus, BPH can become a great alternative as antioxidant additives in the meat industry, which it could also benefit some tecnofuncionales properties that have been shown to bovine plasma (Isaza et al., 2010).
The greatest antioxidant activity was reached at 19.1% DH, with values 853.3 ± 22.7 μmolTE/g and 1388.8 ± 10.7 μmolTE/g, for ORAC and TEAC methods, respectively. The differences in the antioxidant capacities found with the two methods could be explained by the different nature of the two assays.
The antioxidant capacity of BPH as demonstrated in the present study may be attributed, in part, to protein structural changes. Non-hydrolyzed plasma protein, because of its compact structure, had a minimal antioxidant activity. Disruption of the native protein structure by enzyme hydrolysis resulted in the opening and exposure of active amino acid residues and patches capable of reacting with the oxidants (Kong et al., 2007).
Other research works have shown a direct relationship between soluble protein/peptide concentration and the increase in the antioxidant activity; in the same way it has been demonstrated that the smaller peptides resulting from hydrolysis are more polar than polypeptides in non-hydrolyzed proteins, in consequence they may form stronger hydrogen bonds with water; therefore, they are more soluble in aqueous solutions (Liu et al., 2010). This way we could understand why enzymatic hydrolysis is an alternative for increasing the antioxidant capacity of food proteins.
After the analysis, we observed that for both methods, TEAC y ORAC assays, the increase in the DH corresponded to a greater antioxidant capacity, and in both cases the experimental data were fit to squared-X models (p < 0.05), μmolTE/g = 773.854 + 1.674DH2 with r 2= 0.969, and μmolTE/g = 658.485 + 0.519DH2 with r 2= 0.953, respectively. Figure 1 shows the plot of the fitted models for the antioxidant activity in relation to DH.
3.2 Correlation between the TEAC and ORAC methods
Upon comparing the antioxidant capacity results obtained from the TEAC method and the ORAC method we found a correlation coefficient of 0.974 when the DH changes (r2=94.87, p < 0.05); this gives an increase in total antioxidant capacity with both methods. The experimental data were fitted to a double squared model (Fig. 2), μmolTE ORAC = sqrt (311745 + 0.214467*μmolTE TEAC2).
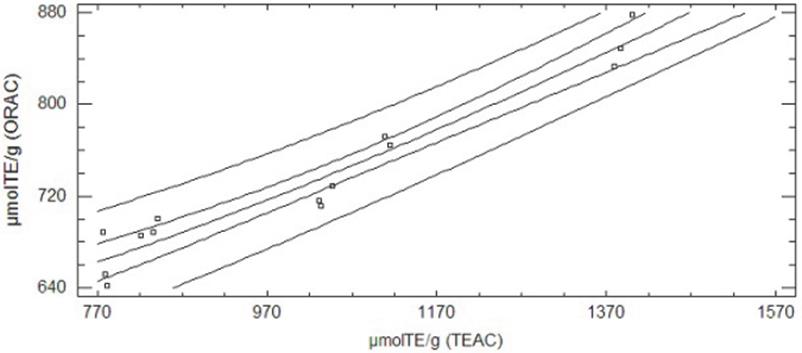
Figure 2 Adjusted model plot for the relationship between antioxidant capacity results of PBH with DH 19.1% obtained with the TEAC method and the ORAC method.
Several authors have obtained correlations between the results found after analyzing antioxidant capacity with the TEAC and ORAC methods. This seems to be for foods in which the main antioxidants are water-soluble and, as seen, the action of these antioxidants easily takes place with both methods (Proteggente et al., 2002; Stintzing et al., 2005).
3.3 Isolation of antioxidant peptides
The antioxidant activity of three ultrafiltration fractions with different molecular weights (<3 kDa, 3-10 kDa y >10 kDa) is shown in Table 2. We can see that the antioxidant activity increased as the molecular weight diminished; therefore the <3 kDa fraction exhibited the highest TEAC and ORAC activity (p < 0.05). This result corresponds to the ones reported by Nalinanon (Nalinanon et al., 2011) in that those antioxidant peptides from food sources have a molecular weight between 500 and 1800 Da.
Table 2 Antioxidant capacity of peptide purified fractions of PBH with DH 19.1

a-f Means in the same column with different superscript show significantly statistic differences (p < 0:05).
To identify the antioxidant peptides derived from BPH, the <3 kDa antioxidant hydrolysate, which had the highest antioxidant activity, was separated by ion- exchange chromatography into three fractions (I, II and III) (Fig. 3). Fraction I eluted with 0% NaCl was found to have a higher antioxidant activity (Table 2). This fraction was further separated by RP-HPLC (Fig. 3).

Figure 3 Separation processes of the peptidic fraction with the highest antioxidant capacity, A) Elution anion exchange chromatography profile of BPH (DH: 19.1%, <3 kDa), B) Reverse-phase HPLC profile of peak I.
The antioxidant activity of the three most representative peptide fractions was evaluated (Ia, Ib and Ic). Subfraction Ia had the highest antioxidant activity, increasing 25 times with respect to the non- hydrolyzed bovine plasma (Table 2).
The high activity found in peak Ia shows the potential application this peptide has as a natural antioxidant that may even substitute synthetic antioxidants. It may additionally present nutritional and functional properties besides its antioxidant activity (Schaafsma 2009); furthermore, since it obtained its applications in foods from natural food proteins it is less restricted than synthetic antioxidants whose use is regulated because of their possible health risks (Sarmadi and Ismail 2010).
3.4 Mass spectrometry analysis
The material found at the peak Ia was analyzed using MALDI-TOF mass spectrometry positive ion mode, with m/z values between 700 and 4000. Figure 4 shows the presence of several mono-charged ions with masses below 1700 Da, in which an intensive precursor stands out at 879.6 Da, other precursors stand out as well with values of 868.5 and 1058.2 Da (monoisotopic masses). A fragmentation of these three precursors was performed through MALDI-TOF-TOF and the resulting spectra were compared against a search in the UniProt/SwissProt data base (201202). The results suggest the sequence Q(K)Q(K)PVRDQ(K) with 95% confidence for the 879.6 Da precursor; the other two precursors did not generated clear enough fragmentation spectra after the MALDI-TOF-TOF analysis.
As a complement, a peptide fragmentation was done through a manual nESI-MS/MS. A precursor of 405.2+2 (M+H+=809.4) generated a full fragmentation spectrum that corresponded to the octapeptide sequence GAHQPSGR (Fig. 5), compatible with the hypothetical BOS_23161 protein.
Conclusions
This study shows that the antioxidant capacity of bovine plasma hydrolysates increases as the DH is increased. This increment fits the square-X model with a r 2 of 0.969 and 0.953 (p < 0.05) for the methods TEAC and ORAC respectively. Likewise, it is possible to correlate the antioxidant activity obtained through methods TEAC and ORAC with a correlation coefficient of 0.974 (p < 0.05) fitted to a double squared model. It is also possible to separate a peptide fraction from enzymatic hydrolyzed of bovine plasma with a high antioxidant capacity of 4457.9 ± 72.5 and 1409 ± 43.41 μmolTE/g of protein for TEAC and ORAC methods, respectively. From this peptidic fraction was possible to identify two peptides with sequences Q(K)Q(K)PVRDQ(K) and GAHQPSG.