Introduction
Several different Constructed Wetland (CW) technologies have been deployed and evaluated in Europe and the United States for decentralized treatment of domestic wastewater (Vymazal & Kröpfelová, 2008; Kadlec & Wallace, 2009). Despite their recognized environmental benefits and low capital and operating costs, these same CW technologies have been implemented only infrequently in Latin America or on an experimental basis (Torres et al., 2017; Burgos et al., 2017; Caselles-Osorio et al., 2017).
Among, current wastewater treatment technologies, CW technology is considered as an eco-friendly, low cost technology with some distinct advantages such as; low operation and maintenance cost, as well as provide aesthetic value, generate usable plant biomass, and help support wildlife habitat (Sehar et al., 2014).
Until now most wastewater treatment technologies, including CWs, have been designed and operated to optimize removal of organic contaminants, pathogens and fertilizer nutrients based on mandated effluent discharge standards (Vymazal, 2014). Moreover, alternative forms of wastewater treatment are often needed, as centralized treatment is not always an economical option. This is especially the case in rural and remote regions in developing countries (Wu et al., 2016). In Central and South America, discharge standards are not mandated or enforced and as a result sanitary wastewater is often discharged via straight pipe to receiving streams, where they degrade pristine freshwater resources, pollute highly productive estuaries, and exacerbate human health issues. However, in various communities and economic sectors of some countries like Mexico, there are applications of new technologies for wastewater treatment throughout the country (Noyola, 2013; De Anda, 2017).
The re-use of wastewater in agriculture is gaining wider acceptance in many parts of the world (Gatta et al., 2015). In fact, the World Health Organization (WHO, 2006) considers that wastewater in agriculture is a legitimate method of combining water and recycled nutrients, but recommends it should be done safely to maximize public health gains and environmental benefits. Domestic wastewater is potentially rich in nutrients and has traditionally been used as an important source of fertilizers for either agriculture or aquaculture. Yet, many of the advanced wastewater treatment technologies in use today, in many countries discharge treated wastewater to receiving streams with little regard for reclamation of residual nutrients or water reuse. Reused wastewater for irrigation is considered to be a major resource of nutrients in many parts of the world (Boyden and Rababah, 1996). Cirelli et al. (2012) affirm that under controlled conditions, treated wastewater can be used as an additional water resource to increase the eggplant and tomato production in water-scarce environments. Similarly, García-Pérez et al. (2014) assure that nutrient recovery through the direct use of sewage as fertilizer and water reuse is feasible to have a crop of sunflowers (Helianthus annuus L.).
Population growth, urbanization, migration and industrialization, along with increases in production and consumption, have generated ever-increasing demands for freshwater resources causing critical and inter-related problems linked to food security and sustainable use of land, water and energy resources (WWAP, 2015). For example, there is already intense competition for water among domestic, industrial, and agricultural uses (FAO, 2011).
Given that fertilizer nutrients and most forms of energy are expensive and world prices are escalating, there is strong impetus to develop energy-efficient wastewater treatment systems in which irrigation and reuse of nutrient-rich water will be utilized. In this decentralized paradigm, it is envisioned that treated wastewater will not be discharged to water bodies as in the past, but will instead be reused judiciously to culture valuable crops onsite, or to irrigate communal gardens, pastures, and tree farms (Nelson et al., 2008). These actions will reduce pollution caused by discharge of treated and untreated wastewater to receiving streams, make valuable use of water and residual nutrients, help to recharge depleted groundwater resources, and serve as a potential source of food and income for urban and rural residents.
Taking the above into account, the aim of this study was to evaluate integrated CW technologies for treating domestic wastewater while producing a crop of tomatoes (Lycopersicum sculentum) and analyze removal efficiency of dissolved organic matter and nitrogenous compounds from domestic wastewater by subsurface flow CWs during two years.
Materials and methods
Study location and Pilot plant design
During March and April of 2008, a small pilot-scale integrated CW wastewater treatment facility was designed, built and put into operation at the Biological Reserve CAOBA, which is located 15 km from the city of Santa Marta (Colombia), in the Manzanares river basin, a warm region, which is characterized by a complex mosaic of habitats and very high levels of biodiversity. The design of this integrated system was based in part on in-depth studies of shallow horizontal subsurface-flow wetlands, HSSF (Caselles & García, 2006; 2007). The systems provided primary and secondary wastewater treatment for 6 people. The system operated in serial flow (Figure 1), and consists of a 2.6 m3 sedimentation tank for primary clarification, followed by a series of 5 subsurface-flow wetland cells (1m x 1m x 0.5m), a single surface-flow wetland (2m x 0.9m x 0.5m), and a lagoon reservoir (1 x 0.9 x 0.5) polishing of the wastewater and water reuse options (irrigation). The lagoon provided an area for culture of native floating plants and nutrient polishing. The multicell design enabled frequent redistribution of flow to mitigate short-circuiting and to enable periodic removal of sludge from cells 1 and 2.
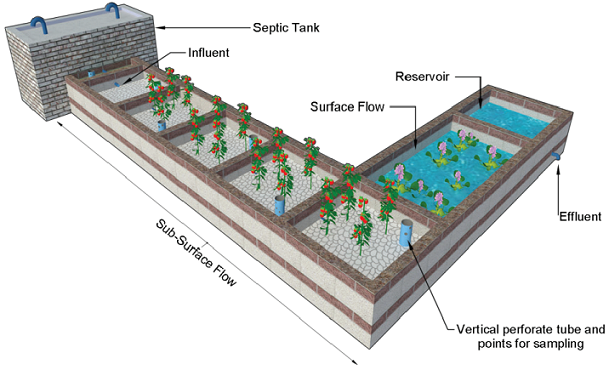
Figure 1 Schematic representation of the pilot plant. Note both wetlands types and a terminal holding reservoir for water reuse options. The sampling points where put in the corner of each cell, assuming the same possibility to examination the wastewater quality
Multiple cell designs with appropriate plumbing also allow for bypassing influent cells to facilitate periodic removal of sludge. The clarifier and cells were constructed of hollow cement blocks and sealed with commercial epoxy paint. Each of the subsurface-flow cells were backfilled to a depth of 0.5 m with screened 10 mm river gravel harvested from the nearby river. The wetland system was backfilled to a depth of 0.1 m with river’s sand to facilitate rooting of aquatic plants. Plastic standpipe distribution headers at the entrance and exit of each cell facilitated wastewater distribution and maintenance of water level at approximately 0.4 m depth. In addition, each of cells had slotted vertical plastic pipes installed at strategic locations to allow for in situ measurements and collecting of discrete whole column water samples
Influent wastewater flow rates (L/minute), were estimated using the bucket and stop watch method at one to two hour intervals during daylight hours. It is envisioned that integrated systems such as portrayed in Figure 1 can be planted with adapted vegetable crops, such as tomatoes, and that treated wastewater can be stored in the reservoir and reused as toilet flush water or nutrient rich water for irrigating adjacent crop lands, fish ponds or gardens. These objectives are similar to those as described for high diversity wastewater gardens and irrigated ecoscopes (Nelson et al., 2008).
Planting tomatoes (Lycopersicum sculentum)
Seeds of tomatoes were germinated in culture flats and grown for 30 days prior to being transplanted into the treatment system. At the time of transplanting, the plants ranged from 10-15 cm high and were planted on 20 cm. It should be noted that the first treatment cell in the series was not planted due to severe organic clogging. After 94 days of culture, the tomato biomass, including roots, shoots and fruit were harvested in each cell and processed. Harvested tomato fruits were stored in a cardboard box and transferred to laboratory. Two samples of fruit from each treatment cell were rinsed in dechlorinated tap water and the surface and flesh analyzed for coliform bacteria. The remaining tomatoes were weighed in Ohaus TR6RS balance for total biomass calculations.
Pilot plant performance monitoring
Water quality monitoring was carried out between October of 2009 and May of 2010. During the period December/09 to March/10, tomatoes were cultivated in the treatment cells. During the study, four (4) water samples were collected from each of the 5 treatment cells and four of influent and effluent. The average values were reported. All samples were preserved in an insulated cooler and temperatures were maintained at near 5ºC. Subsequently, and within 24 hours, the samples were analyzed for organic matter (COD, BOD5), ammonium nitrogen (NH4+-N), nitrate nitrogen (NO3−-N), nitrite nitrogen (NO2−-N) and ortho-phosphate (PO4−3-P) using methods as described in (APHA-AWWA-WEF, 2005). Water temperature, dissolved oxygen (DO), pH and conductivity measurements were obtained by monitoring the water within the vertical perforated PVC tubes (Figure 1). Measurements were taken at the midpoint of the water depth and water temperature, conductivity, pH and DO measurements were recorded with WTW 315i Set probes. Paired comparisons of inlet and outlet concentrations for each treatment cell were evaluated for COD, BOD5, (PO4−3-P), (NO3−-N), (NO2−-N) and (PO4−3-P).
Microbiological examination for total coliform (TC) and fecal coliform (FC),
Samples of wastewater were collected in labeled plastic bottles, placed in a cooler and transported directly to the laboratory where they were stored under refrigeration. The samples were analyzed within 24 h of collection for the presence of coliform bacteria group (total and faecal coliform) using the multiple-tube fermentation technique (APHA-AWWA-WEF, 2005).
Results and discusion
Septic tank effluent can be a strong pollutant, but it can also be a rich and valuable source of organic carbon, total nitrogen (39-82 mg/L), phosphorus (11-21 mg/L), and other essential micro nutrients (Boom, et al., 2008; EPA, 2002; Ferreira et al., 2007). Household wastewater flows used in this study ranged from 423.4 to 1102.3 L/d. Higher flows were recorded in the early morning and late afternoon hours. Daily flow averaged 832.2 L/day (138 L/person/day), which is within the range of Minimum and maximum net endowment reported in Colombia (RAS, 2000). Assuming 40% void space of the gravel substrate and 0.4 meters depth, total water volume (excluding the septic tank), totaled approximately 1.7 m3. Therefore, the calculated hydraulic retention time (HRT) was approximately 1.8 days, which is significantly less than conventional recommendations of four to seven days (Kadlec & Wallace, 2009). The organic loading rate averaged 15.3 g BOD5/m2.d and was also relatively high when compared to recommended rates of 4-8 gBOD5/m2.d (EPA, 2000; Burgos et al., 2017). The high hydraulic and organic loading rates used in this study proved to be land conserving, but eventually provided challenges in year II related to cell clogging and short-circuiting.
Water Quality Dynamics
Tables 1 and 2 and Figure 2 provide summary information related to design criteria, water quality and treatment dynamics. Anoxic / anaerobic conditions prevailed in all treatment cells during the study, as evidenced by high NH4+-N concentrations (>30 mg/L) and low DO conditions (< 0.2 mg/L). High hydraulic and organic loading rates encouraged high respiration rates leading to anoxic conditions, poor nitrification and no a NH4+-N removal (Table 2). Chronically low DO concentrations and low nitrification rates have been commonly reported for horizontal sub surface flow systems (EPA, 2002; Zhang et al., 2015). Where required, enhanced rates of nitrification and denitrification can be achieved in SSF wetlands by decreasing the depth of the system (Garcia et al., 2014), although it decreases the HRT. However, these design changes often require additional land and capital expenses which may not be justified (EPA, 2002). Poor removal of phosphorus during the second year was also evident (Figure 2), and was most likely related to rapid saturation of gravel micro-sites (Drizo et al., 1999). The stable tropical temperature regime observed in this study (near 26 ºC), was viewed as being beneficial as it provided year-round opportunities for plant production (Trang et al., 2010). pH values were relatively stable throughout the system, and always less than 7 which helped to minimize losses of ammonia to volatilization. Electrical conductivity values increased progressively throughout the system, probably due to high rates of evapotranspiration and release of nutrients and elements via organic mineralization (Coleman et al., 2001) Scirpus validus L., and Typha latifolia L.. The pH and electrical conductivity values range within Colombian environmental standards for agricultural wastewater (Resolution 1207 of 2014).
Table 1 Average conditions observed for the pilot-scale system during eight months of monitoring. Average values for horizontal subsurface-flow wetlands (SSF) for the period Oct/09 to May/10. The standard deviation is shown in brackets, N=8
Influent (Septic tank) |
Cell 1 | Cell 2 | Cell 3 | Cell 4 | Cell 5 | Effluent (SF-Reservoir) |
|
---|---|---|---|---|---|---|---|
D.O.(mg/L) | 0.15 (0.16) |
0.15 (0.17) |
0.12 (0.12) |
0.12 0.09) |
0.18 (0.19) |
0.14 (0.17) |
0.19 (0.28) |
Temp. (C) | 25.8 (1.6) |
25.6 (1.9) |
25.5 (1.6) |
25.4 (1.0) |
25.3 (1.4) |
26.2 (2.1) |
25.8 (1.6) |
pH | 6.5 (0.2) |
6.5 (0.2) |
6.6 (0.2) |
6.5 (0.2) |
6.6 (0.2) |
6.7 (0.1) |
6.7 (0.1) |
Conductivity, µS/cm | 330.8 (22.6) |
341.8 (37.5) |
356.8 (20.0) |
361.0 (26.2) |
363,5 (21.5) |
376,9 (15,6) |
378,8 (22,0) |
1Flow m3/d | 0.59 (0.44) | ||||||
2Applied organic loading rate (g/m2/day) |
15.3 g BOD5 | ||||||
3Nominal Hydraulic Retention Time (HRT) in hours |
105 | 8.1 | 8.1 | 8.1 | 8.1 | 8.1 | 18.3 |
1. Does not account for losses due to evapotranspiration
2. Organic loading rate is calculated as organic load / total surface area of pilot-scale system (7.7 m2)
3. HRT values (hours), for septic tank, SSF wetlands, surface flow wetland and lagoon-reservoir respectively
Table 2 Concentration averages (mg/L), standard deviations (in brackets) and mean removal efficiencies of the COD, BOD5, ammonium, nitrate, nitrite and ortho-phosphates in the pilot plant in Santa Marta, Colombia four times by month during 8 months. Total and faecal coliform bacteria are expressed in MPN/100 ml. (N= 32)
Variables | Influent | Effluent | % Total removal (SF-Reservoir) |
---|---|---|---|
COD | 326 (176) | 189 (155) | 42 |
BOD5 | 124 (33) | 73 (32) | 41 |
Ammonium | 30 (26) | 32 (21) | - |
Nitrate | 3.0 (3.6) | 1.6 (1.0) | 46 |
Nitrite | 2.8 (2.4) | 2.5 (2.4) | 10 |
Ortho-phosphate | 6.7 (2.5) | 5.9 (2.1) | 10 |
Total Coliforms, NMP/100 ml | 7406 (1980) | 1382 (471) | 81 |
Faecal Coliforms, NMP/100 ml | 365 (125) | 198 (69) | 46 |
Influent COD concentrations (326 mg/L ±176), BOD5 (124 mg/L ± 33), and nutrients were within the ranges reported for septic tank effluents (Crites & Tchobanoglous, 1998; EPA, 2000). COD and BOD5 removal efficiency were modest and progressive throughout the system (Figure 2) and averaged 42 and 41% respectively. These levels of removal are low for the tropics as compared to other studies in which values ranged from 54 to 85% ( Machado, et al., 2016; Caselles et al., 2017) since they can remove a broad range of contaminants by a combination of physical, chemical and biological processes with a low cost. Therefore, CWs can be successfully applied for decentralized wastewater treatment in regions with low population density and/ or with large land availability as Brazil. The present work provides a review of thirty nine studies developed on CWs implemented in Brazil to remove wastewater contaminants. Brazil current sanitation data is also considered to evaluate the potential role of CWs as decentralized wastewater treatment. Performance of CWs was evaluated according to (i. It is inferred that the modest removal rates in this study were influenced by the relatively high hydraulic and organic loading rates. NH4+-N and PO4−3-P removal within the system (Figure 2) were very low and in fact ammonium concentrations increased within the system. This behavior is not uncommon in subsurface-flow wetlands (EPA, 2000) and can be explained on the basis of poor nitrification due to anoxic conditions, while the addition of new ammonium in cells 4 and 5 resulted from mineralization of stored organic matter. During year I, Caselles et al. (2008) reported moderate levels of nitrification and NH4+-N removal, but this was during system start up and before anoxic conditions and cell clogging became chronic. (NO3−-N) and (NO2−-N) concentrations remained less than 3 mg/L, and were probably controlled by a lack of nitrification and near optimum conditions for denitrification.
Nitrate and coliform effluent concentration values were low and in compliance with Colombian environmental standards for agricultural wastewater. (Resolution 1207 of 2017). Removal of coliform bacteria was progressive throughout the system (Figure 2), and averaged 46 and 81% for fecal and total coliforms, respectively. It is anticipated that the short hydraulic retention time (< 2 days), did not allow for higher coliform removal rates.
It is know that in small human settlements, the relationship between mean flows and peak flows is higher than in medium and big cities (Crites & Tchobanoglous, 1998). These higher flow variations affected the organic load variations and pollutants concentrations, as COD and nitrogen species (Table 2).
Although the process of clogging was not monitored, it was especially evident in cell 1 where water surfaced and caused short-circuiting. Eventually, during the course of the study, cell 1 was cleaned out in Dec. 2009 and returned to service. Clogging is a chronic problem in SSFW ( Knowles et al., 2011; Corbella et al., 2016) leading to surface ponding, odors, mosquitoes and short circuiting. Mechanical and non-invasive techniques have been evaluated for remediating clogged cells (Pedescoll et al., 2012), and additional studies will be required to validate cost-effective remediation strategies. Note however, that several studies have reported no loss of treatment efficacy, even with severe clogging (Drizo et al., 1999; Caselles & García, 2007; Aiello et al., 2016;). In retrospect, it is surmised that clogging phenomena could have been avoided by installing a shallow surface-flow wetland as the first treatment cell in the series. This would have allowed much of the particulate organic matter in the septic tank effluent to settle in the surface-flow wetland, thus removing some of the particulate load, and delaying clogging in the following sub-surface flow wetland cells (Behrends et al., 2007)
Tomatoes production
Although the influence of tomatoes plants on nutrient removal was not representative, this does not detract from the potential for producing valuable crops that are adapted to saturated conditions. It is possible that growth in waterlogged soils is achieved by exploiting the availability of nutrients. Traditionally tomato cultivation reaches is completed in three months (Ricardo et al., 2013). Tomatoes were cultivated in cells 2 to 5, and resulted in production of 2.2 kg of tomatoes fruit (Figure 3), it is 0.73 Kg/m2. Annual production of tomatoes in a traditional crop in one tropical country like Colombia, is on average, 3.7 kg/m2 (Agronet, 2014). Cirelli et al., (2012), reported a maximum tomato crop of 8.97 Kg/m2 during a two-year trial of tertiary-treated municipal wastewater use in tomato crop irrigation. Our results are lower than those of Cirelli et al., (2012). We produced tomatoes in just four months.

Figure 3 Total fresh weight of tomatoes fruit (g/cell) in SSF CW. Note that Cell 1 was not planted due to clogging and cell 3 productions were negligible for unknown reasons
Although production in the experiment of this study is lower respect a traditional crop, the hydroponic conditions could affect the total production. Growth performance and crop yield of tomato plants were studied by Rana et al. (2011) with different wastewater concentrations. These authors reported that maximum crop production was recorded at 100% wastewater. The Cell 3 production was nil, probably due to not enough oxygen to nitrification of ammonia and (NH4+-N) high levels (>30 mg/L), which could be toxic for tomatoes (Martínez et al., 2013). Additionally, tomatoes prefer nitrate as a source of nitrogen and may explain the zero growth of tomatoes in cell 3 where the plants were short and thin and produced no fruits (Figure 4).

Figure 4 Picture of the pilot plant with planted tomatoes. Note growth of tomatoes in most cells except cell 3
Soil conditions in HSSF constructed wetlands can limit use of terrestrial plants, like tomatoes. Nevertheless, several studies have shown that some plants adopt strategies to grow in stress conditions (Hough et al., 2015). One the major biological consequences of soil flooding is oxygen deficiency. According with Lin et al. (2004), most tomato varieties are not able to tolerate flooding stress, but they have evolved a complex antioxidative defense system composed of both antioxidant enzymes and metabolites to survive oxidative stress. Then, it may be possible to utilize wastewater treatment in CW for the production of crop tomatoes.
Testing tomatoes for coliform bacteria indicated that there was negligible contamination of tomatoes fruits and suggests that with care, treated wastewater can be used for cultivating and drip-irrigating vegetable crops. Al-Lahham et al. (2003) reported similar results in tomatoes drip irrigated with treated wastewater, but with contamination on the tomato skin. These authors suggested that the treated wastewater can be used as an alternative for irrigation, and that the tomatoes can be eaten after cooking.
Finally, the experiments showed that treating wastewater with CW could become a profitable business in rural areas with little space limitation; instead, the occupied surface could generate income by tomatoes production.
Conclusions
The concept of using natural treatment systems and irrigation of treated wastewater to enhance ecosystem sustainability is not novel (Gatta et al., 2015). Nonetheless, the system deployed in the present study deviated significantly from conventional wastewater treatment goals, which continue to emphasize maximizing removal of organic matter and nutrients with little or no regard for reuse of water and residual nutrients. This study was conducted to evaluate the potential for using integrated constructed wetland technologies for sustainably treating sanitary wastewater while conserving valuable fertilizer nutrients and producing a tomato crop. It is further proposed and envisioned that these nutrient-rich effluents can be reused to irrigate gardens, landscape plantings and various other high-value crops. During year II of this study, the integrated treatment system provided moderate removal of COD and BOD5 (≈43%) and coliform bacteria (>45%). The system effectively conserved fertilizer nutrients, which we found to be a positive attribute in terms of potential for sustainable irrigation and crop production. The integrated system produced a crop of tomatoes that were eaten by the farm residents who found the tomatoes to be tasty and with no ill effects. With respect to sustainability issues, we surmise that use of residual organic matter and nutrients may be cost effective for on-site irrigated systems. It is concluded that production of tomatoes or other valuable crops using treated wastewater can be sustainable with respect to reducing pollution, improving water quality and augmenting the diet and income of farm residents.