Services on Demand
Journal
Article
Indicators
-
Cited by SciELO
-
Access statistics
Related links
-
Similars in SciELO
Share
Agrociencia
On-line version ISSN 2521-9766Print version ISSN 1405-3195
Agrociencia vol.51 n.6 Texcoco Aug./Sep. 2017
Crop Science
THE EFFECT OF MESOCLIMATE ON THE RIPENING OF NEBBIOLO GRAPES (Vitis vinifera) IN VALLE DE VALLE DE GUADALUPE, BAJA CALIFORNIA, MEXICO
1Instituto de Investigaciones Oceanológicas, Universidad Autónoma de Baja California, Carretera Transpeninsular Ensenada-Tijuana #3917, Ensenada, Baja California 22860, México acabello@uabc.edu.mx
The physiology of vines (Vitis vinifera) is regulated by the mesoscale and large-scale climatic conditions. Valle de Guadalupe is a winegrowing basin where most of Mexico’s wine production takes place and presents climatic variations in mesoscale that could influence the grape ripening. The objective of this study was to evaluate the effect of climatic differences on the ripening of the Nebbiolo grape variety, in two winegrowing areas of Valle de Guadalupe. Due to the distance from the coast, finding climatic differences in the valley and in the grape ripening in the study areas was to be expected. Over the course of four seasons, soluble solids concentration, titratable acidity, and pH were evaluated in grapes from the central zone of Valle de Guadalupe and the area nearest to the sea, San Antonio de la Minas (SAM), Baja California, Mexico. In addition, temperature, relative humidity, irradiance, rainfall, wind speed, and wind direction were evaluated from 2012 to 2015. The soluble solids concentration and pH were significantly higher in the Guadalupe grapes’ must than in SAM grapes during the four seasons. On the contrary, the concentration of organic acids always was lower in the must of Guadalupe grapes than in the SAM’s. The irradiance was similar in both areas, but the daily average temperature and the number of days with extreme temperatures were higher in Guadalupe. On the contrary, relative humidity and precipitation were higher in SAM. The early ripening of Guadalupe’s Nebbiolo grapes seems to be the result of higher average temperatures, frequent warm periods, and lower relative humidity in Guadalupe compared with SAM.
Key words: mesoclimate; temperature; Nebbiolo; Valle de Guadalupe; viticulture; Vitis vinifera
La fisiología de la vid (Vitis vinífera) está regulada por las condiciones climáticas de mesoescala y gran escala. El valle de Guadalupe es una cuenca vitivinícola que produce la mayoría del vino de México y presenta variaciones climáticas en mesoescala que podrían influenciar la maduración de las uvas. El objetivo de este estudio fue evaluar el efecto de las diferencias climáticas en dos zonas vitivinícolas del Valle de Guadalupe, sobre la maduración de la uva variedad Nebbiolo. Debido a la distancia con respecto a la costa, se esperaban encontrar diferencias climáticas en el valle y en la maduración de las uvas en las zonas de estudio. Durante cuatro temporadas, se evaluó concentración de sólidos solubles, acidez titulable y pH de uvas de la zona centro del Valle de Guadalupe y de la zona más cercana al mar, San Antonio de la Minas (SAM), Baja California, México. Además, se evaluó la temperatura, humedad relativa, irradiancia, precipitación pluvial, y velocidad y dirección del viento de 2012 al 2015. La concentración de sólidos solubles y el pH fueron significativamente mayores en el mosto de las uvas de Guadalupe que en las de SAM en las cuatro temporadas. Al contrario, la concentración de ácidos orgánicos fue siempre menor en el mosto de las uvas de Guadalupe que en las de SAM. La irradiancia fue similar en ambas zonas, pero la temperatura diaria promedio y el número de días con temperaturas extremas fueron mayores en Guadalupe. Al contrario, la humedad relativa y la precipitación fueron mayores en SAM. La maduración temprana de las uvas Nebbiolo de Guadalupe parece que es resultado de las temperaturas promedio mayores, los períodos frecuentes de calor y la humedad relativa menor en Guadalupe respecto a SAM.
Palabras clave: mesoclima; temperatura; Nebbiolo; Valle de Guadalupe; viticultura; Vitis vinífera
Introduction
The identification and description of diverse environments in different scales is fundamental for the wine making industry in Mexico and the world. The climate of winegrowing regions (especially temperature) plays a fundamental role in the physiology of vine and wine quality (Winkler et al., 1974). Differences of 1 to 3 °C in the environmental average temperature in the Pinot noir and Riesling grape vineyards in New South Wales, Australia, resulted in photosynthetic rates and vines growth differences (Hendrickson et al., 2004). In this study, vines exposed to higher temperatures showed C incorporation rates and growths up to 10-40 % higher than vines exposed to lower temperatures. These differences in C incorporation affected the concentration of soluble solids and organic acids in the grapes, and, at the same time, they modified the quality of the wines produced with those grapes.
In addition to seasonal climatic changes, the weather -in an intermediate or mesoclimate geographic scale- also affects the physiology of native plants and agricultural crops (Holden and Brereton, 2004). The mesoclimate or regional weather (<50 km) is the climate that dominates a growing area or district, an agricultural valley or a forest, and it is influenced by hills, land use, bodies of water, air movement, vegetation cover, and cloudiness. These local climatic differences may cause considerable discrepancies in crop phenology in some winegrowing regions. In France, northwestern Bordeaux receives less rainfall than its southeastern region, which generates wide differences in the number of frosts in the year (Lecocq and Visser, 2006; Bois et al., 2008). The climatic differences in Bordeaux are largely responsible for the great variability of wine types and qualities in the region (Lecocq and Visser, 2006).
Valle de Guadalupe in Baja California, Mexico, is more than 20 km long. No studies have evaluated whether regional climatic differences have or have not an effect on the ripening of the grapes grown there. Valle de Guadalupe is the main winegrowing valley of Baja California and Mexico, where approximately 1800 ha of grapes are grown for wine production. There, like in other winegrowing valleys of Baja California, numerous grape varieties can be found. In 2011, more than 50 wine grape varieties and more than 200 grape producers were registered in the winegrowing valleys of Baja California (Sepulveda-Betancourt, 2009; Secretariat of Agricultural Development, 2011). All varieties are exposed to climatic variability within and between the winegrowing valleys. Although Valle de Guadalupe is Mexico’s main winegrowing area, there is no record of the climate effect on the grape ripening in this area, and little about its weather.
Valle de Guadalupe is 10-30 km away from the Pacific Ocean coast, so some of its regions are exposed to fogs and sea breezes, which generates relatively mild temperatures throughout the year. The sea breezes that reach vineyards near the coast have a significant effect on the physiology of the vines and grape ripening (Nemani et al., 2001; Bonnardot et al., 2002). In Napa and Sonoma, California, USA, a decrease in the number of frosts was observed during the spring due to the change in breeze circulation patterns. This was the result of temperature increase in the adjacent sea and atmospheric water steam concentration, due to global climate change. This decrease in frosts in the growing areas caused an approximate reduction of 20 days in the budbreak season, an increase in the vineyard yield and in the wine quality of the area (Nemani et al., 2001).
The Nebbiolo grape variety for wine production is native to the north of Italy (Giovanelli and Brenna, 2006). This variety is planted in all the valleys of Baja California and valued by winemakers, because of the high quality of the wine that they obtain.The objective of this study was to evaluate the effect of climatic variability in Valle de Guadalupe, Baja California, on the ripening of the Nebbiolo grape. The hypothesis was: due to the climatic variation in Valle de Guadalupe, there are differences in the ripening of the Nebbiolo grapes grown in the valley’s inner zone and the area closest to the coast.
Materials and Methods
Study area
Valle de Guadalupe (32° 03’ N, 116° 37’ W) is a basin, located at approximately 30 km northeast of Ensenada, Baja California, Mexico (Figure 1). San Antonio de las Minas (SAM) is located in the western area of the valley, approximately 10 km from the Pacific Ocean coast. The town of Guadalupe (Francisco Zarco) is located in the eastern region of Valle de Guadalupe, approximately 30 km from the coast. Valle de Guadalupe is classified as a semi-arid region, and the highest precipitation levels are recorded in winter (285 mm year-1).
Weather data
From September 2012 to December 2015, weather data (ambient temperature, relative humidity, photosynthetically active radiation, wind speed and wind direction) were recorded every 15 min, in the meteorological stations (HOBO U30, USA) located in each vineyard included in the study, both in Guadalupe (32° 06’ 50’’ N, 116° 30’ 47’’ W) and in SAM (31° 59’ 05’’ N, 116° 38’ 38’’ W). The linear distance among the vineyards was approximately 20 km. Station sensors were placed 2.5 m above the ground, where they were directly exposed to solar radiation and wind.
Cultivation of the vines
The study included four production periods (2012-2015), in commercial vineyards with Nebbiolo grapes. The vegetative material of both varieties came from the same clone and was 5 years old at the beginning of the study. The vines were grown on unilateral cordon and a two-wire trellis system. The distance between corridors and between vines was 2.7 m and 1.2 m, respectively; the orientation was east-west and pruning was maintained at 30 buds per vine. Both study areas featured an alluvial sandy loam soil. Drip irrigation (20 L) was supplied weekly. The soluble solids content in the irrigation water was less than 600 mg L-1 in the irrigation period and in both growing zones.
Chemical and statistical analysis
The grape maturity variables were determined weekly from the veraison date. Between 200 and 300 berries were collected randomly, weekly, in each study area, and transported -in a cooler with ice gel- to be analyzed in the laboratory. The grapes were harvested in both areas of the corridors, internal and external canopy, and upper and lower bunches. The grapes were macerated for 1 h and the soluble solids concentration was determined with a Fisher Scientific™ Abbe Bench-top Refractometer. The titratable acidity was quantified by titration with 0.1 N NaOH and the pH of the must was determined using a potentiometer (ThermoOrion 410) (Zoecklein et al., 1995).
Statistical differences of weather and grapes data were determined with one-way ANOVA, after testing the normality and homoscedasticity of the data (Sokal and Rohlf, 1995). All the multiple comparisons of means were carried out using Tukey’s multiple comparison test (p≤0.05).
Results and Discussion
The ripening of grapes for wine production is a complex process involving the accumulation of glucose, fructose, organic acids, polyphenols and aromatic substances, and the reduction of some compounds that give unwanted bouquets to wines. The synthesis and catabolism of these compounds in grapes are closely regulated by environmental factors and cultivation practices that influence the typicity and quality of wines. Annual and mesoscale climatic variations modify the organoleptic properties and the quality of the wines from one production year to another in each region. This may explain the different ripening between vines in areas with different microclimates within Valle de Guadalupe that we observed in this study.
The soluble solids content in the four samples was significantly higher (p≤0.01) in the Guadalupe Nebbiolo grapes compared with SAM’s (Figure 2). At the beginning and at the end of the ripening, the soluble solids concentration was higher (p≤0.05), from 3 to 5 and more than 20 °Bx, in the Guadalupe grapes compared with SAM’s. The highest values of Guadalupe grapes were reached one to three weeks earlier (p≤0.05) than in SAM’s. The titratable acidity of the four samples was 50 % lower (p≤0.05) at the beginning of the ripening in the Guadalupe grapes than in SAM’s and remained like that during the ripening. The pH of the Guadalupe grape must was significantly higher (p≤0.05) than SAM’s. The higher rates of soluble solids incorporation and the faster catabolism of organic acids in Guadalupe grapes, compared with SAM’s, matches the differences in ripening rates observed in many winegrowing valleys in the world. These differences in ripening are generally linked to mesoscale or large-scale climatic differences. This is the case of Carignan, Petite sirah, and Zinfandel grapes in which the incorporation of soluble solids is more accelerated in the warm areas than in the cold areas of California, USA (Amerine and Winkler, 1944; Winkler et al., 1974). The soluble solids concentration in these grapes was 2 to 5 °Bx higher than in the cold areas of California and the organic acids concentration was double than those of cold areas (Amerine and Winkler, 1944). In addition, in warm areas -or in years with higher maximum temperatures during the ripening period-, the soluble solids concentration increases rapidly and the Sangiovese, Sauvignon blanc, and Nebbiolo grapes harvest is accelerated (Conradie et al., 2002, Guidoni et al., 2008, Filippetti et al., 2015). From the commercial point of view, the concentration of soluble solids and organic acids that the grapes have reached maturity and is used to identify the date of harvest and start the fermentation. As a result of the difference in the ripening rates of these grapes harvest takes place up to two months earlier in the warm areas of California than in the cold ones. This difference in the harvest time was also observed in this study, which in Guadalupe takes place earlier (up to 27 days) than in SAM.

Figure 2 Soluble solids concentration, titratable acidity, and pH of Nebbiolo grapes during ripening, in Guadalupe and San Antonio de las Minas, Baja California, Mexico.
In both study sites, no significant differences were observed in the irradiance received by the vines (p≤0.05) (Figure 3). In both years, the highest daily irradiances (65 mol quanta m-2 d-1) were recorded in July, and the lowest (3-6 mol quanta m-2 d-1) between November and March. Although the maximum daily irradiance was similar in both sites, the number of days with low irradiance due to coastal fog or clouds was higher (p≤0.05) in SAM. The winegrowing area of SAM is located 10 km from the coast and receives the influence of recurrent coastal fog. In contrast, the westernmost part of Valle de Guadalupe is located 30 km from the coast and is not as frequently affected by the fog. The reduction of irradiance in SAM is consistent with the reduction of irradiance in the coastal zone of southern California due to the fog effect (Tont, 1975; Fischer et al., 2009). This proved that there is a linear reduction of the irradiance from the coast to the inland, as a consequence of the fog in the coastal zone. The frequency of fog in southern California, and probably northern Baja California, has decreased significantly over the past 60 years, as a result of changes in circulation patterns (Laochy and Witiw, 2011). These changes in fog patterns have affected coastal ecology and agriculture in these areas, including winemaking (Nemani et al., 2001).

Figure 3 Photosynthetically active radiation (mol cuanta m-2 d-1) in Guadalupe and San Antonio de las Minas, Baja California, Mexico.
The daily average temperature patterns were similar in both sites (p≤0.05), but the number of days with average temperatures higher than 25 °C was 131 in Guadalupe and only 67 in SAM (p≤0.05, Figure 4). The average growth temperature is one of the factors that regulates the grapes’ maturity. In Navarre, Spain, the ripening of tempranillo grapes was more accelerated in vines grown at 28 °C than those grown at 24 °C (Salazar Parra et al., 2010). The soluble solids concentration reached 24 °Bx significantly sooner when the vines grew at 28 °C compared with those that grew at 24 °C. The lower titratable acidity and higher pH values of Guadalupe’s Nebbiolo grape -with regard to SAM grapes- were also a result of higher temperatures in the valley’s inner zone. Higher temperatures reduce titratable acidity levels and consequently increase the pH of the must (Winkler et al., 1974, Tarara et al., 2008). Tartaric and malic acids make up about 90 % of the grapes’ organic acids. The concentration of tartaric acid is relatively stable throughout grape ripening, but the malic acid is actively catabolized and consumed in respiration, throughout the Krebs cycle. The malic acid catabolism increases in grapes developed in warmer climates; therefore, their concentration decreases (Winkler et al., 1974, Lakso and Kliewer, 1975). In addition, the grape growth temperature affects its ripening (soluble solids, titratable acidity, pH) and the accumulation of molecules that regulate the quality of the wines produced with them. For example, the concentration of methoxypyrazines increases in Cabernet sauvignon and Carmenere grapes when growth temperatures are low (Belancic and Agosin, 2007; Falcão et al., 2007). The accumulation of methoxypyrazines in grapes growing in cold areas gives red pepper, hay, and other “green” bouquets to the wines produced with them (Falcão et al., 2007). These bouquets -associated with methoxypyrazines- are considered negative and reduce the wine’s quality perception. This suggests that the differences in average temperatures in SAM and Guadalupe affect the ripening rates of the Nebbiolo grape and probably other grapes. In addition, the mesoclimate differences between the two zones likely influenced the accumulation of soluble solids and organic acids, and also on the final concentration of molecules, such as methoxypyrazine, which modify the quality of wine.

Figure 4 Daily air average temperature in Guadalupe and San Antonio de las Minas, Baja California, Mexico.
The maximum temperature values were slightly higher in Guadalupe than in SAM (Figure 5). The number of days with temperatures higher than 35 °C was three times greater in Guadalupe (118 d) than in SAM (37 d) (p≤0.05). In addition, during this study period, no temperatures higher than 40 °C were recorded in SAM, while there were 10 days with such temperatures (p≤0.05) in Guadalupe. Therefore, Guadalupe grapes were more frequently exposed to extreme temperatures than SAM’s. On the contrary, the minimum temperature was relatively similar in both winegrowing valleys: 83 days and 76 days with temperatures under 5 °C, in Guadalupe and SAM, respectively. The production of high quality wine is limited to climate regions which: 1) maintain a thermal accumulation that suitably expresses the character of the grape variety; 2) present low risk of frost damage to vines; and, 3) are not subject to extreme temperatures (White et al., 2006). The results of our study indicate that Guadalupe grapes are more frequently exposed to extreme temperatures -and, therefore, to a greater dehydration- than SAM’s. The higher temperatures and the greater number of days with temperatures above 35 °C in Guadalupe increased the soluble solids concentration, decreased the titratable acidity in the must -as a result of the greater dehydration-, and increased the malic acid catabolism. Likewise, an increase of maximum temperatures was observed during the period of study, mainly in SAM. It is likely that this increase in the time series was influenced by the extreme temperatures experienced globally during 2014 and 2015, including northern Baja California. This increase in temperature is probably linked to large-scale climate changes and extended periods (Kilmister et al., 2016; Mann et al., 2016).

Figure 5 Daily maximum (black circles) and minimum (white circles) temperatures in Guadalupe and San Antonio de las Minas, Baja California, Mexico.
The difference between the maximum and minimum daily temperatures (amplitude) was relatively similar in both winegrowing valleys (Figure 6). A daily temperature difference between 3 and 26 °C was observed throughout the year in both valleys, without a recognizable seasonal pattern. The difference in the amplitude of the range of maximum and minimum temperature fluctuations remained constant during the study period in SAM. In contrast, there was a decrease of approximately 2 °C in Guadalupe, in the amplitude between the maximum and minimum daily temperatures. The lower temperature difference between day and night is consistent with the decrease of diurnal amplitude in southern California, as a result of to global climate change over the past 100 years (Steve et al., 2007). The concentration of some grape metabolites is mainly regulated by the ripening stage, soil type, light, cultivation method, ambient temperature, and other factors. The increase in the growth temperature of the Merlot grape reduced the pigments concentration in the grape’s skin and the concentration of total polyphenols (Spayd et al., 2002). In addition, exposing grapes to extreme temperatures during ripening -even for short periods- modifies their polyphenolic and anthocyanic profile (Tarara et al., 2008). Therefore, the differences in the extreme temperatures between Guadalupe and SAM could influence the soluble solids concentration and titratable acidity in the grapes, resulting in differences in the polyphenolic composition and the bouquet of grapes from both valleys.
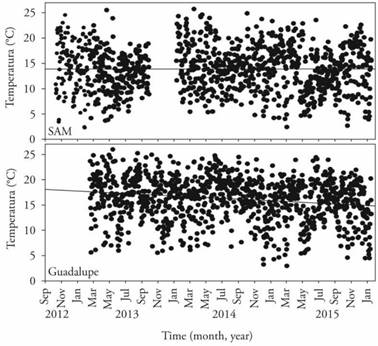
Figure 6 Daily temperature amplitude range in Guadalupe and San Antonio de las Minas, Baja California, Mexico.
During the study, the relative humidity was higher (p≤0.01) in SAM than in Guadalupe (Figure 7). The average relative humidity was approximately 70 % and less than 60 % in SAM and in Guadalupe, respectively. In addition, there were three more days in which relative humidity was higher than 80 % in SAM than in Guadalupe. Relatively higher humidity values in SAM, with regard to Guadalupe, match higher humidity values throughout the coastal zone of California, in relation with the central area of the state. The high levels of relative humidity near to the coast is the result of the sea water evaporation and the sea breezes towards the coast. Relative humidity has a critical role in foliar and fruit transpiration in numerous species, including vines. Stomatal conductance and photosynthesis increase with relative humidity (Mooney et al., 1983). On the contrary, the decrease in relative humidity increases the transpiration rates of grapes, as a consequence of which soluble solids concentration of the must increase with dehydration (Rebucci et al., 1997). Therefore, the increase in the soluble solids accumulation rates (ripening) of the Guadalupe grapes, in relation to SAM’s, must be the partial result of lower relative humidity throughout the year.

Figure 7 Daily average relative humidity in Guadalupe and San Antonio de las Minas, Baja California, Mexico.
Winter rainfall was responsible for most of the annual precipitation in Valle de Guadalupe (p≤0.05, Figure 8). In addition, precipitation variability in was observed from one year to another, during our study: the lower temperatures were recorded in both areas during the winter of 2013-2014. Because there are no perennial streams or rivers in the area, the recharge of Valle de Guadalupe’s aquifer depends entirely on winter rainfall (Campos-Gaytan et al., 2014). Rains in southern California, USA, and the north of Baja California, Mexico, are a consequence of the weakening of the North Pacific system in winter, which allow convective systems and rain fronts to enter this region (Cavazos and Rivas, 2004). The interannual rainfall variability in northern Baja California is mostly the result of the effect of El Niño events, such as the one observed in 2015 (Schonher and Nicholson 1989, Cavazos and Rivas 2004, Varotsos et al., 2016). As a result of interannual variability -and because most rainfall occurs in winter-, irrigating the vines is necessary to mitigate the increase of evapotranspiration and water stress in these crops during the summer (Mendoza-Espinosa et al., 2008, Acosta-Zamorano et al., 2013a and 2013b).

Figure 8 Monthly rainfall in Guadalupe and San Antonio de las Minas, Baja California, Mexico, and correlation of monthly rainfall in both areas
Regression analysis of rainfall data in both study areas indicated that precipitation was approximately 30 % greater (p≤0.05) in SAM than in Guadalupe. This contrasts with the slight precipitation increase from the coast towards the inland, and the increase of topographic height in northern Baja California reported by Minnich et al. (2000). SAM is near the coast, immediately after an abrupt increase in coastal topography (Figure 1). Guadalupe shares the same topographic height as SAM, but is located 20 km inland. Therefore, it is likely that greater discharges of water occur in SAM, as a result of the rain fronts. Higher rainfall in SAM probably does not have an immediate impact in grape ripening in both regions. The reduction of more than 20 m of the water table in the Guadalupe area -as a consequence of water extraction in recent years and the current drought in the region- has exceeded the recharge (Campos-Gaytan et al., 2014, Robeson 2015). Major declines in water table have been observed in Valle de Guadalupe’s inner zone, and levels have remained relatively constant in the area closest to the coast. The lower depth of the water table in crops near the coast probably provides more moisture in the radicle area and causes slow rates of grape ripening. In addition, higher rainfall in SAM can generate higher recharge in the region of the valley closest to the coast.
Significant differences (p0.05) were observed in temperature, relative humidity, velocity and direction of the dominant Guadalupe and SAM winds, during the grape ripening period (Figure 9). The dominant winds during the day (10:00 a.m. to 2:00 p.m.) in SAM blew in a southwest direction, with intensities generally lower than 4 m s-1; in Guadalupe, they blew in the same direction, but they were significantly more intense than in SAM. On the contrary, the afternoon and night winds blew in a north direction in SAM, while Guadalupe, they blew towards the east. In addition, the intensity of the evening/night winds was slightly stronger in SAM than in Guadalupe. The evapotranspiration process depends greatly on wind speed and turbulence in the leaf boundary layer and the grape clusters. Higher wind speeds reduce the thickness of the boundary layer and increase the plants’ evapotranspiration, which in turn, increases water stress (Onoda and Anten, 2011). Therefore, the greater intensity in the wind speed likely causes greater dehydration and water stress in Guadalupe grapes than in SAM’s.
Conclusions
Valle de Guadalupe is an extensive winegrowing region that presents mesoscale climatic variations. The Guadalupe area has higher wind speeds, and greater average and maximum temperatures than San Antonio de las Minas. Relative humidity and rainfall were lower in Guadalupe than in SAM. The climatic conditions differences seem to accelerate the accumulation of soluble solids and the titratable acidity reduction of the Guadalupe Nebbiolo grape compared with SAM’s. Perhaps this effect on ripening is also regulating the ripening and the physiological features of other grapes in the valley. Ripening differences are likely to cause differences in the quality of the region’ grapes and wines
Literatura Citada
Acosta-Zamorano, D., V. Macias-Carranza, L. Mendoza-Espinosa, and A. Cabello-Pasini. 2013a. Effect of treated wastewater on growth, photosynthesis and yield of tempranillo grapevines (Vitis vinifera) in Baja California, Mexico. Agrociencia 47: 753-766. [ Links ]
Acosta-Zamorano, D., V. Macías-Carranza, L. Mendoza-Espinosa , andA. Cabello-Pasini. 2013b. Effect of treated wastewater on the chemical composition of Tempranillo grapes (Vitis vinifera) in Baja California, Mexico. Agrociencia 47: 767-779. [ Links ]
Amerine, M. A., and A. Winkler. 1944. Composition and quality of musts and wines of California grapes. Hilgardia 15: 493-675. [ Links ]
Belancic, A., and E. Agosin. 2007. Methoxypyrazines in grapes and wines of Vitis vinifera cv. Carmenere. Am. J. Enol. Vitic. 58: 462-469. [ Links ]
Bois, B., C. VanLeeuwen , P. Pieri, J.-P. Gaudillère, E. Saur, D. Joly, L.Wald, and D. Grimal. 2008. Viticultural agroclimatic cartography and zoning at mesoscale level using terrain information, remotely sensed data and weather station measurements. Case study of Bordeaux winegrowing area. VIIth International terroir Congress. Changins, Switzerland. pp: 455-462. [ Links ]
Bonnardot, V., O. Planchon, V. Carey, and S. Cautenet. 2002. Diurnal wind, relative humidity and temperature variation in the Stellenbosch-Groot Drankenstein wine-growing area. S. Afr. J. Enol. Vitic. 23: 62-71. [ Links ]
Campos-Gaytan, J. R., T. Kretzschmar, and C. S. Herrera-Oliva. 2014. Future groundwater extraction scenarios for an aquifer in a semiarid environment: case study of Guadalupe Valley Aquifer, Baja California, Northwest Mexico. Environ. Monit. Assess. 186: 7961-7985. [ Links ]
Cavazos, T., and D. Rivas. 2004. Variability of extreme precipitation events in Tijuana, Mexico. Clim. Res. 25: 229-243. [ Links ]
Conradie, W.J., V.A.Carey, V.Bonnardot, D.Saayman, and L. H.Van Schoor. 2002. Effect of the different environmental factors on the performance of Sauvignon blanc in the Stellenbosch/Durbanville districts of South Africa. I. Geology, soil, climate, phenology and grape composition. S. Afr. J. Enol. Vitic . 23: 78-91. [ Links ]
Falcão, L.D., G. deRevel , M.C.Perello, A.Moutsiou, M.C.Zanus, and M.T. Bordignon-Luiz . 2007. A survey of seasonal temperatures and vineyard altitude influences on 2-methoxy-3-isobutylpyrazine, C13-norisoprenoids, and the sensory profile of Brazilian Cabernet Sauvignon Wines. J. Agric. Food Chem. 55: 3605-3612. [ Links ]
Fischer, D. T., C. J. Still, and A. P. Williams. 2009. Significance of summer fog and overcast for drought stress and ecological functioning of coastal California endemic plant species. J. Biogeogr. 36: 783-799. [ Links ]
Giovanelli, G., and O. V. Brenna. 2006. Evolution of some phenolic components, carotenoids and chlorophylls during ripening of three Italian grape varieties. Eur. Food Re. Technol. 225: 145-150. [ Links ]
Guidoni, S., A. Ferrandino, and V. Novello. 2008. Effects of seasonal and agronomical practices on skin anthocyanin profile of Nebbiolo grapes. Am. J. Enol. Vitic . 59: 22-29. [ Links ]
Hendrickson, L., M. C. Ball, J. T. Wood, W. S. Chow, and R. T. Furbank. 2004. Low temperature effects on photosynthesis and growth of grapevine. Plant Cell Environ. 27: 795-809. [ Links ]
Holden, N. M., and A. J. Brereton. 2004. Definition of agroclimatic regions in Ireland using hydro-thermal and crop yield data. Agric. For. Meteorol. 122: 175-191. [ Links ]
Kilmister, R., D. Unwin, M. Treeby, E. Edwards, and M. Krstic. 2016. Climate change: effect of elevated CO2 and temperature on phenology, carbohydrates, yield and grape composition - preliminary results. Wine Viticult J. 31: 38-40. [ Links ]
LaDochy, S., and M.Witiw. 2011. The continued reduction in dense fog in the Southern California Region: possible causes. Pure Appl. Geophys. 169: 1157-1163. [ Links ]
Lakso, A. N., and W. M. Kliewer. 1975. The influence of temperature on malic acid metabolism in grape berries: I. Enzyme responses. Plant Physiol. 56: 370-372. [ Links ]
Lecocq, S., and M. Visser. 2006. Spatial variations in weather conditions and wine prices in Bordeaux. J. Wine Econo. 1: 114-124. [ Links ]
Mann, M.E., S.Rahmstorf , B.A.Steinman , M.Tingley, and S. K.Miller. 2016. The likelihood of recent record warmth. Sci. Rep. 6: 19831. [ Links ]
Mendoza-Espinosa, L. G., A. Cabello-Pasini , V. Macías-Carranza , W. Daessle-Heuser, M. V. Orozco-Borbon, and A. L. Quintanilla-Montoya. 2008. The effect of reclaimed wastewater on the quality and growth of grapevines. Wat. Sci. Tech. 57: 1445-1450. [ Links ]
Minnich, R. A., E. Franco-Vizcaino, and R. J. Dezzani. 2000. The El Niño/Southern oscillation and precipitation variability in Baja California, Mexico. Atmósfera 13: 1-20. [ Links ]
Mooney, H.A., C. Field , C.V. Yanes, and C.Chu. 1983. Environmental controls on stomatal conductance in a shrub of the humid tropics. Proc. Natl. Acad. Sci. USA. 80: 1295-1297. [ Links ]
Nemani, R., M.A. White, D.R. Cayan, G. Jones, S. Running, J. Coughlan, and D. Peterson. 2001. Asymmetric warming over coastal California and its impact on the premium wine industry. Clim. Res. 19: 25-34. [ Links ]
Onoda, Y., and N. P. R. Anten. 2011. Challenges to understand plant responses to wind. Plant Signal Behav. 6: 1057-1059. [ Links ]
Rebucci, B., S. Poni, C. Intrieri, E. Magnanini, and A.N. Lakso. 1997. Effects of manipulated grape berry transpiration on post-veraison sugar accumulation. Aust. J. Grape Wine Res. 3: 57-65. [ Links ]
Robeson, S.M. 2015. Revisiting the recent California drought as an extreme value. Geophysical Res. Lett. 42: 2015GL064593. [ Links ]
Salazar-Parra, C., J. Aguirreolea, M. Sánchez-Díaz, J. J. Irigoyen, and F. Morales. 2010. Effects of climate change scenarios on Tempranillo grapevine (Vitis vinifera L.) ripening: response to a combination of elevated CO2 and temperature, and moderate drought. Plant Soil 337: 179-191. [ Links ]
Schonher, T., and S.E.Nicholson. 1989. The relationship between California rainfall and ENSO events. J. Clim. 2: 1258-1269. [ Links ]
Secretaría de Fomento Agropecuario. 2011. Estudio estadístico sobre producción de uva en Baja California. Secretaría de Fomento Agropecuario. Ensenada, Baja California. p. 37. http://www.nacionmulticultural.unam.mx/empresasindigenas/docs/1873.pdf . (Consulta: abril 2016). [ Links ]
Sepulveda-Betancourt, J. I. 2009. Aspectos Geográficos y Estadísticos de la Viticultura del Estado de Baja California. Sistema Producto Vid. Ensenada, Baja California. 26 pp. http://www.afintegral.com . (Consulta: abril 2016). [ Links ]
Sokal, R. R., and F. J. Rohlf. 1995. Biometry. W.H. Freeman and Company. New York. 887 pp. [ Links ]
Spayd, S. E., J. M. Tarara, D. L. Mee and J. C. Ferguson. 2002. Separation of sunlight and temperature effects on the composition of Vitis vinifera cv. Merlot berries. Am. J. Enol. Vitic . 53: 171-182. [ Links ]
Steve, L., M. Richard and P. William. 2007. Recent California climate variability: spatial and temporal patterns in temperature trends. Clim. Res . 33: 159-169. [ Links ]
Tarara, J. M., J. Lee, S. E. Spayd, and C. F. Scagel. 2008. Berry temperature and solar radiation alter acylation, proportion, and concentration of anthocyanin in Merlot grapes. Am. J. Enol. Vitic . 59: 235-247. [ Links ]
Tont, S. A. 1975. The effect of upwelling on solar irradiance near the coast of southern California. J. Geophys. Res. 80: 5031-5034. [ Links ]
Varotsos, C. A., C. G. Tzanis, and N. V. Sarlis. 2016. On the progress of the 2015-2016 El Niño event. Atmos. Chem. Phys. 16: 2007-2011. [ Links ]
White, M.A., N.S. Diffenbaugh, G.V. Jones, J.S. Pal, and F. Giorgi. 2006. Extreme heat reduces and shifts United States premium wine production in the 21st century. Proc. Natl. Acad. Sci. USA 103: 11217-11222. [ Links ]
Winkler, A.J., J.A. Cook, W.M. Kliewer , and L.A. Lider. 1974. General Viticulture. University of California Press. Berkley. 710 p. [ Links ]
Zoecklein, B.W., K.C. Fugelsang, B.H. Gump, and F.S. Nury. 1995. Wine Analysis and Production. Chapman-Hall. New York. 296 p. [ Links ]
Received: May 2016; Accepted: November 2016