Services on Demand
Journal
Article
Indicators
-
Cited by SciELO
-
Access statistics
Related links
-
Similars in SciELO
Share
Agrociencia
On-line version ISSN 2521-9766Print version ISSN 1405-3195
Agrociencia vol.51 n.4 Texcoco May./Jun. 2017
Crop science
Combining ability of inbred lines for maize (Zea mays L.) single cross hybrid production under irrigation
1Postgrado en Recursos Genéticos y Productividad-Genética, Campus Montecillo, Colegio de Postgraduados. Km 36.5 Carretera México-Texcoco. 56230. Montecillo, Texcoco, Estado de México.
2Departamento de Fitotecnia, Universidad Autónoma Chapingo. Km 38.5 Carretera México-Texcoco. 56230. Chapingo, Estado de México.
3Facultad de ingeniería y Ciencias, Universidad Autónoma de Tamaulipas. Centro Universitario Adolfo López Mateos. 87149. Ciudad Victoria, Tamaulipas.
Given that maize (Zea mays L.) displays broad genetic diversity, as part of a breeding program, knowledge of the components of genetic variation is necessary to optimize strategies for their use. Combining ability, maternal effects, type of genetic effects of the crosses and their genetic potential were studied through evaluation of simple mating combinations between 10 lines (S6-S8) to produce single-cross hybrids, under irrigation, in Tecámac and Montecillo, of the Estado de Mexico and in Mixquiahuala, of the State of Hidalgo, in the High Valleys of Central Mexico. The experimental design was a simple 10×10 lattice. Genetic analysis was performed with model I of Griffing Method I. Average grain yield (5.6 t ha-1) showed that phenotypical expression of the lines was outstanding. General combining ability of lines L5, L6, L8, L10 and L9 stood out, although there were contrasts in grain yield. Genetic expression of grain yield for the outstanding crosses in the study was based on two possibilities: 1) that the cross was formed by both high general combining ability (GCA) lines, in which case the effects of specific combining ability (SCA) may or may not be high, and 2) that the cross was achieved with at least one high GCA line whose SCA effects are high. Based on the number of outstanding crosses, the foremost lines that conformed these crosses were L10, L9, L8 and L6. Line L10 was part of almost 50 % of all the crosses (10) and in all cases they functioned as female or as male parental lines. The genetic structure of the crosses showed contrasts, which means that there is a possibility of using some specific genotypes as single-cross hybrids, open pollinated varieties, or incorporated into a recurrent selection scheme to take advantage of additive effects.
Key words: maternal effects; components of genetic variation; productivity; High Valleys of Central México
Dado que el maíz (Zea mays L.) presenta amplia diversidad genética, en un programa de mejoramiento genético es necesario conocer los componentes de variación genética para optimizar las estrategias de aprovechamiento. La aptitud combinatoria, los efectos maternos, el tipo de acción génica de las cruzas y su potencial genético se estudiaron a través de la evaluación de las combinaciones simples entre 10 líneas (S6-S8) desarrolladas para producir híbridos de cruza simple, con riego, en Tecámac y Montecillo, Estado de México, y Mixquiahuala, estado de Hidalgo, en los Valles Altos del Centro de México. El diseño experimental fue látice simple 10×10; el análisis genético se efectuó con el modelo I del Método I de Griffing. El rendimiento medio de grano (5.6 t ha-1) mostró que la expresión fenotípica de las líneas fue sobresaliente. Las líneas L5, L6, L8, L10 y L9 destacaron por la expresión de su aptitud combinatoria general, aunque presentaron contrastes en el rendimiento de grano. La expresión genética del rendimiento de grano para las cruzas sobresalientes en el estudio se fundamentó en dos posibilidades: 1) que la cruza haya sido formada por ambas líneas de ACG alta, en cuyo caso los efectos de ACE pudieron ser altos o no, y 2) que la cruza se realice con al menos una línea de ACG alta, pero cuyos efectos de ACE sean altos. Con base en el número de cruzas sobresalientes, las líneas participantes destacadas fueron L10, que fue parte de casi 50 % de las cruzas (10), L9, L8 y L6, que en todos los casos fungieron como hembra o macho. La estructura genética de las cruzas mostró contrastes, lo que resaltó la posibilidad de emplear específicamente algunos genotipos, como híbridos de cruza simple, variedades de polinización libre o incorporarlos en un esquema de selección recurrente para el aprovechamiento de los efectos de aditividad.
Palabras clave: efectos maternos; componentes de variación genética; productividad; Valles Altos del Centro de México
Introduction
Among mating systems, diallel designs, defined as sets of possible single crosses among a group of n parents, are the mating designs most often used in maize (Zea mays L.). Diallel models were presented by Schmidt (1919; cited by Martínez, 1983) and are used to estimate the components of genetic variation for any quantitative trait (such as yield) of the crosses as well as their productivity (Martínez, 1983).
Diallel crosses are useful tools in the study of biological populations for the estimation of main and interaction gene effects of quantitative traits of agricultural importance and also are used to establish breeding schemes with the aim of selecting superior genotypes (Gardner and Eberhart, 1966; Ávila et al., 2009).
The studies of Sprague and Tatum (1942) and Griffing (1956) have contributed to the frequent use of diallel designs in genetic research, especially that concerning hybrid maize. Sprague and Tatum divided combining ability (CA) into general (GCA) and specific (SCA). CA is the capacity of individuals or a population to combine with others. GCA is the average behavior of a line through its hybrid combinations, and SCA refers to the effect in which certain combinations perform better or worse than expected, relative to the average behavior of the involved parental lines. Regarding the type of gene effects that determine the CA of the lines, GCA is predominantly associated with additive effects, while SCA includes effects of dominance and epistasis interactions (Hallauer et al., 2010). Griffing (1956) systematized the statistical analysis for estimating the variance components when using a diallel crossing design based on GCA and SCA effects.
The complete diallel of interest for our study was formally proposed by Griffing (1956). This researcher defined diallel crosses as mating schemes of a set of p parental lines, with which a maximum of p2 combinations are obtained. These combinations are divided into three groups: 1) the p parents; 2) the p(p-1)/2 direct F1 crosses; and 3) the p(p-1)/2 reciprocal F1 crosses. With these three groups, he developed four methods of diallel analysis, which include i) the possible p2 combinations, II) the parents and direct F1 crosses, III) the direct and reciprocal F1 crosses, and IV) the direct F1 crosses.
The more frequently used Griffing designs are II and IV. Design II excludes the reciprocal crosses, while IV excludes reciprocal crosses and parental lines. However, when for any reason the researcher expects maternal effects, it is indispensable to study them; for this purpose, the basic linear model for the analysis of diallel experiments (Y ijk =µ+g i +g j +s ij +r ij +e ijk ) can be developed even more as: Y ijk =µ+g i +g j +s ij +m i -m j +r ij +e ijk ; where Y ijk is the observed phenotypical value of the cross, with parents i and j, in block k; µ is a common effect in all the observations; g i and g j are the GCA effects of parents i and j; s ij is the effect of the SCA of the cross (i, j); m i and m j represent the maternal effects of the parents (i, j); r ij is the reciprocal effect of the cross (i, j) and e ijk is the random environmental effect corresponding to the observation (i, j, k).
The maternal effect associated with extra nuclear DNA of the female parent is frequently ignored when using the diallel cross system. However, Mahgoub (2011) suggests that the phenotype of an individual can also be determined by this maternal component. One way to quantify this contribution from parents to their descendants is to study reciprocal crosses, of which the complete diallel is the convenient mating system to demonstrate a phenomenon such as this (Martínez, 1983).
Based on the above, the objective of the this study was the assessment of combining ability and maternal effects of a group of inbred lines (S6-S8) through their crosses to determine the type of genetic effects (additive or dominant) for their use in the MMR breeding program (Dr. Moisés Mendoza Rodríguez†) that would permit increasing grain productivity. The hypothesis proposes that there is predominance of non-additive effects over additive effects, as well as the existence of maternal effects and the expression of superior crosses, developed from outstanding lines.
Materials and Methods
All possible combinations of 10 inbred lines (S6-S8) developed for maize production were evaluated under irrigated conditions in the High Valleys of Central México. The study was carried out in the spring-summer 2014 growing season in three locations of this ecological area (Table 1).
Table 1 Locations where diallel crosses were field evaluated.

T: annual mean temperature; Pp: annual precipitation; Alt; altitude; Fs: sowing date and Ds: sowing density.
In the spring-summer growing season of 2013, the 10 maize parental lines were planted in the Agriculture Experimental station of the Colegio de Postgraduados, Campus Montecillo. In 2012 the lines had been previously selected by a topcross test. With these genotypes, the diallel cross scheme was developed. With seed obtained (F1) in 2014, the field evaluation assays were set up in a simple 10×10 lattice experimental design with four replications in three locations (Table 1). The experiment was carried out under only one planting irrigation in Tecámac. The experimental unit was one 5 m long, 0.8 m wide row.
During the growing season and harvest, information on quantitative characteristics associated with phenology, morphology and grain yield was recorded. Values were the average of five ears from each experimental unit. The data were analyzed with Pearson correlation (Restrepo and González, 2007), and traits with higher and positive correlation with grain yield were identified. These traits were primary ear weight (Pmz1), grain weight per ear (Pgr) and ear length (Lmz). These traits were further analyzed for estimation of variance components.
Grain yield per experimental unit (Rto1) was determined with the equation: Rto1=((PMz1+PMz2) X Id X (100 - %Hum)/100)/0.86; where PMz1 and PMz2 were weights of the primary and secondary ears harvested per experimental unit; Id, grain-ear index; % Hum, moisture content of the ear sample in the field, and 0.86, a correction factor to readjust yield to 14 % moisture (Pérez, 2001). With this result and the size of the experimental unit, grain yield (Rto) in t ha-1 was estimated.
Genetic analysis was performed with grain yield using PROC GLM, with Model I (fixed effects) of Method I (complete diallel) of Griffing (1956), considering that parents were selected based on their performance in a topcross test.
The combined analysis of variance was performed with the linear model Yijkl = µ+αl δ+ k(l) +νij +υij +(αν)ijl +(αυ)ijl +eijkl, where Yijkl is the observed phenotypical value of the cross (i, j) within replication k in location l; µ is the general mean; αl is the effect of location l; δk(l) is the effect of the block or replication k in location l; νij is the effect of the cross=gi +gj +sij, where gi and gj are the effects of GCA of parents i and j, respectively; sij is the effect of the SCA of the cross (i, j); υ ij =mi -mj +rij, where mi and mj are the maternal effects of parents i and j, correspondingly, and rij is the reciprocal effect of the cross (i, j); (αν)ijl =(αg)il +(αg)jl +(αs)ijl where (αv)ijl is the interaction effect between crosses and the environment; (αg) il and (αg)jl are the interaction effects between the GCA effects of g i and gj with the environment, respectively; (αs)ijl is the interaction between the sij effect and the environment; (αυ)ijl =(αm) il +(αm)jl +(αr)ijl , where (αυ)ijl is the interaction effect between υij and the environment; (αm)il and (αm) jl are the interaction effects between mi and mj with the environment; (αr)ijl is the interaction effect between rij and the environment; and eijkl is the random effect of the error corresponding to the observation (i, j, k, l).
The diallel analysis was computed with SAS version 9.3 software (SAS, 2010). Tests of significance for principal effects (GCA, SCA, EMat, ERec) and interactions (GCA×Loc, SCA×Loc, EMat×Loc and ERec×Loc) were carried out with F tests proposed by Martínez (1983) for the Griffing Design I.
To determine the stability of the expression of each genotype, the analysis was performed with the mean value over locations. Nevertheless, given the significant interaction between locations and combining ability, the specific analysis by location was included.
Results and Discussion
Analysis of variance
Mean squares of the combined analysis of variance were significant (p≤0.01) for crosses and locations (Table 2) for grain yield (Rto), and the variables Pmz1, Pgr and Lmz showed high correlation with Rto. Differences among crosses are attributed to the expression of genetic variability associated with the types of gene effects expressed in each cross and to heterosis, due to interaction of the participating lines.
The contrasting expression among locations for different traits is attributable to environmental effects on genotypes, that is, the inability of some genotypes to overcome differential ecological effects associated with moisture, temperature, and phytopathological and soil conditions. This determined the significant interaction of crosses by locations (Cruzas×Loc). Hallauer et al. (2010) point out that single crosses, compared with trilinear and double crosses, given their homogeneity, generally have greater interaction with the environment.
Because differences among crosses were significant (p≤0.01), sum of squares was fractioned into GCA and EMat of the parents and SCA and ERec of the crosses (Table 2). GCA and SCA were significant (p≤0.01) for all variables, which means that contrasts were due to additive and non-additive effects.
Table 2 Mean squares of the combined analysis of variance for grain yield and three variables that correlated most with yield in a complete scheme of diallel crosses.

** p≤0.01 and * p≤0.05; FV: source of variation; GL: degrees of freedom; Rto: grain yield; Pmz1: weight of primary ears; Pgr: grain weight per ear; Lmz: ear length; ACG: general combining ability; ACE: specific combining ability; EMat: maternal effects; ERec: reciprocal effects; Loc: location; and CV: coefficient of variation.
The EMat were not significant (p>0.05), that is, the traits Rto, Pmz1, Pgr and Lmz expressed only nuclear inheritance and did not show significant effects due to extra nuclear genetic information. ERec had significant differences (p≤0.05) for Rto and Pmzl, which were attributable to interaction effects of nuclear and cytoplasmic DNA. Cockerham (1963) denominated these effects non-maternal.
The significant interaction Cruzas×Loc led to the decomposition of interaction effects: GCA×Loc, SCA×Loc, EMat×Loc and ERec×Loc (Table 2). GCA×Loc and SCA×Loc interactions were significant (p≤0.01), except in the variable Pgr and ACE×Loc; EMat×Loc and ERec×Loc were not significant. The significant interactions showed that GCA and SCA effects were specific for the evaluation environments. Therefore, analysis for each location was included.
The ANOVA sum of squares indicated that the contribution of grain yield variance attributable to the crosses, was explained by SCA (54 %), GCA (34 %), ERec (8.7 %) and EMat (2.3 %). That is, for Rto, non-additive effects were more important than additive effects, although the latter were also significant in the expression of genetic variation of yield. A similar trend was observed for Pgr. According to Ávila et al. (2009), superiority of non-additive effects may be due to heterosis resulting from hybrid combinations, which could be associated with genetic divergence among parental lines (Legesse et al., 2009). For Pmzl, GCA and SCA effects were similar, whereas for Lmz, GCA effects were higher than those of SCA; ERec effects contributed little (<6 %) to the variance among crosses. Similar studies have also shown that grain yield is controlled mainly by non-additive effects (Ávila et al., 2009; Pérez-López et al., 2014; Rodríguez-Pérez et al., 2016).
These results show that the effects of GCA, SCA and ERec had different degrees of relevance for each considered trait and genotype studied. Nevertheless, those lines whose GCA for grain yield correlates with GCA for yield components are desirable (Fan et al., 2008).
The non-significant maternal effect (EMat) would mean that crosses can be used in both directions (direct and reciprocal) with no significant differences. This is backed by Cockerham (1963) and Hallauer et al. (2012), who pointed out that in plants maternal effects are minimal and it is generally not necessary to consider them. However, there could have been erroneous interpretations and some uncertainty could have been generated in the results if they had not been evaluated (Mahgoub, 2011). In contrast, Fan et al. (2013) considered maternal effects important for crops such as maize because grain yield is widely determined by the endosperm.
Grain yield and GCA of parental lines
Phenotypical expression of parental lines was outstanding. Even though they are highly homozygous (S6-S8), their average grain yield was higher than the necessary minimum for seed production (5.6 t ha-1, general average; Table 3). In this regard, Espinosa et al. (2002) stated that, to be economically profitable, the lines used as female parents for seed production of single cross hybrids should yield at least 3.0 t ha-1.
Table 3 Grain yield and general combining ability of parent lines in three locations of the High Valleys of Central Mexico.

** p≤0.01 and * p≤0.05; Rto: grain yield; gi: effect of GCA; and DHS: Honest significant difference. The value in parentheses shows the order of the lines in terms of the effect of their GCA (1: highest and 10: lowest).
Based on the mean value of the three locations, yield of the lines showed an interval of variation between 8.3 to 3.8 t ha-1; particularly, yield potential was greater in Mixquiahuala, Hidalgo (6.3 t ha-1 on average) than in Tecámac, Estado de Mexico (4.8 t ha-1 on average).
This performance was associated with specific conditions of each location. In Mixquiahuala, although water availability was lower than usual, reduced from six to three applications (with wastewater) because of water shortages in the irrigation district, timely supply (at sowing and during flowering and grain filling) produced better expression of genotypes (lines and single crosses). In Montecillo, an atypical incidence of Fusarium spp. in the experimental plot caused a significant reduction (20 %) in yield. This event was unexpected because yield could have been similar to that of Mixquiahuala because of optimal crop management. In Tecámac, the irrigation system was also affected, and only two water applications (at sowing and flowering) caused lower grain yield, relative to the rest of the locations.
In Mixquiahuala, lines exhibited variation for Rto, from 9.7 (L6) to 3.9 (L2) t ha-1 (Table 3). In Montecillo the interval was 9.1 (L6) to 4.2 (L2) t ha-1, and in Tecámac 6.3 (L7) to 3.8 (L9) t ha-1. Despite the satisfactory yield of the lines, it was possible to discriminate superior lines based on yield expression. In accord with the mean value over locations, lines L5, L6 and L7 consistently yielded an Rto equal to or higher than 6.0 t ha-1, while L2, L3, L4 and L9 had yields below 5 t ha-1.
Given the significance of the interaction GCA by locations, expression at each site was analyzed. In Montecillo (Table 3), lines L6, L5, L1 and L7 had higher Rto (9.1, 7.0, 6.7 and 6.7 t ha-1, respectively), but only L6 and L5 had outstanding Rto and significant GCA. Line L8 with an Rto of 5.7 t ha-1 had the highest GCA (0.76). Therefore, lines L5, L6 and L8 were considered to have superior GCA.
In Mixquiahuala (Table 3), lines L6, L10, L5, L7 and L8 had higher Rto (9.7, 8.4, 8.0, 7.2 and 7.2 t ha-1). According to Rto and GCA effects, it was determined that, for this location, the lines with significant GCA were L5, L6, L8, L9 and L10.
In Tecámac (Table 3), lines with higher Rto were L7, L6, L5, L1 and L10, but only three of them (L5, L6 and L10) had positive GCA effects. Lines L8 and L9 would be added as lines with high GCA and medium yield.
The above, plus the mean for Rto and GCA, made it possible to differentiate and classify the parental lines based on performance into the following groups: 1) high GCA and superior Rto, L5 and L6; 2) high GCA and medium Rto, L8 and L10; 3) positive GCA and low Rto, L9; 4) low GCA and medium Rto, L1 and L7; and 5) low GCA and low Rto, L2, L3 and L4.
Classification of the lines based on their GCA and Rto was adequate because those with high GCA are expected to form crosses with higher yields due to the contribution of their favorable alleles (Reyes et al., 2004; Legesse et al., 2009; Escorcia-Gutiérrez et al., 2010; Pérez-López et al., 2014). On the other hand, lines with low GCA mated among themselves would not be expected to originate outstanding crosses.
Rto and GCA are not directly related since in Montecillo lines L1 and L7 had high Rto and negative gi , and L8 had medium Rto but positive gi . That is, of the lines with higher Rto, only some had higher GCA effects. These results partially contrast with those of Reyes et al. (2004), who found that inbred lines with high Rto were also those with high GCA. However, results of our research agree with those of Pérez-López et al. (2014), who also found this type of performance (high per se grain yield but not necessarily high GCA effects) and attributed it to selection of lines by multi-trait criteria: superior grain yield with other desirable characteristics such as earliness, plant height, ear weight, number of rows, which could lead to good inbreds but not necessarily with high GCA.
Grain yield and SCA of the crosses
Although SCA×Loc interaction was significant (Table 2), the analysis of yield expression of crosses and their SCA was based on the mean value of the three locations. This allowed recognizing the specific stability or adaptation of the crosses and the effect of SCA.
By location, the mean yield of the 90 possible crosses followed the same trend as yield of lines: Mixquiahuala (8.5 t ha-1) > Montecillo (6.8 t ha-1) and Tecámac (6.0 t ha-1). This performance was dependent on the environmental conditions of each respective location (Table 4).
Table 4 Effect of specific combining ability in 22 crosses with the highest grain yield and five with the lowest grain yield in each location of study and their mean values.

Rto: grain yield; sij: effect of SCA; DHS: honest significant difference; No: ascending order of the genotypes (1-90) based on the mean yield of the three localities studied. In bold type, the ten crosses with the highest yields in each location.
In general and based on the mean value, grain yield of the 22 crosses with outstanding yield, which included the ten highest yielding crosses of each location, was between 8.7 and 7.3 t ha-1. In contrast, the lowest yielding cross produced 4.9 t ha-1 (Table 4).
The SCA values were predominantly positive for the superior yielding crosses and negative for low-yielding crosses. This expression was similar to other studies on combining ability (Reyes et al., 2004; Pérez-López et al., 2014). The SCA variation interval was 1.21 to -1.31, which in the extreme positive case the value did not match the highest expression of Rto, but it did coincide in the extreme negative case with lower Rto. Nevertheless, despite the existence of variation for this effect, the values were smaller compared with those obtained by Reyes et al. (2004) and Escorcia-Gutiérrez et al., (2010), but similar to those obtained by Legesse et al. (2009). This may have been due to genetic divergence among the lines, which was also observed through comparisons of yield of the best lines and their respective crosses.
According to mean yield, the eight superior crosses (>8.0 t ha-1) were outstanding because they had superior Rto in at least two locations, but none of the ten superior crosses were superior at all the locations. No perfect direct relationship was observed between SCA and Rto; crosses L9×L6 and L9×L10, with high Rto showed high positive SCA values, but crosses L3×L9 and L5×L7 had higher SCA values. In contrast, cross L6×L10, with a medium Rto, had negative SCA.
Although SCA values could not entirely explain yield expression, there was a direct trend between GCA of parental lines and yield expression of their crosses. Of the 90 possible crosses, 22 were outstanding (Table 4), and of these, eight had superior Rto (>8.0 t ha-1) and were formed only by high GCA lines (L5, L6, L8 and L10) or at least one positive GCA line (L9). Of the rest of the outstanding crosses (<8.0 t ha-1), most of them had at least one low GCA line, but also there were some crosses which were made by two high GCA lines, such as L9×L8 and L5×L8. There were also crosses between two lines with higher Rto and high GCA, for example, L5×L6.
The genetic expression of grain yield, for the outstanding crosses, was attributable to two possibilities: 1) that the cross was formed by two high GCA lines, in which case the effects of SCA may or may not be high, and 2) that the cross is formed with at least one high GCA line, but whose SCA effect is high in at least one location. These results coincide partially with those of Reyes et al. (2004) and Pérez-López et al. (2014) and are part of the genetic variation for expression of maize traits in such a way that satisfactory yields are also possible with crosses of low GCA lines, as shown by Rodríguez-Pérez et al. (2016).
Regarding low yielding crosses, SCA effects were negative, and the four crosses with inferior yield performance were formed by low GCA lines: L1, L2, L3 and L7. There was only one cross (L3×L5) where one high GCA line participated.
Taking the 22 outstanding crosses for grain yield, GCA of the parental lines was identified and classified based on their participation in outstanding crosses. Line L10 participated in practically 50 % (10 crosses), followed by L9, L8 and L6, which, with the exception of the last (with high Rto), were classified previously as high GCA lines (L8 and L10) or positive GCA (L9), but medium or low per se yield. Moreover, considering the yield expression of cross L5×L6, whose lines were of high GCA and high Rto, it was determined that yield per se of a line was not indicative of its GCA nor does it guarantee that its crosses will express superior yield.
No significant maternal effects among lines was confirmed (Table 4); there were at least five pairs of crosses with similar yield. That is, both direct and reciprocal crosses, such as L9×L10 and L10×L9, L10×L8 and L8×L10, L1×L7 and L7×L1, showed equivalent expressions.
Genetic structure of the crosses
The genetic structure of a cross can reveal the types of genetic effects that operate in the cross. If additive effects are more important in a genotype than non-additive effects (dominance and epistasis), we recommend breeding through recurrent selection. On the other hand, if the non-additive effects are predominant, hybridization is the best option (Rodríguez-Pérez et al., 2016). Based on the above, the following analysis was carried out on ten crosses (of the 90 possible) that had the highest mean yield over locations, as well as on the cross with the lowest expression (Table 5). Maternal and reciprocal effects were not considered in this analysis because of their low contribution to yield variation.
Table 5 Genetic structure of ten crosses with the highest grain yield and the cross with the lowest grain yield, with the mean value of the evaluation in three locations of the High Valleys of Central Mexico.
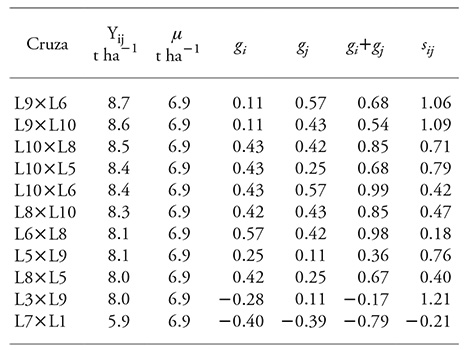
Y ij : observed phenotypical value of the cross (i, j); µ: general mean; g i and g j : effect of the general combining ability of parents i and j, respectively; s ij : effect of the cross SCA (i, j).
With exception of L3×L9, effects of GCA and SCA were positive for the crosses of superior yield, and for the cross with the lowest yield, all effects were negative.
The genetic structure of the crosses had contrasting composition, which highlights the possibility of using specific genotypes. Crosses L9×L6 and L9×L10, L5×L9 and L3×L9, where s ij >g i +g j , and additive effects were lower than non-additive effects, can be used only as hybrids because inbreeding depression in segregant generations would be expected; this is an undesirable characteristic in maize production.
Crosses L10×L8 and L10×L5, where g i +g j =s ij , can be used as hybrids or can be incorporated into a process of recurrent selection. Crosses L10×L6, L8×L10, L6×L8 and L8×L5, where g i +g j >s ij and additive effects were greater than dominance, can be used as open pollinated varieties with no detriment to yield of advanced generations.
The results of genetic structure coincided with the explanation of the sum of squares of the analysis of variance, which indicated that the contribution to grain yield variance was mainly determined by non-additive effects, which, however, were not negligible.
Conclusions
Predominance of non-additive effects (dominance and epistasis) over additive effects in the complete system of crosses allowed determining that using the parents in a system of hybridization is the most adequate scheme for a genetic breeding program.
The lines did not show significant maternal (extranuclear) effects, and therefore outstanding crosses can be programmed for seed production, using females or males indistinctly.
Lines and crosses with superior yields showed potential for use in the production of grain for the locations evaluated in the High Valleys of Central México
Literatura Citada
Ávila P., M., S.A. Rodríguez H., M.E. Vázquez B., F. Borrego E., A. J. Lozano R., y A. López B. 2009. Aptitud combinatoria y efectos recíprocos en líneas endogámicas de maíz de Valles Altos del Centro de México. Agric. Téc. Méx. 35: 285-293. [ Links ]
Cockerham, C. C. 1963. Estimation of genetics variances. In: W. D. Hanson, and H. F. Robinson (eds). Statistical Genetics and Plant Breeding a Symposium and Workshop Sponsored by The Committee on Plant Breeding and Genetics of the Agricultural Board at the North Carolina State College, Raleigh, N.C., March 20-29, 1961. National Academy of Sciences-National Research Council. No. 982. pp: 53-94. [ Links ]
Escorcia-Gutiérrez N., J. D. Molina-Galán, F. Castillo-González, y J. Mejía-Contreras. 2010. Rendimiento, heterosis y depresión endogámica de cruzas simples de maíz. Rev. Fitotec. Mex. 33: 271-279. [ Links ]
Espinosa C. A., M. Sierra M., y N. Gómez M. 2002. Producción y tecnología de semillas mejoradas de maíz por el INIFAP en el escenario sin la PRONASE. Agron. Mesoam. 14: 117-121. [ Links ]
Fan, X. M. et al. 2008. Combining abilities for yield and yield components in maize. Maydica 53: 39-46. [ Links ]
Fan, X. M. et al. 2013. Reciprocal diallel crosses impact combining ability, variance estimation and heterotic group classification. Crop Sci. 54: 89-97. [ Links ]
Gardner, C. O., and S. Eberhart A . 1966. Analysis and interpretation of variety cross diallel and related population. Biometrics 22: 439-452. [ Links ]
Griffing, B. 1956. Concept of general and specific combining ability in relation to diallel crossing systems. Aust. J. Biol. Sci. 9: 463-493. [ Links ]
Hallauer A. R., M. Carena and J. B. Miranda F. 2010. Quantitative Genetics in Maize Breeding. Springer-Verlag. New York. 664 p. [ Links ]
Mahgoub M. G. 2011. Partitioning of general and specific combining ability effects for estimating maternal and reciprocal effects. J. Agric. Sci. 3: 213-222. [ Links ]
Martínez G. A. 1983. Diseño y Análisis de Experimentos de Cruzas Dialélicas. Centro de Estadística y Cálculo. Colegio de Postgraduados. 252 p. [ Links ]
Legesse B. W., K. V. Pixley, and A. M. Botha. 2009. Combining ability and heterotic grouping of highland transition maize inbred lines. Maydica 54: 1- 9. [ Links ]
Pérez-López F. J. et al. 2014. Líneas homocigóticas de maíz de alto rendimiento como progenitoras de híbridos de cruza simple. Agrociencia 48: 425-437. [ Links ]
Pérez C. J. P. 2001. Metodología para la evaluación de cosecha de maíz en parcelas comerciales. Campo Experimental Pachuca, Hidalgo. INIFAP, CIR-CENTRO. Desplegable para productores No. 5. 5 p. [ Links ]
Reyes L. D., J. D. Molina G., M. Oropeza R., y E. Moreno P. 2004. Cruzas dialélicas entre líneas autofecundadas de maíz derivadas de la raza Tuxpeño. Rev. Fitotec. Mex . 27: 49-56. [ Links ]
Restrepo B., L. F., y J. González L. 2007. De Pearson a Spearman. Rev. Colomb. Cienc. Pecu. 20: 183-192. [ Links ]
Rodríguez-Pérez G., F. et al. 2016. Aptitud combinatoria y heterosis entre líneas de dos tipos de maíz para grano. Interciencia 41: 48-54. [ Links ]
SAS Institute. 2010. SAS/STAT User’s Guide: Version 9.2 SAS Institute, Inc. Cary, North Carolina, USA. 1689 p. [ Links ]
Sprague, G. F., and A. Tatum L. 1942. General vs. specific combining ability in single crosses of corn. J. Amer. Soc. Agron. 34: 923-32 [ Links ]
Received: July 2016; Accepted: December 2016