Introduction
Studies on the use of renewable energy sources advance because oil, a nonrenewable source of energy is running out and by concerns regarding the environmental factor due to global climatic changes. Glycerin is a byproduct of biodiesel, a renewable source of energy that is widely studied (Ma et al., 1998; Muñoz-Lagos et al., 2010).
Glycerin is the primary byproduct of biodiesel production and its chemical composition depends on the type of the raw material used (Thompson and He, 2006). In the biodiesel production process sodium hydroxide is used as a transesterification catalyst to increase the chemical reaction speed and improve industry yield (Ma et al., 1998). This procedure includes approximately 1 % of sodium in the raw glycerin (Lammers et al., 2007), which could affect the agricultural use of this residue.
Sodium is considered a beneficial element for plant nutrition, can replace the function of K in the osmotic control, and promotes plant growth (Marschner, 1995), especially in species classified as salinity tolerant such as beet (Beta vulgaris) (Aquino et al., 2006). However, the excess of Na can raise the salt content of the soil (Demiral, 2005), affect soil microbial life (Chávez and González, 2009), decrease nutrients absorption (Amini et al., 2007) affecting cell metabolism that may cause osmotic imbalance, membrane disruption, inhibition of cell division and expansion, interruption of ionic balance and reduction of photosynthesis (Mahajan and Tuteja, 2005), increase plant transpiration (Chen and Jiang, 2010) and, therefore, restrict plant growth and crop yields (Cruz et al., 2003). One possibility to increase plant tolerance to glycerin containing Na would increase K fertilization, since there are reports that high K ion concentrations in plants increases the ratio K/Na, ensuring optimal cellular metabolism and increased plant growth even under saline stress (Bartels and Ramanjulu, 2005).
Data on the agricultural use of glycerin are scarce, what is of concern considering the high increase in the supply of this byproduct due to the growing biodiesel world production. Knowledge of its agronomic performance can guide its efficient use in crop production.
The hypotheses would be if the use of glycerin containing Na in its composition associated with K fertilization could minimize possible damage by salinity and allow the use of larger rates of the residue. However, the use of very high rates of glycerin can increase soil salinity, decreasing microbiological activity, affecting plants dry matter regardless of the concentration of K in the soil.
The objective of this work was to evaluate the effect of glycerin rates in the absence or presence of K fertilization on the microbial activity, nutrition and production of beet.
Material and methods
The study was conducted in a greenhouse at Universidade Estadual Paulista, Jaboticabal Campus, Brazil (21° 15’ 22” S, 48° 18’ 58” W and altitude of 575 m) with beet cv. Early Wonder, from September to November 2014.
The experimental design was randimized blocks and treatments were glycerin doses: 0, 50, 100, 200, and 400 m3 h -1 in the absence and presence of K (150 mg dm3), with four replicates. Crude glycerin diluted in water at 14 % and KCl as the source of K (K2O 60 %) were used. Each 5 dm3 pot was filled with 4 dm3 medium texture Red-Yellow Agrisol, classified according to EMBRAPA (1999). The initial soil chemical analysis was performed according Raij et al. (2001), with the following results: pH 5.1, 9 g dm3 organic matter, 0.8 K, 12 Ca, 8 Mg, 20 H Al, 20.8 SB (sum of bases: K Ca Mg), 40.8 mmolC dm3 CEC (cation exchange capacity: SB H Al) and 51 % V (base saturation: SB 100/CEC).
Liming was performed to raise the base saturation to 70 %, as suggested by Raij et al. (1997) using calcined lime (Total Relative Neutralizing Power 125 %). After liming, soil was kept in plastic bags for 30 d to keep moisture at 60 % of field capacity.
Basic fertilization was incorporated to the soil at planting and received rates for leveling N (200 mg dm3), P (400 mg dm3), Zn (2 mg dm3), and B (0.5 mg dm3) in the form of urea, superphosphate, zinc sulfate and boric acid, as indicated by Malavolta (1981) and K applied in conjunction with the basic fertilization before planting.
Seedlings were obtained from 200 cell trays with substrate and vermiculite and after 30 d, two seedlings were transferred to each pot.
Glycerin was applied 30 d after transplanting and rates were subdivided in two weekly applications, following the experimental design. The control treatment received water only. In all treatments, moisture was kept at 70 % of the soil retention capacity. Raw glycerin was diluted in water at 14 %, with 0.6 % of Na, 0.02 % K2O and pH6.0.
At 30 d after transplanting the immediate effect of treatments was evaluated by recording data from plant height, number of leafs, and dry weight of shoot and tuber in a plant per pot. At the same time a microbiological analysis of the soil layer at 0-10 cm depth was performed. It was determined the respiratory activity of the soil following methodology proposed by Rezende et al. (2004) and the C of the microbial biomass was determined according to Ferreira et al. (1999).
At the end of the crop cycle, 70 d after transplanting, plant height, number of leaves and tuber diameter were evaluated. The areal portion and tuber roots were washed with a detergent solution and an acid solution, both with distilled water and dried in a forced air oven at 60-70 °C until dry mass was attained. At this time the dry mass of the aerial portion and tubers were quantified. Afterwards the material was milled and chemical analyses conducted to determine the K content in these organs, as described by Bataglia et al. (1983). Soil chemical analyses were conducted to determine the K concentration following methodology described by Raij et al. (2001) and to measure the electrical conductivity.
Data was subjected to the analysis of variance and polynomial studies conducted using software Sisvar (Ferreira, 2011).
Results and discussion
Soil salinity increased with quadratic adjustment as a function of glycerin rates for treatments with and without K, at 30 and 70 d after applications (Figure 1 A, B). These results may be related to the levels of sodium (0.6 %) present in the composition of the glycerin used, leading to increased soil salinity. Lammers et al. (2007) also report the presence of Na in glycerin and the risk of soil salinity. The highest salinity contents were obtained in the treatments with K compared to those without this nutrient. This is explained by the fact that glycerin applications associated with K increased concentration of this element in soil (Figure 1C) because we used the source of KCl, which is a salt fertilizer.

Figure 1 Soil salinity index at 30 (A) and 70 d (B) after glycerin application, soil K content (C), soil microbial respiratory activity (D) and soil C microbial biomass (E) as a function of glycerin applications in the absence and presence of K in beet crop (Beta vulgaris).
Glycerin applications promoted quadratic increases in the soil respiratory activity, reaching maximum rates of 53 and 120 m3 h-1 with and without K (Figure 1 D) and also in the C microbial biomass, reaching the maximum at rates of 80 and 193 m3 h-1, with and without K (Figure 1 e). Soils with glycerin and K fertilization rates above 53 m3 h-1 decreased the microbial activity, with reduction in the biomass C content, especially with glycerin rates above 80 m3 h-1, a fact that could be explained due to the higher soil salinity content previously discussed. This deleterious effect on the soil microbial life is reported by Chávez and González (2009).
Glycerin increased K accumulation in the shoots, reaching the maximum at rates of 14 and 300 m3 ha-1 with and without K (Figure 2 A) and reflected its accumulation in the tuber, reaching the maximum at rate of 59 m3 h-1 associated with the nutrient. However, for the treatments without K there were reductions in its accumulation in the tuber (Figure 2 B).

Figure 2 Potassium accumulation in the shoot (A) and tuber (B) in beet plants, 70 d after treatments application.
This reduction by beet plants due to the use of higher rates of glycerin probably occurred due to the increase of the salinity of this residue in the soil (Figure 1 A, B). The concentration of K in plants decreases with increased salinity (Cornic, 2004) due to the competition of Na and K ions for the carrier sites, given the chemical similarity of the cations (Rodríguez-Navarro, 2000; Rus et al., 2004; Chazen et al., 2005; Wang et al., 2007).
Glycerin rates affected growth variables regardless of the K presence or absence, except tuber diameter and dry matter (DM), 70 d after experiment implementation, when there was interaction (Table 1).
Table 1 Growth and yield of beet (Beta vulgaris) at 30 and 70 d after treatments implementation. Jaboticabal-SP, Brazil, 2014.
Treatments | PH | NL | TD | LDM | TDM | ||||
---|---|---|---|---|---|---|---|---|---|
K | 30 | 70 | 30 | 70 | 70 | 30 | 70 | 30 | 70 |
cm | cm | g pot -1 | |||||||
Without K | 28.7 | 32.85 | 8.90 | 12.50 | 48.9 | 2.47 | 5.78 | 1.04 | 8.82 |
With K | 28.3 | 35.05 | 8.32 | 14.05 | 52.4 | 2.32 | 6.90 | 1.07 | 10.27 |
Test F | 0.20 ns | 5.96** | 7.99** | 18.55** | 10.30** | 1.04ns | 9.56** | 0.05ns | 18.91** |
Glycerin(G) | |||||||||
0 | 33.2 | 35.87 | 9.69 | 13.25 | 53.37 | 2.77 | 6.42 | 1.42 | 10.67 |
50 | 34.1 | 38.25 | 9.88 | 14.75 | 61.62 | 3.72 | 8.06 | 1.83 | 13.75 |
100 | 32.0 | 36.00 | 8.87 | 13.62 | 56.12 | 2.89 | 7.18 | 1.28 | 10.93 |
200 | 29.1 | 32.87 | 8.44 | 13.25 | 51.75 | 2.04 | 5.96 | 0.73 | 9.75 |
400 | 14.2 | 26.75 | 6.19 | 11.50 | 30.37 | 0.57 | 4.10 | 0.0 | 2.62 |
Test F | 75.33** | 19.54** | 42.21** | 8.41** | 95.88** | 51.28** | 13.29** | 24.47** | 124.2** |
KG | 2.33 ns | 1.13 ns | 2.21 ns | 1.62 ns | 4.91** | 2.71 ns | 1.01 ns | 1.24 ns | 4.96** |
CV (%) | 9.4 | 8.4 | 7.5 | 8.6 | 6.8 | 19.6 | 18.2 | 38.4 | 11.0 |
**: significant, Tukey test (p≤0.01); ns: not significant. PH: plant height; NL: number of leaves; TD: tuber diameter; LDM: leaf dry matter; TDM: tuber dry matter
In the first evaluation, 30 d after glycerin application, there was a linear decrease in the number of leaves with and without K (Figure 3 B) and in the leaf DM (Figure 3 C) and tubers (Figure 3 D) when associated K. However, the use of glycerin at rates higher than 3.5 and 11 m3 h-1 reduced plant height with and without K (Figure 3 A).

Figure 3 Plant height (A), number of leaves (B), leaf dry matter (C) and tuber dry matter (D) in beet (Beta vulgaris) plants 30 d after treatment applications.
These effects reduced plant DM production; doses of glycerin greater than 70 and 43 m3 h-1, in presence of K, decreased leaves and tubers dry weight (Figure 3 C, D). These results indicate that beet seedlings are sensitive to glycerin, especially if associated with K because of salinity, as Chinnusamy et al. (2005) reported in plants in early growth stages. Glycerin increased growth variables at harvest with quadratic adjustment (Figure 4). The use of glycerin with and without K reached the maximum point at rates of 28 and 13, 60 and 80, 54 and 82, 45 and 25, 5 and 83, and 16 and 90 m3 ha-1 for plant height, number of leaves, leaf DM, tuberous root diameter, tuberous root DM and total DM (Figure 4).
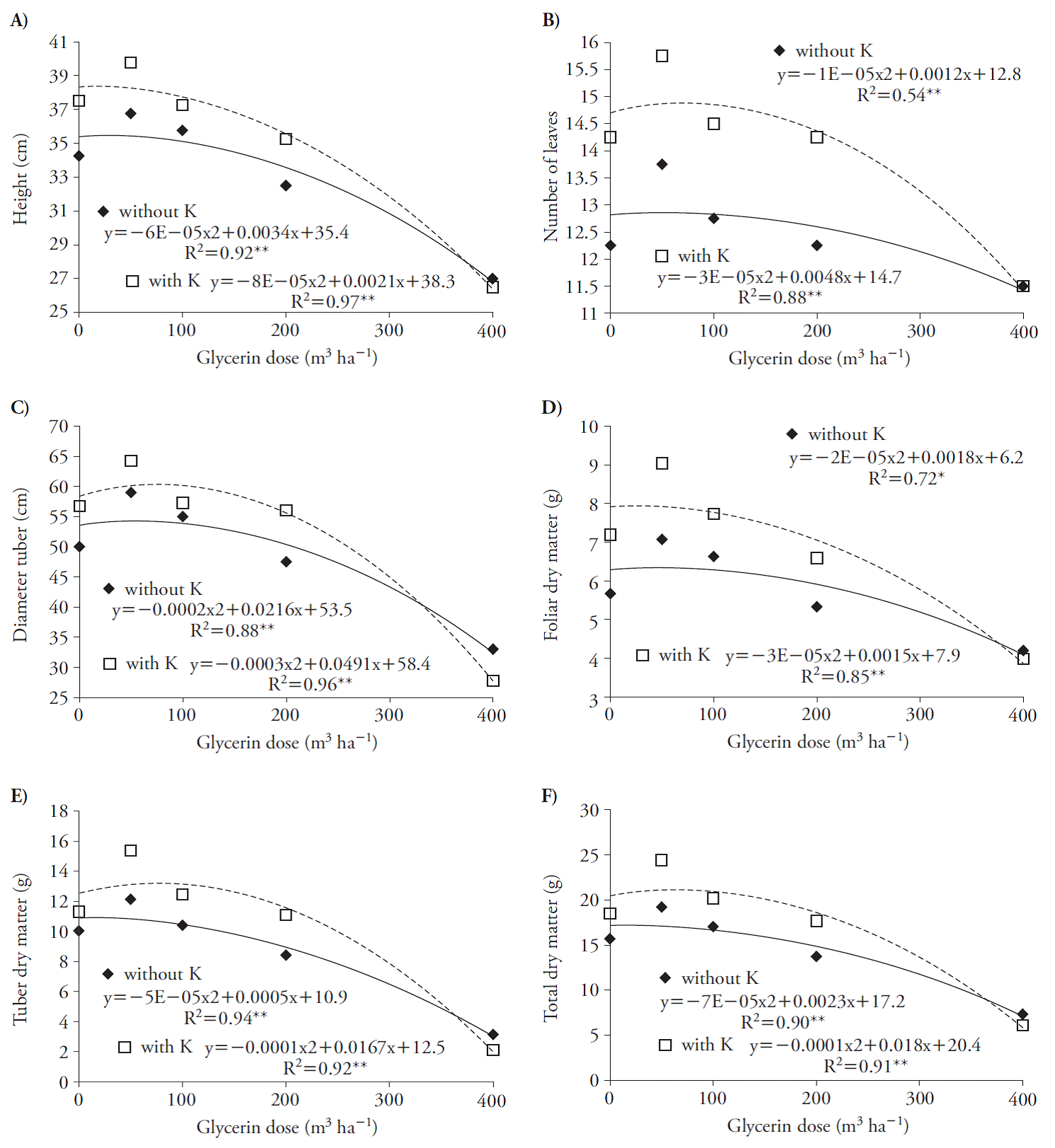
Figure 4 Plant height (A), number of leaves (B), diameter of the tuberous root (C), leaf dry matter (D), dry weight of the tuberous root (E) and total dry matter (F) of beet (Beta vulgaris) plants 70 d after treatments application.
The use of high rates of glycerin was harmful to the crop because it limited the total plant DM accumulation, especially in rates higher than 16 and 90 m3 ha-1 in the absence and presence of K (Figure 4 F). This fact is probably due to salinity, since it can affect the development of crops due to changes in the photosynthetic rate and in the carbohydrate metabolism (Argente et al., 2009), including beet, which is a plant species tolerant to soil salinity (Chávez and González, 2009). Besides, larger decreases in the DM of the whole plant due to glycerin (above 16 m3 ha-1) occurred without the K, probably due to increases in Na concentration in the plants which induced more severe reduction in K, affected the ionic balance and reduced the development of plants (Khoshgoftermanesh and Naeini, 2008).
There was higher tolerance of beet plants to glycerin when combined with K fertilization because there was high accumulation of this element in the plant, affecting the maintenance of ionic homeostasis, process of vital importance in the functioning of the plant cell. This was reported by Bartels and Ramanjulu (2005), who observed the need to maintain high K ion concentrations in plants subjected to salt stress, to maintain an appropriate K+/Na+ ratio, ensuring adequate cellular metabolism and major plant growth.