Services on Demand
Journal
Article
Indicators
-
Cited by SciELO
-
Access statistics
Related links
-
Similars in SciELO
Share
Agrociencia
On-line version ISSN 2521-9766Print version ISSN 1405-3195
Agrociencia vol.50 n.3 Texcoco Apr./May. 2016
Crop science
Productivity and physiological quality of irrigated rice seeds under salt stress and carbonized rice husk ashes fertilization
1Programa de Pós-Graduação em Ciência e Tecnologia de Sementes-UFPel/FAEM. Campus Universitário-Caixa Postal 354-CEP 96001-970.
The yield potential of rice in Brazil can be severely impaired by salinity, which affects about 2 % of the country's rice cultivation lands. Conditions of high salinity may be caused by inappropriate irrigation practices and soil flooding by sea water in coastal regions. Therefore, the objective of this study was to evaluate the effect of carbonized rice husk ashes applied to the soil on the yield and physiological seed quality of rice grown under salt stress. The experimental design was randomized blocks with five levels of factor A (0.0, 500, 1000, 1500 and 2000 kg ha -1 of rice husk ash) and three of factor В (0.0, 4.0, and 8.0 mM of NaCl) applied to the soil, with four replications. The response variables were the number of panicles per plant, number of seeds per plant, seed weight per plant, number of sterile glumes per plant and 1000 seed weight. The physiological quality of seeds was assessed through the germination test, first germination count, cold test, accelerated aging, seedling shoot and root lengths and field emergence. The salinity caused a negative effect on the agronomic traits and physiological quality of rice seeds. Weight and physiological quality of rice seeds were positively affected by increasing doses of carbonized rice husk ashes, even in the presence of salt concentrations in the soil.
Keywords: Oryza sativa L.; silicate salinity; germination; vigor
El potencial del rendimiento del arroz en Brasil puede ser muy perjudicado por la salinidad, que afecta cerca de 2 % de las tierras cultivables con arroz del país. Las condiciones de alta salinidad pueden ser causadas por prácticas de riego inadecuadas e inundaciones de suelos con agua marina en regiones costeras. Por lo tanto, el objetivo de este estudio fue evaluar el efecto de la ceniza de cáscara de arroz carbonizada aplicada al suelo sobre el rendimiento y la calidad fisiológica de la semilla sembrada bajo condiciones de estrés. El diseño experimental fue bloques al azar con cinco niveles de factor A (0.0, 500, 1000, 1500 y 2000 kg ha-1 de ceniza de cáscara de arroz) y tres de factor B (0.0, 4.0, y 8.0 mM de NaCl) aplicados al suelo, con cuatro réplicas. Las variables de respuesta fueron el número de panículas por planta, el número de semillas por planta, el peso de semillas por planta, número de glumas estériles por planta y el peso de 1000 semillas. La calidad fisiológica de las semillas se evaluó con la prueba de germinación, conteo de primera germinación, prueba de frío, envejecimiento acelerado, tallo de plántula y lengitud de raíces y emergencia de campo. La salinidad causó un efecto negativo sobre características agronómicos y calidad fisiológica de las semillas de arroz. En el peso y la calidad fisiológica de las semillas de arroz hubo un efecto positivo por dosis en aumento de ceniza de cáscara de arroz, incluso en presencia de concentraciones de sal en el suelo.
Palabras clave: Oryza sativa L.; salinidad de silicato; germinación; vigor
Introduction
Rice is the staple food of over half the world's population, with significant influence on socio-economic development. Brazil is among the top ten world producers, with about 12 million Mg of rice in 2012/2013, which was 2.2 % more than the previous cropping season. The states of Rio Grande do Sul and Santa Catarina account for almost 75 % of Brazil's rice harvest (Conab, 2013).
About 2 % of Brazil's rice area is affected by soil salinity (Menezes-Benavente et al, 2004), compromising yield potential. For irrigated rice one of the main factors determining the quality of irrigation is water salinity, which is due to excessive concentrations of soluble salts (Gomes et al. 2004) that disrupt plant growth. Conditions of high salinity may be caused by inappropriate irrigation practices and soil flooding by sea water in coastal regions (Tester and Davenport, 2003)· In Rio Grande do Sul, the standard for rice irrigation is to flood the rice fields, which could lead to soil salinization, especially in cropping areas that use water from coastal rivers like the Tramandai and Mampituba rivers, and Lagoons Patos, Mirim and Mangueira, which are subjected to salinization by seawater input when the level of these water springs decreases.
The intensity of salt stress upon rice cultivation is determined by the soil salt composition, the intensity and duration of stress, soil and climatic conditions, species cultivar and the crop's phenological stage (Maas and Hoffman, 1977)· Regarding the latter, rice seeds are relatively tolerant during germination but very sensitive at the seedling stage. After establishment, plant tolerance increases progressively until panicle differentiation, decreasing again through the flowering stage (Yoshida, 1981).
Proper plant nutrition is a key factor for satisfactory crop production. Silicon (Si) is beneficial for various crops (Korndorfer et al, 2002); it is needed for plant development (Epstein, 1994), especially in grasses, contributing to their adaptation to biotic and abiotic stresses. Silicon is important for rice cultivation (Pereira et al, 2004) since it increases growth and yield rates, it positively interacts with nitrogenous, phosphate and potassium fertilizers and provides resistance to biotic (pests and diseases) and abiotic (drought, salinity, lodging) stresses, and enhances productivity in soils with high levels of Al, Fe and Mn (Savant et al., 1997). Besides, Si may be involved in plant metabolic or physiological activities under salt or water stress or both (Gunes et al, 2008).
In Brazil there are several commercial products containing Si, such as gray carbonized rice husk, with a concentration of 92 % Si (Foletto, 2005) and can be used as a very efficient source of Si due to its high concentration. Rio Grande do Sul is Brazil's largest rice producer (Conab, 2013), generating rice husks as a by-product of rice milling, thus making it easily available, especially in the southern part of the state. According to Chungsangunsit et al. (2004), the rice husk accounts for 20 % of the total rice weight. After the complete combustion of rice hulls for power generation, approximately 20 %, is converted to gray (Pouey, 2006).
Therefore, the aim of this study was to evaluate the effects of rice husk ashes applied onto the soil on the yield and physiological quality of rice seeds produced under salt stress.
Materials and Methods
This experiment was conducted in a greenhouse and at the Seed Testing Laboratory (LAS) Flavio Farias Rocha, Plant Science Department, Agronomy Eliseu Maciel (FAEM) Faculty, Federal University of Pelotas (UFPel).
The experimental design was ranndomized blocks with a factorial arrangement of: 1) Rice husk ashes 0.0, 500, 1000, 1500, and 2000 kg ha -1; 2) NaCl 0.0, 4.0 and 8.0 mM; with four replications. Gray carbonized rice husks, milled to achieve a uniform particle size, was used as Si source, and rice cultivar IRGA 424 was the testing material.
Prior to sowing, seeds were treated with MAXIM XL®* (Fludioxonil + Metalaxyl-M) fungicide, CRUISER 350 FS® (Thiamethoxam) insecticide and Color Seed® polymer, at concentrations of 150, 150 and 300 mL 100 kg seed-1 , respectively, with a spray volume of 1200 mL 100 kg seed-1, which was completed with water. Seed coating was performed manually, according to the methodology described by Nunes (2005).
The coated seeds were thereafter sown into pots filled with soil collected from the Al horizon of a Eutrophic Haplic Planosol Solodicin (Streck et al., 2008), belonging to the mapping unit Pelotas; soil was sieved to achieve particle uniformity. The fertilization was adjusted according to the results of soil analysis and recommendations by the Committee on Soil chemistry and Fertility - RS / SC (2004). Liming was performed 30 d prior to sowing and rice husk ashes were applied at time of sowing, incorporated into the soil at a depth of 0.1 m. Treatments were 0.0, 500, 1000, 1500 and 2000 kg ha-1 SiO2, adjusting the concentrations to the soil volume of the experimental units (pots). Since gray carbonized rice husk contains approximately 92 % Si (range between 88-96 %), the equivalent to 0.0, 1.90, 3.80, 5.71, 7.61 g pot of gray carbonized rice husk was used, respectively, for increasing levels of Si.
Each experimental unit was sown with 10 seeds, thinning down to two seedlings per pot after emergence. Pots were irrigated daily in the morning, with the respective concentrations of NaCl according to treatment. The soil in each pot was maintained at field capacity until the establishment of the water shield, 30 d after emergence. The experiment was thus conducted until reaching the field maturation phase.
The inflorescences and seeds were assessed for agronomic traits and physiological quality, for which individual panicles were manually threshed, recording: 1) Number of panicles per plant (NPANPL): count of all panicles from each plant; 2) number of seeds per plant (NSPL): manual counting of seeds per plant; 3) seed weight per plant (SWPL): estimated through the weight of all seeds from individual plants, corrected to 13 % moisture content and expressed in g plant-1; 4) number of sterile glumes per plant (NSGPL): quantified through manual counting of all seeds from each experimental unit and expressed as number of vain seeds plant-1; 5) 1000 seed weight (1000W): estimated through eight 100-seed samples weighed in analytical scale; data for the above are presented as mean, standard deviation and coefficient of variance; 6)1000 seed weight estimated by multiplying the mean value by the factor of 10 (Brasil, 2009).
The assessment of seed physiological quality involved:
1) Germination (G) test: performed according to the Brazilian Rules for Seed Testing-RAS (Brasil, 2009), sowing 200 seeds in each experimental unit, divided in four replicates of 50 seeds each. The seeds were placed on wet germitest paper roll, adding 2.5 times the weight of the dry paper roll of distilled water. The germitest paper rolls were placed in a germination chamber at 25 °С, the seedling count was performed 14 d after sowing, and results expressed as percentage of normal seedlings.
2) First germination count (FGC): performed 7 d after the start of the test; it involved counting the normal seedlings within each replication. The results were expressed as percentage of normal seedlings;
3) Cold test (CT): four replicates of 50 seeds for each experimental unit were uniformly distributed on germitest paper rolls, moistened with distilled water at a ratio of 2.5 times the weight of dry paper. Immediately after the germitest rolls were placed in plastic bags, which were sealed and kept in a BOD chamber set at 10 °С ± 1 °С for 7 d. Afterward the rolls were transferred to a germination chamber and maintained under the same conditions of the germination test, assessing the percentage of normal seedlings after 7 d.
4) Accelerated, aging (AA): seeds were uniformly spread over a metal screen suspended in the germination box, containing 40 mL of distilled water. The boxes were capped and placed in growth chamber at 42 °С for 96 h. Afterward, the seeds were germinated according to the methodology described for the germination test and evaluated on the seventh day. The results were expressed as percentage of normal seedlings.
5) Seedling shoot length (SL) and root length (RL): seedlnigs from four replicates of 20 seeds for each experimental unit were collected after 7 d in the germination chamber at 25 °С, and the root and shoot lengths of ten randomly selected seedlings were measured using a ruler (mm).
6) Field emergence (FE): 200 seeds per treatment were sown in small plots and seedling emergence recorded on a single count at 21 d after sowing with results expressed as percentage of normal seedlings.
The experimental data were subjected to ANOVA by the Winstat Statistical Analysis System version 1.0 and treatment means were compared by the Tukey test (p≤0.05). The effect of Si concentrations on the response variables was analyzed through polynomial regressions (p≤0.05). Data on percentage basis were transformed [arc.sen (√x/100)] prior to analysis of variance.
Results and Discussion
Only main factor effects were detected through ANOVA for number of panicles per plant (NPANPL), number of sterile glumes per plant (NSGPL), germination test (G), cold test (CT), acelerating aging (AA), root length (RL) and field emergence (FE) (Table 1). Interaction between Si levels and salt concentration was observed for the response variables seed weight per plant (SWPL), thousand seed weight (1000W), first germination count (FGC) and shoot length (SL).
Table 1 Analysis of variance, mean squares and their significance for number of panicles per plant (NPANPL), number of seeds per plant (NSPL), seed weight per plant (SWPL), number of sterile glumes per plant (NSGPL), thousand seed weight (1000W), first germination count (FGC), germination test (G), cold test (CT), accelerated aging (AA), shoot length (SL), root length (RL) and field emergence (FE) originating from seeds of rice cultivar IRGA 424, due to the levels of carbonized rice husk ashes and NaCl concentrations applied to the soil. Pelotas, Brazil, 2013.

SV: source of Variation; DF: degrees of freedom. *F-test, p≤0.05; ոs: not significant
The number of panicles per plant (NPANPL) for the control NaCl concentration treatment (0 mM) was higher than for the other treatments (Figure 1). Increased NaCl concentrations contributed to the formation of sterile glumes. The mean number of sterile glumes per plant (NSGPL) was 21 units higher for the 8 mM concentration in relation to the control treatment; however, no differences on this trait were observed between the 4 mM and 8 mM concentration treatments, respectively. Productivity losses due to salinity can occur due to several causes, such as an induced reduction of the photosynthetic capacity (Sultana et al, 1999) and lower assimilate accumulation in kernels (Asch et al, 2000), which will decrease the filling rate due to insufficient carbohydrate supply to the panicles (Khatun and Flowers, 1995).

Figure 1 Mean numbers for panicles per plant (NPANPL) and sterile glumes per plant (NSGPL) in rice cultivar IRGA 424, the application of different carbonized rice husk ashes levels and NaCl concentrations to the soil. Pelotas, RS, Brazil, 2013. Means with different letter in each factor are statistically different (Tukey; p ≤0.05).
For NSPL, SWPL and 1000W, saline concentration o mM yielded higher values than 4 and 8 mM, at all doses of SiO2 concentration (Table 2). According to Rhoades et al. (2000), salinity affects the development and yield of plants, which is manifested through reductions on plant stand and seed development, with symptoms similar to drought stress. Soil salinity may be due to irrigation with saline water or by a combination of factors, such as water, soil and crop management, which may increase days to harvest, and reductions on the number of seed filling sites and seed weight, impacting directly on seed mean yields.
Table 2 Mean number of seeds per plant (NSPL), seed weight per plant (SWPL), thousand seed weight (1000W), first germination count (FGC), shoot length (SL) and mean root length (RL) of seedlings grown from seeds of rice cultivar IRGA 424, due to the levels of carbonized rice husk ashes and NaCl concentrations applied to the soil. Pelotas, Brazil, 2013.

Means with different letter within each factor are statistically different (Tukey, p≤0.05)
Thousand seed weight varies according to the genotype and may be related to seed vigor, it is influenced by environmental conditions and management, such as plant nutrition (Carvalho and Nakagawa, 2000). This was observed in our study since salinity stress interfered on the thousand seed weight; besides, seeds weight is reduced as a function of salinity for castor (Silva et al, 2008) and peanut (Correia et al, 2009).
Interaction between both main factors was observed for first germination count and shoot length (Table 2). Thus, 0 mM concentration yielded higher values than all the other treatments, but there were no differences for 500 kg ha-1 SiO2 concentration compared to 4 mM treatment as well as for 2000 kg ha-1 Si concentration between treatments 4 and 8 mM. Shoot length measurements were highest at the 0 mM treatment, whereas for 2000 kg SiO2 ha-1 there were no differences for shoot length between treatments o mM and 4 mM. No interactions were established between carbonized rice husk ashes levels and NaCl concentration on the root length. Root lengths were highest for the control treatment (0 mM), regardless of the level of applied carbonized rice husk ashes.
The mean number of panicles per plant (NPANPL) fitted a quadratic model (Figure 2A) with increasing values up to a dose equivalent to 500 kg ha-1 SiO2. The NaCl concentrations between 0-4 mM fitted a quadratic model also for the mean number of seeds per plant (NSPL) (Figure 2B). At the 0 mM salt concentration a 4.28 % increase (72 seeds per plant) was observed when increasing SiO2 levels up to 600 kg ha-1. Despite a low coefficient of determination (R2), maximum efficiency was achieved at a level of 722 kg ha-1 carbonized rice husk ashes, that is an increase of 4.39 % (47 seeds per plant) as compared to the control treatment. The mean number of seeds per plant did not change for the 8 mM, implying no response to the increase on SiO2 concentrations. Singh et al. (2005) observed that Si fertilization increased the number of panicles per unit area as well as productivity, when Si was applied in two consecutive rice harvests.
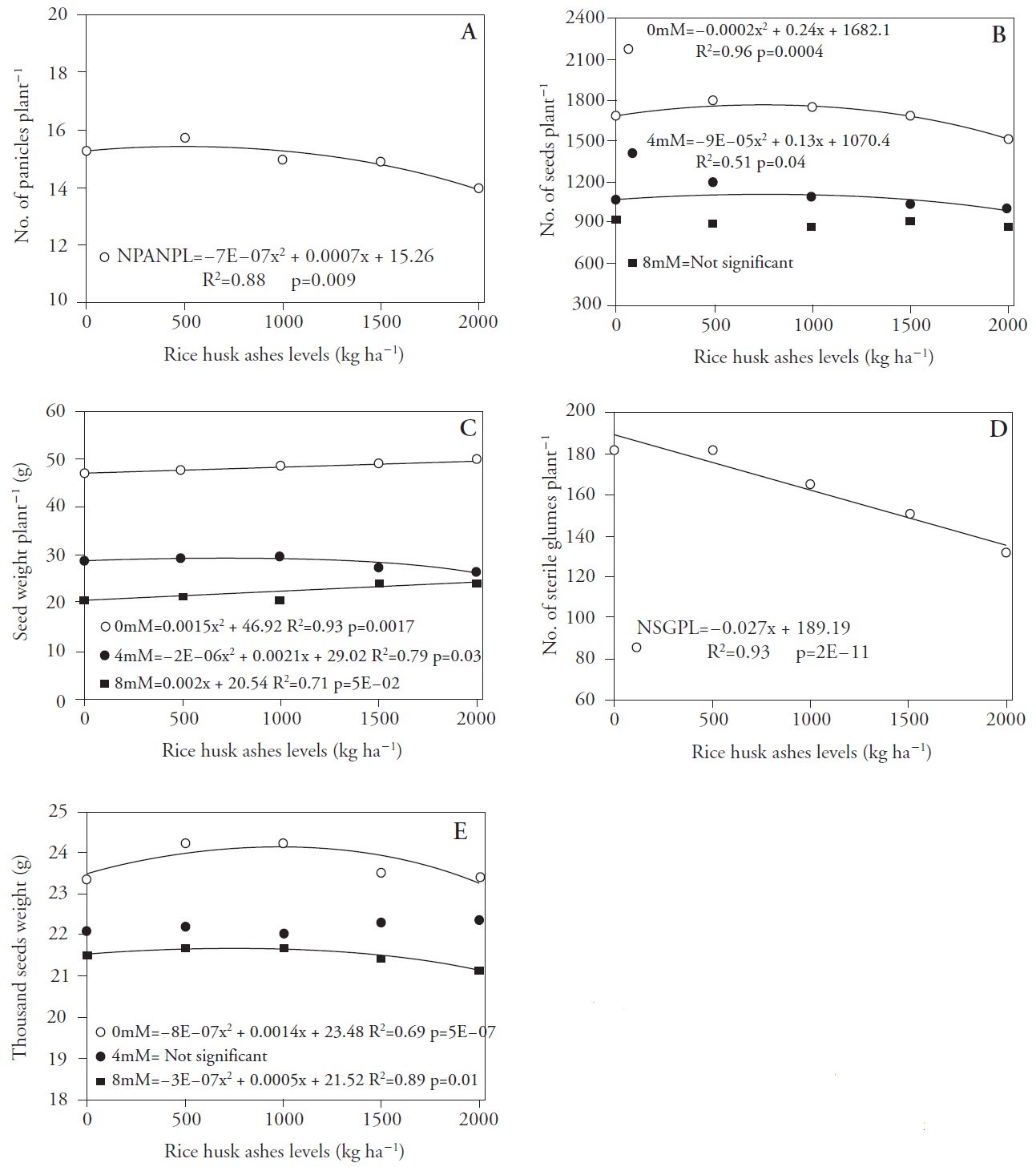
Figure 2 Mean number of panicles per plant (A), mean number of seeds per plant (B), seed weight per plant in grams (C), number of sterile glumes per plant (D) and thousand seed weight in grams (E) of seeds of rice cultivar IRGA 424, due to the different levels of carbonized rice husk ashes and NaCl concentrations applied to the soil. Pelotas, Brazil, 2013.
The seed weight per plant (Figure 2C) fitted a positive linear model for the NaCl concentration range 0 to 8 mM, where the highest SiO2 dose applied to the soil (2000 kg ha-1) caused an increase of 3 to 4 g plant-1, respectively. For the 0 to 4 mM concentration, the data fitted a quadratic model with maximum efficiency at 525 kg SiO2 ha-1 (29.57 g plant-1). Tahir et al. (2006) found that the application of calcium silicate significantly increased the seed yield of two wheat genotypes, both under normal and salty conditions, stressing its importance on the mineral nutrition of wheat.
The mean number of sterile glumes per plant (Figure 2D) decreased 0.027 units for each unit increase in the dose of carbonized rice husk ashes, whereas at the highest dose (2000 kg ha-1) this decrease was 28.54 % (54 glumes by sterile plant) relative to the zero dose. According to Pershin et al. (1995), Si fertilization enhances grain yield and thousand seed weight, and reduces spikelet sterility.
NaCl concentration from 0 to 8 mM had no effect on the thousand seed weight (Figure 2E), but there were no differences for the 4 mM concentration across the SiO2 doses. For o mM an increase in the thousand seed weight was observed, up to a 875 kg ha-1 SiO2 dose. For the 8 mM salt concentration, the maximum seed weight was achieved for 833 kg ha-1 SiO2. Oliveira et al. (2015) reported the benefits of bulk Si application on soybean seeds. However, calcium silicate, applied as powder or granulated, did not change the thousand seed weight and seed yield (Juliatti et al, 2004). The thousand seed weight is an important quality indicator that may increase productivity, since heavier seeds result in higher yields.
The results for physiological seed quality germination, cold test, accelerated aging and field emergency, showed no interaction between the NaCl concentration and SiO2 dose; therefore, only treatment means for salt concentrations were compared (Figure 3), and the effect of SiO2 doses was studied with a polynomial regression (Figure 4A, 4B and 4C). The values for these variables at the NaCl concentration o mM were higher than for the other NaCl concentrations. According to Vieira et al. (1994) and Marcos Filho (2005), seed physiological quality is more influenced by the prevailing environmental conditions during the ripening and harvesting stages, than by the type of cultivar itself. Lima et al. (2005) found a decrease in the germination percentage of rice seeds due to the increase in salt concentration, suggesting that salinity affects the development of normal seedlings and decreases the vigor and viability of seeds. Likewise, Deuner et al. (2011), in seed from different cowpea genotypes, found that the increase of soil salt concentration caused a negative effect on the first germination count and on the final germination value.

Figure 3 Percentage of normal seedlings form germination test (G), cold test (CT), accelerated aging (AA) and field emergence (FE) originating from seeds of rice cultivar IRGA 424, due to the different levels of carbonized rice husk ashes and NaCl concentrations applied to the soil. Pelotas, Brazil, 2013. Means with different letter within each factor are statistically different (Tukey, p≤0.05).

Figure 4 Germination (A), cold test and accelerated aging (B) and field emergence (C) of seedlings grown from seeds of rice cultivar IRGA 424, resulting from the application of different levels of carbonized rice husk ashes and NaCl concentrations applied to the soil. Pelotas, Brazil, 2013.
Final germination values (Figure 4A) were not affected by SiO2 levels applied to the soil, which is similar to that found in common oat seeds (Toledo et al, 2011) and seeds of Brachiaria (Santos et al, 2010) with increasing Si doses. However, the application of Si increased germination of wheat seeds under salt stress (Matichenkov et al., 2005)· Figure 4B shows that the accelerated aging and cold tests responded in a similar fashion to increased doses of SiO2, both exhibiting a positive quadratic behavior. The accelerated aging test yielded higher germination (92 %) up to 1375 kg SiO2 ha-1. The highest percentage of normal seedlings for the cold test was recorded for 1067 kg SiO2 ha-1, with 85 % germination. According to Ran et al. (1997), Si enhances biological capacity of seeds and seedlings to tolerate harsh environmental conditions. As for the field emergence test, Figure 4C shows that 2000 kg SiO2 ha-1 yielded an increase of 2.6 % as compared to the control treatment.
Increasing the NaCl concentration from 0 nМ to 4 mM for carbonized rice husk ashes concentrations of up to 950 to 900 kg ha-1, respectively, increased the percentage of normal seedlings for the first germination count (Figure 5), whereas higher concentrations reduced the count values. The highest germination count at salt concentration of 8 mM was at a carbonized rice husk ashes dose of 1150 kg ha-1, whereas higher doses reduced normal seedling counts but in all cases above those for the control treatment. The comparison of the three salt concentrations seem to show that the highest NaCl concentration requires higher carbonized rice husk ashes doses to achieve the point of maximum efficiency.
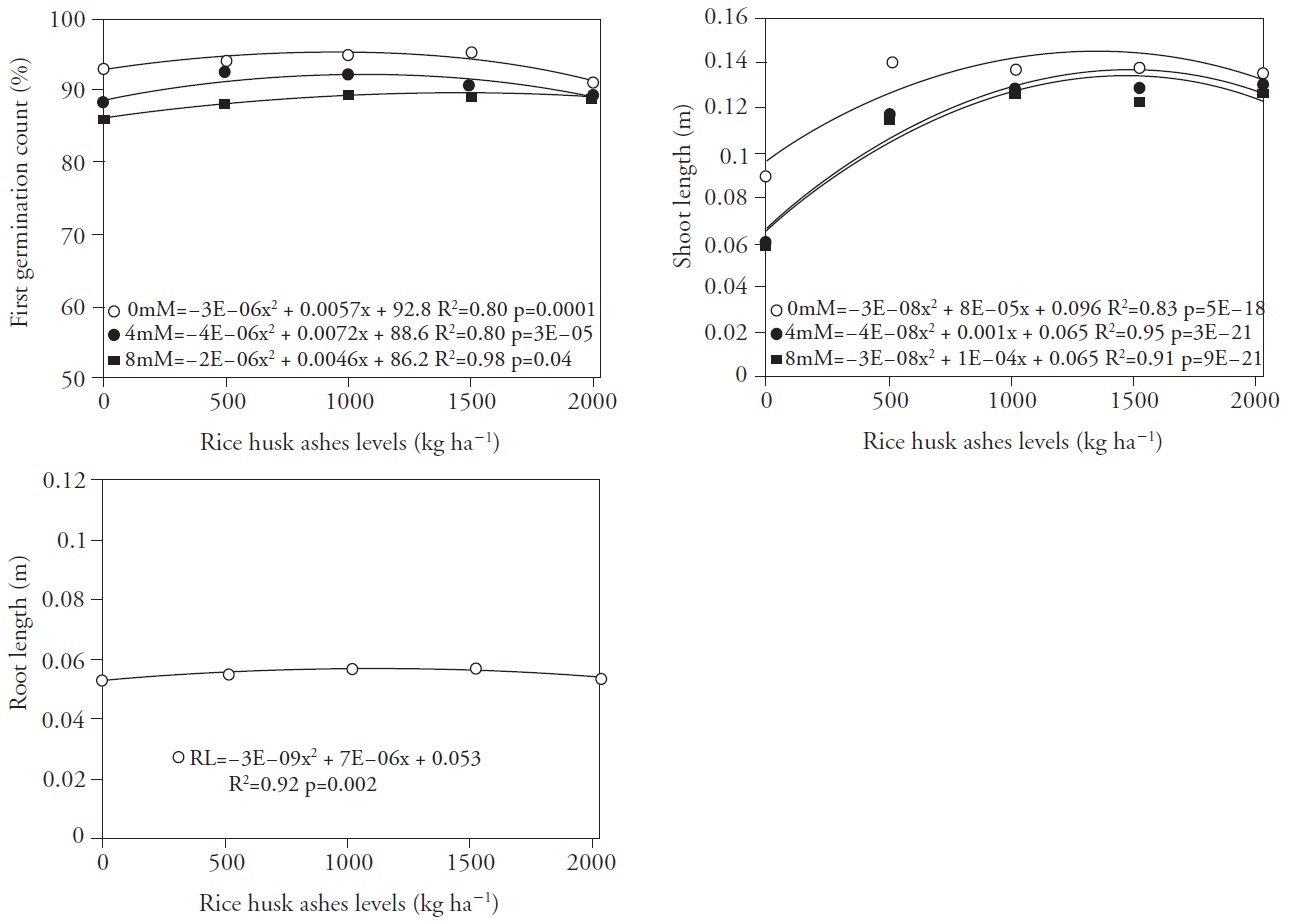
Figure 5 First germination count (a), shoot length (B) and root length (C) of seedlings grown from seeds of rice cultivar irga 424, resulting from the application of different levels of carbonized rice husk ashes and NaCl concentrations applied to the soil. Pelotas, Brazil, 2013.
Increase on the application of carbonized rice husk ashes yielded larger shoot lengths (Figure 5B). For the NaCl concentration of 0 mM the point of maximum efficiency was obtained with 1333 kg ha-1 carbonized rice husk ashes, resulting in an increase of 0.053 m on seedling length. For the NaCl concentration of 4 mM increases on shoot length were recorded up to the 1250 kg ha-1 carbonized rice husk ashes dose, representing an increase of 0.063 m. The increase on shoot length for NaCl concentration of 8 mM was 0.083 m at a dose of 1667 kg ha-1 carbonized rice husk ashes. Seedlings with higher early growth rate have the potential to originate higher yielding plants, since a larger leaf area will provide for a higher photosynthetic rate. According to Gustafson et al. (2004), plants exhibiting high speed of emergence and early growth have priority on the use of ambient resources and, therefore, have a competitive advantage on their use.
Root length showed a slight increase to increasing NaCl concentrations (Figure 5C) up to 1167 kg ha-1 carbonized rice husk ashes, with an overall mean of 0.057 m.
Carbonized rice husk ashes can increase plant salt tolerance, enhancing their capacity for water absorption (Romero et al, 2006). Other benefits include enhancement of leaf blade structure and photosynthetic potential (Shu and Liu, 2001), enhancement of the antioxidant system (Zhu et al, 2004) and increasing the concentration of soluble substances in the xylem, which will reduce plant sodium absorption (Liang et al, 1996; Matichenkov et al, 2001).
Literature Cited
Asch, F., M. Dingkuhn, K. Dörffling, and K. Miezan. 2000. Leaf K/Na ratio predicts salinity induced yield loss in irrigated rice. Euphytica 113: 109-118. [ Links ]
Brasil. 2009. Ministério da Agricultura, Pecuária e Abastecimento. Regras para análise de sementes. Ministério da Agricultura, Pecuária e Abastecimento. Secretaria de Defesa Agropecuária. Brasília, DF: Mapa/ACS. 395 p. [ Links ]
Carvalho, N. M., and J. Nakagawa. 2000. Sementes: ciência, tecnologia e produção. 4ed. Jaboticabal, Funep 588 p. [ Links ]
Chungsangunsit, T., S. G. Gheewala, and S. Patumsawad. 2004. Environmental Profile of Power Generation from Rice Husk in Thailand. Disponível em: <http://www.thaiscience.info/Article%20for%20ThaiScience/Article/3/Ts-3%20environmental%20profile%20of%20power%20generation%20from%20rice%20husk%20in%20thailand >. (Acess: April 2013). [ Links ]
Comissão de Química e Fertilidade do Solo - CQFS RS/SC. 2004. Manual de adubação e de calagem para os Estados do Rio Grande do Sul e de Santa Catarina. 10. ed. Porto Alegre, Sociedade Brasileira de Ciência do Solo. 400 p. [ Links ]
Conab Companhia Nacional de Abastecimento. 2013. Acompanhamento da Safra Brasileira de Grãos 2012/2013 Décimo Levantamento Junho/2013 Brasília: Conab. [ Links ]
Correia, K. G., P. D. Fernandes, H. R. Gheyi, R. G. Nobre, and T. S. Santos. 2009. Crescimento, produção e características de fluorescência da clorofila a em amendoim sob condições de salinidade. Rev. Ciê. Agr. 40: 514-521. [ Links ]
Deuner, C., S. M. Maia, S. Deuner, A. S. Almeida, and G. E. Meneghello. 2011. Viabilidade e atividade antioxidante de sementes de genótipos de feijão-miúdo submetidos ao estresse salino. Rev. Bras. Sem. 33: 711-720. [ Links ]
Epstein, E. 1994. The anomaly of silicion in plant biology. Proc. Nat. Acad. Sci. 91: 11-17. [ Links ]
Foletto, E. L., R. Hoffmann, R. S. Hoffmann, U. L. Portugal Jr., and S. L. Jahn. 2005. Aplicabilidade das cinzas da casca de arroz. Quí. Nova 28: 1055-1060. [ Links ]
Gomes, A. S., E. A. Pauletto, and A. F. H. Franz. 2004. Uso e manejo da água em arroz irrigado. In: Gomes, A. S. , and A. M. Magalhães Júnior (eds). Arroz Irrigado no Sul do Brasil. Brasília, Embrapa Informação Tecnológica; Embrapa Clima Temperado. pp: 417-455. [ Links ]
Gunes, A., D. J. Pilbeam, A. Inal, and S. Coban. 2008. Influence of silicon on sunflower cultivars under drought stress, in growth, antioxidant mechanisms, and lipid peroxidation. Com. Soil Sci. Plant Anal. 39: 1885-1903. [ Links ]
Gustafson, D. J., D. J. Gibson, and D. L. Nickrent. 2004. Competitive relationships of Andropogon gerardii (Big Bluestem) from remnant and restored native populations and select cultivated varieties. Fun. Eco. 18: 451-457. [ Links ]
Juliatti, F. C., M. G. Pedrosa, R. M. Lanna, S. H. Brito, and B. Melo. 2004. Influência do silício na redução de podridão de sementes por Fusarium semitectum na cultura da soja. Bio. J. 20: 57-63. [ Links ]
Khatun, S., and T. J. Flowers. 1995. The estimation of pollen viability in rice. J. Exp. Bot. 46: 151-154. [ Links ]
Korndörfer, G. H., S. H. Pereira, and M. S. Camargo. 2002. Silicatos de Cálcio e Magnésio na Agricultura. 2.ed. Uberlândia, GPSi/ICIAG/UFU. 24 p. [ Links ]
Liang, Y. C., Q. R. Shen, and T. S. Ma. 1996. Effects of silicon on salinity tolerance of two barley cultivars. J. Plant Nut. 19: 173-183. [ Links ]
Lima, M. G. S., N. F. Lopes, D. M. Moraes, and C. M. Abreu. 2005. Qualidade fisiológica de sementes de arroz submetidas a estresse salino. Rev. Bra. Sem. 27: 54-61. [ Links ]
Maas, E. V., Hoffman, G. J. 1977. Crop salt tolerance current assessment. J. Irrig. Drainag. Divis. 103: 115-134. [ Links ]
Machado, M. O., and A. L. Terres. 1995. Tolerância de genótipos à salinidade do solo safra 1994/1995. Reunião da Cultura do Arroz Irrigado, 21, Porto Alegre, RS. Anais. Porto Alegre: IRGA. pp: 48-50. [ Links ]
Marcos Filho, J. 2005. Fisiologia de Sementes de Plantas Cultivadas. Piracicaba: FEAlQ. 495 p. [ Links ]
Matichenkov, V. V., E. A. Bocharnikova, and J. M. Ammosova. 2001. The influence of silicone fertilizers on the plants and soils. Agrokhimiya 12: 30-37. [ Links ]
Matichenkov, V. V. ; A. A. Kosobrukhov, N. I. Shabnova, and E. A. Bocharnikova. 2005. Plant response to silicon fertilizers under salt stress. Agrokhimiya 10: 59-63. [ Links ]
Menezes-Benavente, L., F. K. Teixeira, C. L. A. Kamei, and M. Margispinheiro. 2004. Salt stress induces altered expression of genes encoding antioxidant enzymes in seedlings of a brazilian indica rice (Oryza sativa L.). Plant Sci. 166: 323-331. [ Links ]
Nunes, J. C. 2005. Tratamento de semente qualidade e fatores que podem afetar a sua performance em laboratório. Syngenta Proteção de Cultivos Ltda. 16 p. [ Links ]
Oliveira, S., Lemes, E. S., Meneghello, G. E., Tavares, L.C., Barros, A. C. S. A. 2015. Aplicação de silício via solo no rendimento e na qualidade fisiológica de sementes de soja. Semina: Ciên. Agr. 36: 3029-3042. [ Links ]
Pereira, H. S., G. H. Kondörfer, A. A. Vidal, and M. S. Camargo. 2004. Fontes de silício para a cultura do arroz. Sci. Agr., Piracicaba 16: 522-528. [ Links ]
Pershin, B. M., A. N. Pershima, and L. M. Egorina. 1995. Silicon and rice production in the Primorskii region. Agrikhimiya 10: 68-74. [ Links ]
Pouey, M. T. F. 2006. Beneficiamentos da cinza de casca de arroz residual com vistas à produção de cimento composto e/ou pozolânico. Tese (Doutorado em Engenharia Civil) Escola de Engenharia da Universidade Federal do Rio Grande do Sul, Porto Alegre. 320 p. [ Links ]
Rafi, M. M., E. Epstein, and R. H. Falk. 1997. Silicon deprivation causes physical abnormalities in wheat (Triticum aestivum L.). J. Plant Phy. 151: 497-501. [ Links ]
Rhoades, J. D., A. Kandiah, and A. M. Mashali. 2000. Uso de águas salinas para produção agrícola. Campina Grande: UFPB 117 p. [ Links ]
Romero-Aranda, M. R., O. Jurado, and J. Cuartero. 2006. Silicon alleviates the deleterious salt effects on tomato plant growth by improving plant water status. J. Plant Phy. 163: 847-855. [ Links ]
Santos, F. C., J. A. Oliveira, E. V. R. Von Pinho, R. M. Guimarães, and A. R. Vieira. 2010. Tratamento químico, revestimento e armazenamento de sementes de Brachiaria brizantha cv. Marandu. Rev. Bras. Sem. 32: 69-78. [ Links ]
Savant, N. K., G. H. Snyder, and L. E. Datnoff. 1997. Silicon management and sustainable rice production. Adv. Agr. 58: 151-199. [ Links ]
Shu, L. Z., and Y. H. Liu. 2001. Effects of silicon on growth of maize seedlings under salt stress. J. Agr-Env. Sci. 20: 38-40. [ Links ]
Silva, S. M. S., A. N. Alves, H. R. Gheyi, N. E. M. Beltrão, L. S. Severino, and F. A. L Soares. 2008. Desenvolvimento e produção de duas cultivares de mamoneira sob estresse salino. Rev. Bras. Eng. Agr. Amb. 12: 335-342. [ Links ]
Singh, A. K., R. Singh, and K. Singh. 2005. Growth, yield and economics of rice (Oryza sativa) as influenced by level and time of silicon application. Ind. J. Agr. 50: 190-193. [ Links ]
Streck, E. V., N. Kämpf, R. S. D. Dalmolin, E. Klamt, P. C. Nascimento, P. Schneider, E. Giasson, and L. F. S. Pinto. 2008. Solos do Rio Grande do Sul. 2.ed. Porto Alegre, EMATER/RSASCAR. 222 p. [ Links ]
Sultana, N., T. Ikeda, and R. Itoh. 1999. Effect of NaCl salinity on photosynthesis and dry matter accumulation in developing rice grains. Env. Exp. Bot. 42: 211-220. [ Links ]
Tahir, M. A., Rahmatullah, T. Aziz, M. Ashraf, S. Kanwal, and M. A. Maqsoos. 2006. Beneficial effects of silicon in wheat (Triticum aestivum L.) under salinity stress. Pak. J. Bot. 5: 1715-1722. [ Links ]
Tester, M., and R. Davenport. 2003. Na+ tolerance and Na+ transport in higher plants. Ann. Bot. 91: 503-527. [ Links ]
Toledo, M. Z., R. A. Garcia, A. Merlina, and D. M. Fernandes. 2011. Seed germination and seedling development of white oat affected by silicon and phosphorus fertilization. Sci. Agr. 68: 18-23. [ Links ]
Vieira, R. D., and N. M. Carvalho. 1994. Testes de Vigor em Sementes. Jaboticabal: FUNEP. 164 p. [ Links ]
Yoshida, S. 1981. Fundamentals of Rice Crop Science. Los Baños, IRRI. 269 p. [ Links ]
Zhu, Z. J., G.Q. Wei, J. Li, Q. Q. Qian, and J. Q Yu. 2004. Silicon alleviates salt stress and increases antioxidant enzymes activity in leaves of salt-stressed cucumber (Cucumis sativus L.). Plant Sci. 167: 527-533. [ Links ]
Received: June 01, 2014; Accepted: January 01, 2016