Introduction
Plant growth-promoting rhizobacteria (PGPR) have been widely studied for their ability to improve plant health and development. The positive activities that they exert on plants can include those that are of agricultural interest, increasing production and reducing costs, in addition to not causing harm to the environment or human health. Recent studies clearly show that the use of PGPR in agricultural crops can help to reduce the use of agrochemicals (Adesemoye & Kloepper, 2009). Similarly, PGPR can exert biological control against several phytopathogens, whether fungi, insects, bacteria or other organisms, which prevents the proliferation of plant diseases (Keel et al., 1992).
It has been proposed that PGPR can exert several mechanisms from and through the colonization of the rhizosphere. These can be defined as direct or indirect (Santoyo, Orozco-Mosqueda, & Govindappa, 2012). Indirect mechanisms can include those where bacteria produce antibiotics and other metabolites with antagonistic effect towards phytopathogens (Ahmad, Ahmad, & Khan, 2008; Santoyo, Moreno-Hagelsieb, Orozco-Mosqueda, & Glick, 2016). On the other hand, direct ones are those where bacteria have a direct impact on plant growth and development through synthesis of phyto-stimulating substances (auxins, cytokinins, indoleacetic acid, etc.) and excretion of volatile organic compounds (acetoin, sulfur compounds, etc.) (Noh-Medina, Yam-Chimal, Borges-Gómez, Zúñiga-Aguilar, & Godoy-Hernández, 2014; Hernández-León et al., 2015). These mechanisms can also include the synthesis of compounds that enhance plant immunity (jasmonic acid, 2-hydroxybenzoic acid or phytoalexins) (Ahmad et al., 2008).
The rhizosphere has been described as a highly competitive environment for microorganisms due to the need to occupy spaces and obtain nutrients (Raaijmakers, Vlami, & de Souza, 2002). Therefore, those beneficial organisms or phytopathogens that are highly competitive for colonizing and obtaining nutrients are going to proliferate in that microclimate and possibly have an effect on the development and health of the plant (Haas & Keel, 2003). Unfortunately, many of the studies that attempt to use PGPR as bioinoculants do not turn out as desired, since these strains are not competent in the rhizosphere or there is some antagonism among them, producing inconsistent results in the field (Kang, Shen, Yang, Cheng, & Zhao, 2014). Similarly, few studies follow up on bacterial inoculation experiments in the analysis of survival and competition to colonize spaces.
Therefore, one of the aims of this work was to analyze the ability of selected strains of Bacillus and Pseudomonas to colonize, survive and compete for space on maize (Zea mays) roots. The strains used in this experiment have been previously characterized and shown an excellent ability to improve plant growth, in addition to exhibiting antagonism towards various phytopathogens (Martínez-Absalón et al., 2012; Martínez-Absalón et al., 2014; Hernández-León et al., 2015). These experiments included monitoring the colonization in non-sterile soil of the strains, either separately or in a consortium, using the method proposed by Scher, Ziegle, and Kloepper (1984). Since the strains selected in this work have shown multiple mechanisms for plant growth promotion and biocontrol of phytopathogens, all this separately, another of the aims was to assess the promoting effect of the co-inoculation of Bacillus and Pseudomonas mixtures in green tomato plants in experiments in vitro.
Materials and methods
Biological material
The bacterial strains used were Bacillus thuringiensis UM96 and Pseudomonas fluorescens UM16, UM240, UM256 and UM270, which were isolated from the rhizosphere of plants and previously characterized (Martínez- Absalón et al., 2012 and 2014; Hernández-León et al., 2015; Hernández-Salmerón et al., 2016). The maize (Zea mays) and green tomato (Physalis ixocarpa Brot. ex Horm.) seeds were acquired at the “Vita” commercial seed store (Morelia, Michoacán, Mexico).
Antagonism experiment between strains
Before performing bacterial co-inoculation experiments, it was determined whether there is any antagonism in vitro between the analyzed strains (Garbeva, Silby, Raaijmakers, Levy, & de Boer, 2011). Therefore, B. thuringiensis UM96 and P. fluorescens UM16, UM240, UM256 and UM270 were cultured for 24 h, and the optical density (OD) was measured; subsequently, 1 x 106 ± 0.1 x 106 colony forming units (CFU) of each strain were inoculated. This was done in Petri dishes with nutrient agar (NA) by way of drops, 1.5 cm apart. After the drop had dried, the specimens were incubated at 28 °C for 24 h. Three replications were performed for each treatment. Next, the growth of each dish was collected separately and dilutions were performed to determine the CFU again (Garbeva et al., 2011).
Bacterial inoculation and seed treatment
To determine the colonization ability of the bacterial strains of B. thuringiensis and P. fluorescens, the method proposed by Scher et al. (1984), with some modifications, was used. This method suggests analyzing the colonization of the root or rhizoplane; however, in this study, colonization of the rhizosphere is considered. From 25 to 30 Zea mays seeds were inoculated (per treatment) with each of the Bacillus and Pseudomonas strains, as well as the following combinations (consortia) between B. thuringiensis strain UM96 and each of the P. fluorescens strains (UM96 + UM16, UM96 + UM240, UM96 + UM256 and UM96 + UM270). Each seed was inoculated with 103 to 104 CFU of each strain, and the consortia were inoculated with 105 to 106 CFU per seed, in solution with 0.1 M of MgSO4. The control seeds were inoculated with only the MgSO4 solution. The standard deviation in each inoculum was never greater than 10 %. Mean CFU per seed was determined in triplicate after culturing in Petri dishes with NA at 28 °C for 48 h.
Rhizosphere colonization experiments
Once the seeds were inoculated, each one was placed in a separate 25 x 200 mm glass tube, which had previously been filled with sand (6 cm). Next, soil (4 cm) was added and they were sealed with Parafilm. The soil was collected in Zamora, Michoacán, Mexico. Its physicochemical characteristics have been previously analyzed and published (Velázquez-Sepúlveda, Orozco- Mosqueda, Prieto-Barajas, & Santoyo, 2012). The tubes were placed in a Percival growth chamber (Percival Scientific, Perry, IA USA), with a photoperiod of 16 h light: 8 h dark at 22 °C for 30 days. Three replicates were performed for each treatment.
After 30 days of incubation, the plants were carefully removed and the roots that had grown in the sand were cut and 1-g fragments obtained. Each fragment was placed in a 1-mL vial containing 0.1 M of MgSO4; dilutions (in triplicate) were performed and it was plated in NA with100 μg of carbenicillin per mL. To confirm that the recovered colonies belong to the pre-inoculated strains, random amplified polymorphic DNA (RAPD) analysis was carried out. For this purpose, genomic DNA was isolated (Sambrook & Russel, 2001) from randomly-chosen colonies to amplify, by polymerase chain reaction (PCR), the banding pattern of each strain with oligonucleotide OPA-02 (5′-TGCCGAGCTG-3′) (Hernández-Salmerón, Prieto- Barajas, Valencia-Cantero, Moreno-Hagelsieb, & Santoyo, 2014).
Growth-promoting effect on Physalis ixocarpa Brot. ex Horm.
To determine the promoting effect of the strains separately and in a consortium, we used the Long, Schmidt, and Baldwin (2008) method of inoculating seeds (seedling vigor) on paper, with some modifications. Subsequently, green tomato seeds were sterilized and inoculated with the different bacterial culture treatments (108 CFU∙mL-1) in sterile distilled treated only with sterile distilled water for the same time. Subsequently, 15 to 20 treated seeds, as well as the respective controls, were placed on Whatman paper immersed in distilled water, wrapped in polyethylene to prevent drying, and incubated at 25 °C for seven days. The percentage of germinated seeds, the length of the hypocotyl and root, and the total weight of the seedlings were quantified. Three replications were performed for each treatment.
Results and discussion
Antagonism in vitro between strains
When attempting to perform bacterial co-inoculation experiments, one of the first aspects to be analyzed is the antagonism between strains, especially if they are of different species. Therefore, panel A (Figure 1) shows the growth of the B. thuringiensis and P. fluorescens strains, which were co-inoculated in the same Petri dish. The result shows that the development of both strains does not have any kind of antagonism, since there were no significant differences in the CFU at the end of the experiment. Panel B (Figure 1) shows the growth of the confrontation experiment where, similarly, no antagonism is observed, indicating that the growth of any P. fluorescens strain in the presence of B. thuringiensis is not affected, and vice versa.
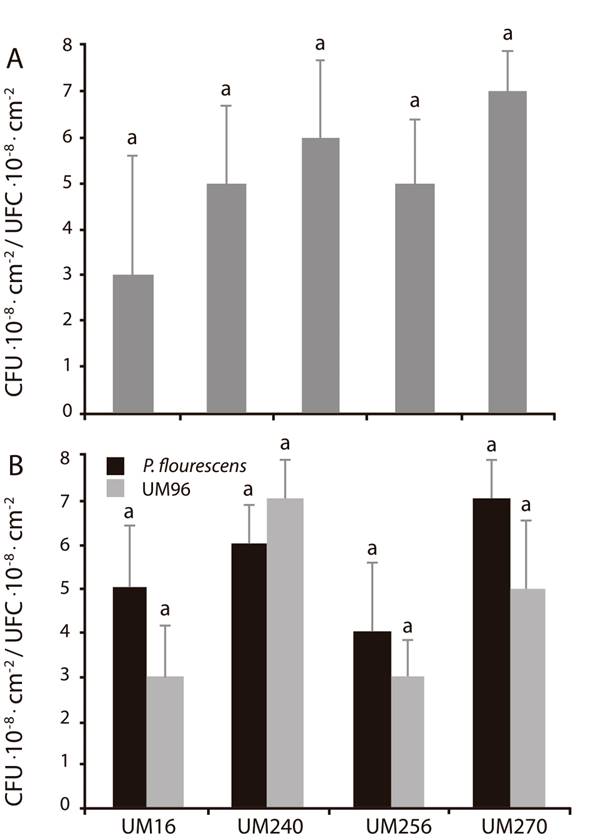
Figure 1 Competitive interaction between Bacillus thuringiensis UM96 and Pseudomonas fluorecens (UM16, UM240, UM256 and UM270). The upper panel (A) shows the growth of the strains individually in Colony Forming Units (CFU∙cm-2), while the lower panel (B) shows the values in CFU∙cm-2 of the competition and antagonism between strains. Values shown are the mean of three independent replicates. The bars show the mean ± standard error values (n. 15). Different let above the bars indicate significant differences by means of Duncan’s multiple range test (P < 0.05).
The PGPR commonly contain an array of antimicrobial metabolites. In particular, bacteria belonging to the genera Bacillus and Pseudomonas produce compounds such as antibiotics, lipopeptides, bacteriocins and volatile compounds (Glick, 2014; Santoyo et al., 2012). The analyzed bacteria belong to gram-positive (Bacillus) and gram-negative (Pseudomonas) microorganisms, so it could be hypothesized that any antibiotic that affects the cell wall could inhibit their growth.
Other studies have shown antagonistic effects between PGPR, which are excellent bioinoculants separately, but not when they are in a consortium (Kang et al., 2014). However, in this study no in vitro antagonism between Bacillus and Pseudomonas strains was directly observed, so we proceeded to study whether there was some sort of synergistic effect.
Rhizosphere colonization
Having determined that there is no antagonism between the strains, we proceeded to perform the root (or rhizosphere) colonization experiment in maize plants. Table 1 shows the results. In the case of B. thuringiensis strain UM96, 2.6 x 103 CFU were inoculated per seed and 1.42 x 107 CFU were recovered per seed, representing a significant increase. The same was observed in all four Pseudomonas strains, where it increased from between 103 and 104 to between 106 and 107, respectively.
Table 1 Individual colonization ability of the strains Bacillus thuringiensis UM96 and Pseudomonas fluorescens (UM16, UM240, UM256 and UM270).
Strain | CFU per seed | CFU∙g-1 per root |
---|---|---|
B. thuringiensis UM96 | 2.6 x 103 (± 0.23 x 103)a | 1.42 x 107 (± 0.12 x 107)e |
P. f luorescens UM16 | 1.2 x 103 (± 0.19 x 103)a | 4.40 x 106 (± 0.30 x 106)d |
P. f luorescens UM240 | 7.0 x 104 (± 0.63 x 104)b | 4.42 x 107 (± 0.48 x 107)e |
P. f luorescens UM256 | 2.0 x 104 (± 0.17 x 104)b | 4.07 x 107 (± 0.39 x 107)e |
P. f luorescens UM270 | 4.0 x 104 (± 0.39 x 104)b | 6.52 x 107 (± 0.54 x 107)e |
Values shown are the mean of three independent replicates with n ≥ 25 seeds inoculated per replica. The standard deviation is shown in parentheses (±). Different letters indicate significant differences at α = 0.05.
Having analyzed the colonization ability of the strains separately, the Bacillus-Pseudomonas consortia were studied. The experiment results showed high rhizosphere colonization ability in the four consortia (Table 2). For example, UM96 + UM16, UM96 + UM240 and UM96 + UM256 recorded an increase of two orders of magnitude in the CFU recovered per gram of root. In the case of the UM96 + UM270 consortium, 1.0 x 106 CFU were inoculated in seed and 1.9 x 107 CFU were recovered, which shows a significant increase. Thus, it can be seen that the analyzed rhizobacteria show an excellent ability to colonize the root of maize plants, either separately or in a Bacillus-Pseudomonas consortium. This confirms that the system proposed by Scher et al. (1984) is an excellent method to assess the colonization ability of rhizobacteria. Therefore, it was decided to assess this ability in isolated strains, whether in sterile soil or where they compete with other microorganisms.
Table 2 Colonization ability of the consortia between Bacillus thuringiensis UM96 and each of the strains of Pseudomonas fluorescens (UM16, UM240, UM256 and UM270).
Consortia | CFU per seed | CFU∙g-1 per root |
---|---|---|
UM16 + UM96 | 4.0 x 105 (± 0.38 x 105)a | 1.4 x 107 (± 0.07 x 107)c |
UM240 + UM96 | 5.3 x 105 (± 0.46 x 105)a | 2.5 x 107 (± 0.17 x 107)c |
UM256 + UM96 | 4.6 x 105 (± 0.35 x 105)a | 3.4 x 107 (± 0.33 x 107)c |
UM270 + UM96 | 1.0 x 106 (± 0.08 x 106)b | 1.9 x 107 (± 0.11 x 107)c |
Values shown are the mean of three independent replicates with n ≥ 25 seeds inoculated per replica. The standard deviation is shown in parentheses (±). Different letters indicate significant differences at α = 0.05.
The rhizosphere is a microecosystem where a myriad of interactions take place. This soil area, which is directly influenced by root exudates, can be divided into zones of greater interaction, such as the rhizoplane (Scher et al., 1984). The rhizoplane is the surface part of the root where various microbial species interact with the plant. They can also carry out pathogenic or beneficial interactions; therefore, if beneficial organisms have a good ability to colonize the rhizosphere, they can displace potentially pathogenic microorganisms (Santoyo et al., 2012). Therefore, one of the characteristics that strains intended for use as bioinoculants must have is a good ability to colonize the rhizosphere (Scher et al., 1984).
The bacterial species studied have previously shown excellent antagonism and biocontrol ability against various phytopathogens (Martínez-Absalón et al., 2012 and 2014; Hernández-León et al., 2015), including Botrytis cinerea, which causes gray mold in more than 200 plant species (Nambeesan et al., 2012). In the case of the Pseudomonas strains, it has been observed that they can promote the growth of plants such as Medicago truncatula through excretion of various diffusible (siderophores, HCN, 2,4-diacetylphloroglucinol, among others), and volatile metabolites, mainly sulfur compounds such as dimethyl sulfoxide (Hernández- León et al., 2015), while the strain UM96 is an excellent producer of chitinases, involved in biocontrol of the phytopathogen B. cinerea (Martínez-Absalón et al., 2014).
Therefore, the synthesis of multiple compounds or metabolites, by the strains analyzed, could be responsible for the high ability to colonize different areas of the rhizosphere, including the rhizoplane. This includes survival and competition in the soil where they cohabit with other microorganisms to occupy spaces, which is known as a mechanism of inhibition of phytopathogens, as well as an indirect way to induce promotion of plant growth and health. Therefore, the bacteria can access the root exudates or nutrients, and thus have beneficial effects on the plant through production of phytostimulants (Glick, 2014; Santoyo et al., 2012).
Growth-promoting effect in seedlings of Physalis ixocarpa Brot. ex Horm
To determine the promoting effect of the strains 9009 experiments were performed on green tomato (Physalis ixocarpa Brot. ex Horm.) seeds. The results show that the strains did not promote germination (data not shown). However, it was observed that the Pseudomonas strains, separately, increased hypocotyl and root length, as well as the total fresh weight of the seedlings (Figure 2), while B. thuringiensis UM96 only showed significant difference for root length.
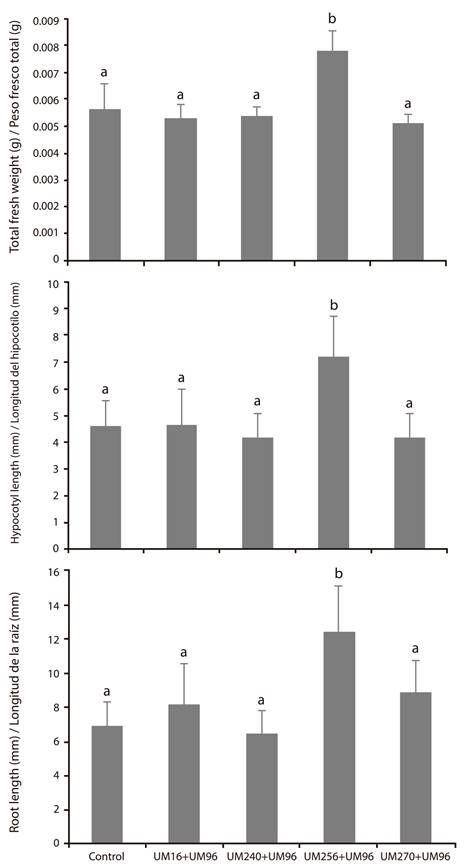
Figure 2 Individual growth-promoting effect of the strains Bacillus thuringiensis UM96 and Pseudomonas fluorecens (UM16, UM240, UM256 and UM270) on the growth of seedlings of Physalis ixocarpa Brot. ex Horm. Values of fresh weight (g) and root and hypocotyl length (mm) are shown. Values shown are the mean of three independent replicates. The bars show the mean ± standard error values (n ≥ 15). Different letters above the bars indicate significant differences by means of Duncan’s multiple range test (P < 0.05).
With respect to the promoting effect of the co-inoculations, Figure 3 shows the results, where it can be seen that the combinations UM96 + UM16, UM96 + UM240 and UM96 + 270 did not promote the development of the green tomato seedlings. The UM96 + UM256 consortium was the only one that showed significant increases in root and hypocotyl length, as well as the total fresh weight of the seedlings. These results show that certain combinations of Bacillus and Pseudomonas can exert a repressive effect on plant growth promotion, while some can maintain such an effect separately or in a consortium, such as the UM96 + UM256 mixture.

Figure 3 Growth-promoting effect of the consortia between Bacillus thuringiensis UM96 and each one of the strains of Pseudomonas fluorecens (UM16, UM240, UM256 and UM270) on the growth of seedlings of Physalis ixocarpa Brot. ex Horm. Values of fresh weight (g) and root and hypocotyl length (mm) are shown. Values shown are the mean of three independent replicates. The bars show the mean ± standard error values (n ≥ 15). Different letters above the bars indicate significant differences by means of Duncan’s multiple range test (P < 0.05).
As far as is known, there is no information about any direct plant growth-promoting effect by B. thuringienesis. Therefore, it was not surprising that UM96 did not increase the growth of the tomato seedlings. However, it is known to have the ability to produce chitinases that can restrict the development of phytopathogenic fungi and thus have an indirect beneficial effect (Martínez- Absalón et al., 2012 y 2014). This activity can supplement those mechanisms by which P. fluorescens strains can antagonize fungi such as B. cinerea (Hernández-León et al., 2015); therefore, it could be hypothesized that the co-inoculation of strain UM96 plus one of Pseudomonas, in a rhizospheric environment, could provide the plant with better protection against pathogen attack.
On the other hand, there are studies that propose the use of mixtures of beneficial bacteria to enhance plant growth-promoting abilities (Raupach & Kloepper, 1998). However, the results of this work show that not all Bacillus-Pseudomonas combinations can work, since three combinations (UM96 + UM16, UM96 + UM240, UM96 + UM270) did not promote seedling development; on the contrary, the promoting effect of Pseudomonas was inhibited by the presence of strain UM96. The only combination that was successful and that maintained a promoting effect was UM96 + UM256.
Kang et al. (2014) found in tomato plants that Bacillus pumilus WP8 and Erwinia persicinus RA2 strains, separately, are excellent plant growth promoters, but combined they do not have these effects and they also negatively affect biocontrol activities. By analyzing this antagonistic effect between strains in more detail, it was observed that biofilm formation was harmed in both strains. This formation is an important characteristic to colonize spaces in the rhizosphere; therefore, it has been indirectly associated with biocontrol ability and plant growth promotion (Beyeler, Keel, Michaux, & Haas 1999; Bianco et al., 2006; Duca, Lorv, Patten, Rose, & Glick, 2014). In this research, the P. fluorescens strains are biofilm producers (Hernández-León et al., 2015), so a similar antagonistic effect on the co -inoculations in the plant could be assumed. However, this effect was not observed in confrontation experiments, so the tomato plants could be producing a compound (root exudate) that triggers defense activities in the bacteria, as a response to competing for spaces. This is a hypothesis that deserves further laboratory work.
Conclusions
Based on the results obtained, it is concluded that the strains of B. thuringiensis and P. fluorescens have excellent colonization ability, either individually or in a consortium. Similarly, it was found that only the UM16 + UM96 mixture showed a growth-promoting effect on green tomato seedlings.