INTRODUCTION
Tropical climates through the Quaternary have been the result of a complex network of interactions among local, regional, and global drivers acting at different temporal and spatial scales (Bradley, 2015). During the Holocene (the last ~11,000 years), although conditions have been warmer on average, precipitation has represented an element introducing large amounts of variability into the tropical climate systems (e.g., Hodell et al., 1991; Haug et al., 2001; Tedesco and Thunell, 2003; Bernal et al., 2011). In the Yucatan Peninsula, precipitation variability through the Holocene has been largely documented based on sedimentary evidence (Hodell et al., 2001; Anselmetti et al., 2007; Mueller et al., 2009; Wahl et al., 2014). Apparently, the regional history has been characterized by alternating periods of wetness and drought that were variable in length, and a high influence of human populations on the landscape (Leyden, 2002).
Despite the relatively large amount of paleoenvironmental information that is available on the Holocene in the Yucatan Peninsula, little is known on the environmental history of the middle elevations (premontane and lower montane altitudinal belts of Holdridge et al., 1964). Mid altitude environments might have been of vital importance for the survival of natural population during times of extreme environmental hardship (e.g., Heinrich events during the Pleistocene, Correa-Metrio et al., 2012). High topographic diversity and steep environmental gradients over relatively short distances make middle elevations regions with high potential for harboring microrefugia (Bush, 2002; Rull, 2009; Correa-Metrio et al., 2014b). The orographic gradient offers conditions where rainfall is more likely, and therefore, the climatic trends of these regions are not necessarily coupled with those of the low flat terrains. However, there is still need to evaluate the role that these regions have played in terms of biodiversity preservation through time based on hard evidence. A first step towards this evaluation would be the understanding of local environmental trajectories in the context of regional climatic variability.
Much of the information gap derives from the fact that lakes in middle elevation are rather scarce. The Lacandón Forest region, southeastern tropical Mexico, is characterized by a series of karst water bodies located in middle elevations, providing a good setting for studying the evolution of local and regional environments through time. In this study, we aimed to infer environmental changes during the late Holocene (the last ~4,000 years). Geochemical indicators are useful to infer past conditions of sediment deposition (Last and Smol, 2001). We used, C, Ca, N, Rb, Sr, Ti, and Zr to infer erosion intensity and organic matter provenance, characteristics that provide information on past precipitation regimes and lake trophic status. Cladocerans are microcrustaceans whose chitinous shells are identifiable at species level and preserve well in lake sediments (Alonso, 1996; Smol et al., 2003). Given their environmental specificity (Table 1), cladocerans are good indicators for reconstructing limnological conditions (Smol et al., 2003). We use changes in the cladoceran assemblages for infering water depth and lake trophic status. Our main questions are: i) What has been the general trend of precipitation in the Lacandón Forest through the last 4,000 years?, ii) Apart from the general trend, has there been a systematic higher frequency mode of variability? and iii) has the climatic variability in the middle elevations been linked to the variability reported for the lowlands?.
Table 1 Environmental preferences of the principal cladoceran species from Lake Lacandón (environmental affinities from Bērzinš and Bertilsson, 1989; Alonso, 1996; Lotter et al., 1998).
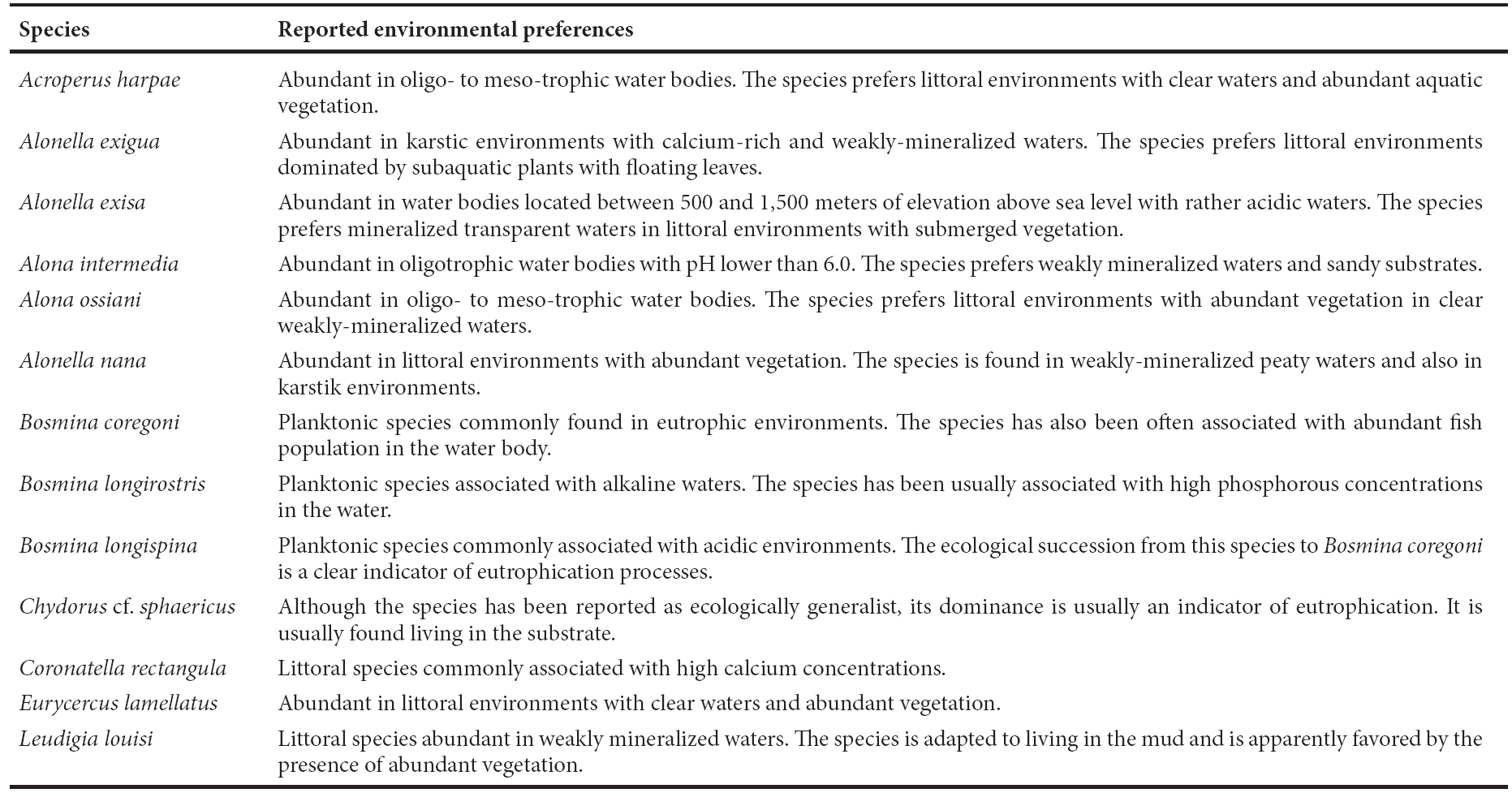
METHODS
Study area
Lake Lacandón is located in the State of Chiapas, Mexico (17°00'55.80"N, 91°35'23.34"W, 810 m a.s.l.) near the protected area of Nahá, northwest region of the Lacandón Forest (Figure 1). With an area of ~1 ha and a maximum depth of ~3 m in its central part, the lake lies on a karstic platform of marine Cretaceous origin, with inclusions of continental lutites and sandstones (Padilla y Sánchez, 2007). Mean annual precipitation in the region is ~1,860 mm, with 92% of it concentrated between May and December (climate data from Lacantún meteorological station, Servicio Meteorológico Nacional, 2016). Mean annual temperature is 23.6 °C, with the coldest and warmest temperatures at 20.9 and 25.6 ºC during January and May, respectively. The regional vegetation is a mosaic composed of tropical rain forest, cloud forest, coniferous forest, secondary vegetation and large agricultural areas and pastures (Rzedowski, 2006).
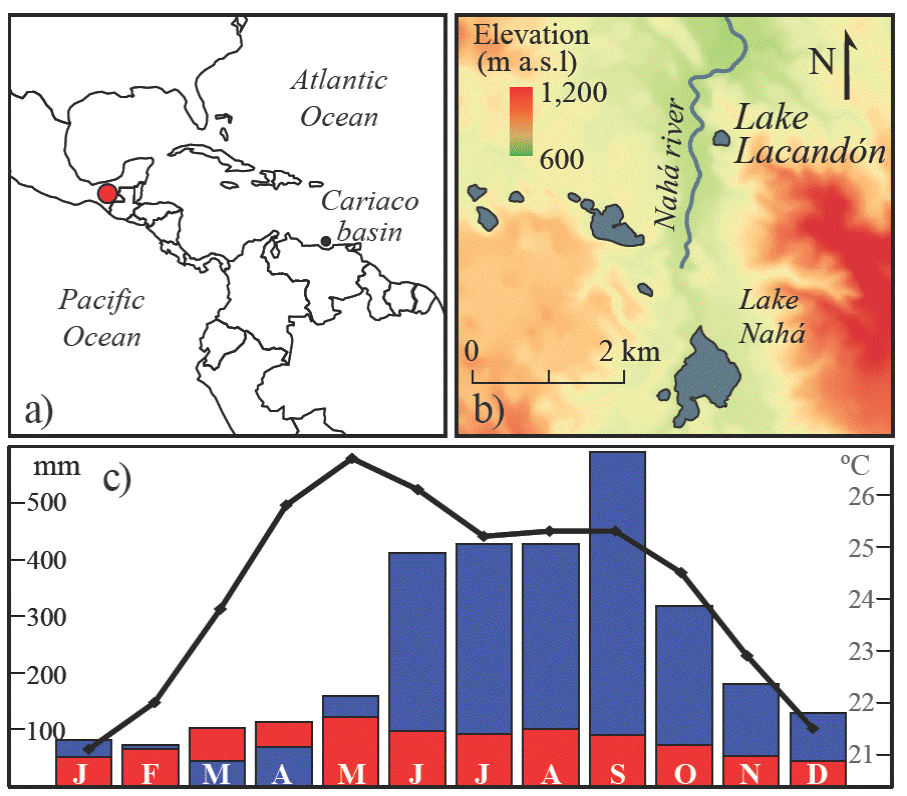
Figure 1 Study area. a) The localization of the Lacandón Forest in southeastern Mexico. b) Details on the topographic context of Lake Lacandón. c) Climograph from Lacatún meteorological station; monthly precipitation (blue bars) and potential evapotranspiration (red), and monthly mean temperature (black line); data from Servicio Meteorológico Nacional (2016).
Data collection and analyses
In July 2012 a 370 cm long sedimentary sequence, core LAC12-I, was recovered from Lake Lacandón using a modified Livingstone piston corer (Colinvaux et al., 1999). Given the consistency of the sediment, the core was bagged in the field and samples were split into a half that was stored as archive, and the other was used for the paleoenvironmental analyses. The lithology was described qualitatively (color, texture and reaction to HCl). Chronological control of the sequence was derived from two pollen extracts submitted to AMS (accelerator mass spectrometry) radiocarbon dating. The samples were extracted from the bottom of the core (369 cm below lake floor, blf hereafter) and from its middle part (118 cm blf), and the analysis was carried out at Beta Analytic Inc. (Miami, Florida). Radiocarbon dates were calibrated and a Bayesian depth-age model was built using Bacon (Blaauw and Christen, 2011). All dates were expressed as calendar years before present (BP hereafter).
The core was sub-sampled every 5 cm for cladoceran and geochemical analysed. For cladoceran counts, 1 cc samples were defloculated using KOH, and carbonates were dissolved with HCl. Samples were then analyzed under a microscope at magnifications 100X and 400X, aiming at a count of 200 individuals containing all the remains found (headshields, shells, postabdomens, postabdominal claws, and ephippia). Cladocera were identified to species level using illustrated guides (Elías-Gutiérrez et al., 1997; Szeroczyńska and Sarmaja-Korjonen, 2007; Elias-Gutierrez et al., 2008). Cladoceran counts were expressed in terms of relative abundance and plotted stratigraphically using the software C2 Data Analysis (Juggins, 2007). For geochemical analyses, 1 cc samples were oven-dried at 40 °C, homogenized and ground with an agate mortar. Concentrations of Ca, Rb, Sr, Ti and Zr were measured with a portable Thermo Scientific NITON FXL 950 X-Ray Fluorescence (XRF) analyzer. Total carbon and nitrogen were measured by duplicate using lyophilized sediment from the same depths through an elemental analyzer CNHS/O Perkin Elmer 2400 series, and the ratio C:N was calculated.
Detrended Correspondence Analysis (DCA) (Hill and Gauch, 1980) was performed on the biological data using all species found to summarize environmental changes along the record (Correa-Metrio et al., 2014a). The association among geochemical variables was estimated through Pearson correlation coefficients (Legendre and Legendre, 1998), whose p-values were corrected for family wise error (Zar, 1999). All statistical analyses were conducted using the statistical software environment R (R Core Team 2015), particularly package vegan (Oksanen et al., 2015).
RESULTS
From the bottom of the record (370 cm blf) to 270 cm blf, the sediment consists of silty clays with interbedded bands of sand or organic mud (Figure 2). From 270 to 200 cm blf, the sediments were composed of coarser materials, mainly a 20 cm wide sand layer (from 270 to 250 cm blf) and silts from 250 to 200 cm blf. From 200 cm blf to the top of the record, sediments were mainly composed of clays with two layers of organic mud, one from ~200 to 162 cm blf, and the other representing the upper ~15 cm of the record. According to the calibrated age model (Table 2 and Figure 2), the sedimentary record spans the last ~4,000 years, i.e. the late Holocene (Walker et al., 2012), with high (low) sedimentation rates in the lower (upper) half of the core.

Figure 2 Chronological model. Age in years before present (BP) as a function of depth in cm below lake floor. The lithological description is included on the right.
Approximately 70,000 individual cladocerans were found in the 77 analyzed samples. The record was composed of 16 species: three planktonic or open water species (family Bosminidae) and 13 littoral species (family Chydoridae) (Figure 3 and 4). The family Chydoridae was present through the entire record, except for the interval between 261 and 194 cm blf (~3,100 and 2,550 BP) where samples were barren of cladocerans. The family Bosminidae was abundant from 170 cm blf (~2,350 BP) to the top of the core (present).

Figure 3 The cladoceran assemblages and geochemical signal of the sedimentary sequence from Lake Lacandón. Cladoceran concentrations in individuals per cm3. Cladoceran habitat preference from Alonso (1996). Cladocera zones are represented by roman numerals superimposed on the abundances of Acroperus harpae. Concentrations of C, Ca, N, and Ti are given in weight percentage.

Figure 4 Body parts of the most representative Cladocera taxa found in the sedimentary record of Lake Lacandón. An individual size scale is shown for each photograph. a) Shell of Bosmina coregoni. b) Shell of Bosmina (E.) longispina. c) Shell of Bosmina longirostris. d) Headshield of Eurycercus lamellatus. e) Headshield of Leydigia acanthocercoides. f) Headshield of Alona intermedia. g) Headshield of Coronatella rectangula. h) Headshield of Graptoleberis testudinaria. i) Shell of Chydorus cf. sphaericus J. Headshield of Leydigia acanthocercoides.
The lengths of DCA, Axes 1 and 2 in the ordination of species (samples) were 4.94 (3.16) and 3.94 (2.26) standard deviations (SD), respectively (Figure 5). The highest scores of Axis 1 corresponded to Leydigia louisi, followed by Camptocercus dadayi and Coronatella rectangula, whereas the lowest scores corresponded to Bosmina longirotris, Eurycercus sp. and Bosmina (E.) longispina (Figure 5). The highest scores of DCA Axis 2 corresponded to Acropercus harpae, Graptoleberis testudinaria and Alonella exigua, whereas the lowest scores were assigned to Leydigia louisi, Coronatella rectangula and Alonella nana (Figure 5). Samples older than 2,500 BP were ordinated in quadrants one and four, whereas those younger than 2,500 BP clustered in quadrants two and three (Figure 5).

Figure 5 Detrended Correspondence Analysis (DCA) of the cladoceran assemblages of the sedimentary sequence from Lake Lacandón. a) Species ordination, with the gray rectangle showing the space occupied by samples (panel b). b) Samples ordination; each samples was labeled with the age derived from the chronological model. c) Axis 1 sample scores along age. The columns on the left and right sides show Cladocera zones and lithology, respectively.
Concentrations of the analyzed elements were highly variable through the record (Figure 3). C and N showed high variability but a general increasing trend towards present. Ca, Sr and C:N ratio showed variable high values from the bottom of the record up to ~180 cm blf, and a less variable lightly decreasing trend from ~180 cm blf to the top of the record. Positive highly significant correlations were determined between C and N, C:N and Ca, Ti and Rb, Rb and Zr, and Ti and Zr (Table 3). Negative highly significant correlations were determined between N and C:N, N and Ca, Ca and Rb, Ca and Ti, Ca and Zr, and Sr and Ti (Table 3).
DISCUSSION
The environmental signal of cladocerans and geochemistry in the sediments of Lake Lacandón
The most evident feature inferred from the cladoceran assemblages of Lake Lacandón was a clear distinction between species associated with littoral and open water areas of the lake. Our a priori distinction of these two groups (Table 1 and Figure 3; environmental affinities from Bērzinš and Bertilsson, 1989; Alonso, 1996; Lotter et al., 1998) was confirmed by the ordination of species along DCA Axis 1 (Figure 5). DCA Axis 1 captures the principal underlying environmental factor that drives ecological changes through time (Correa-Metrio et al., 2014a), which in Lake Lacandón seems lake size and depth. Species reportedly associated with littoral areas were mostly ordinated on the positive side of Axis 1, and planktonic species associated with deeper waters (namely Bosmina spp.) obtained negative scores. Thus, according to our interpretation of the cladoceran community, at Lake Lacandón, the main environmental factor that controlled Cladocera through time was lake size. Lake lowstands cause the development of dense macrophyte mats that in turn favors the dominance of the species we classified as littoral. Conversely, as lake size increases, there is also a slight deepening of its central area that forces microphytes to retreat towards the shallower edges, favoring the dominance of Bosmina spp. Indeed, DCA Axis 1 scores showed a time pattern that was closely associated with total percentage of littoral species (Figure 6). Thus, we interpret DCA Axis 1 as representative of relative lake size/depth.

Figure 6 The environmental history from the sediments of Lake Lacandón. a) Cladocera zones; b) cladoceran concentration, number of individuals per cm3; c) percentage of littoral and planktonic cladoceran species (environmental affinities from Alonso, 1996), and insolation seasonality (summer - winter, in red) (insolation data from Paillard et al., 1996); d) Detrendend Correspondence Analysis, Axis 1 sample scores in standard deviations (SD) plotted in time as representative of relative lake size/depth; e) percentage of lithic grains in the sediments of the North Atlantic (Bond et al., 2001); f-g) carbon and nitrogen weight percentages, respectively; h) C:N ratios, with the dashed vertical line indicating a value of 10; i-j) calcium and titanium concentration, in weight percentage, in the sediments of Lake Lacandón; k) titanium weight percentages, in the sediments of Cariaco basin, northern Venezuela (Haug et al., 2001). The column on the right hand side shows core lithology.
The relationships among geochemical indicators were consistent with a strong climatic influence over these proxies. Ti is an element that has been traditionally interpreted as an indicator of erosive activity (Haug et al., 2001). In tropical environments such as the Lacandón Forest, where the main erosive factor is the runoff produced by rainfall, Ti sequences can be inferred as changes in total annual precipitation and/or seasonality. Such is the case of our Ti record, whose high positive correlation with Zr and Rb (Table 3), both of them conservative lithogenic elements (Boës et al., 2011), offers further support to our interpretation. Also, the negative significant correlation of Ti with Ca and Sr, both indicative of authigenic mineral precipitation during period of low lake level (Eugster and Hardie, 1978), point to Ti as an indicator of terrigenic input into Lake Lacandón.
Lake trophic status through the last 4,000 years
Cladoceran assemblages composition through time suggest mesotrophic conditions during most of the studied time period (Alonso, 1996; Lotter et al., 1998). An exception to this pattern was the short period between ~1,800 and 1,600, when high abundances of Bosmina longirostris suggest eutrophic conditions (Bērzinš and Bertilsson, 1989, Figure 3). However, it is difficult to infer trophic changes because of the dominance of lake size on the cladoceran signal of our record. Nevertheless, geochemical indicators also suggest mesotrophic conditions in the lake through the last 4,000 years. Theoretically, organic carbon to nitrogen ratios above 20 and below 10 are indicative of allochthonous and autochthonous carbon input, respectively (Meyers, 1997). The calculated C:N ratios for Lake Lacandón never dropped below 10 (absolute minimum 12.1 through the entire record, Figure 6), supporting our interpretation of mesotrophic conditions through the record. However, these ratios were based on total carbon, implying that our report represents the scenario of the maximum organic carbon to nitrogen possible values (i.e., assuming all the carbon had an organic origin). From the bottom of the record to ~2,000 BP, C:N ratios reached values up to 68, which would imply substantial input of terrigenic material. Such input would be the product of the development of a dense forest canopy around the lake or high runoff from the catchment. However, our record shows Ti concentrations antiphasing C:N ratios, suggesting that increases in the latter occurred during times of rather dry conditions. It is possible that C concentration during times of drought increased as a result of calcium carbonates precipitation, a process that characterizes dry episodes in the area because of the regional lithology (Hodell et al., 2008). In fact, the correlation between C:N ratios and Ca concentration in the sediments of Lake Lacandón was highly significant (Table 3).
The temporal evolution of Lake Lacandón
Cladoceran assemblages through the stratigraphic profile allowed the identification of five time periods, denoted by roman numerals from the bottom to the top of the record, that were characterized by relatively homogeneous environmental conditions (Figures 3, 5, and 6). This zonation of the stratigraphic profile was further supported by the DCA Axis 1 scores (Figure 5) and the lithology of the sedimentary package (Figures 5 and 6). As in pollen analysis, we define a cladoceran zone as a time period characterized by relatively stable biological assemblages, which would in turn reflect relatively uniform environmental conditions (Birks and Birks, 1980). High dynamism is an implicit characteristic of most biological associations, and transitions between environmental states are rarely discrete (Delcourt and Delcourt, 1991). However, we discretized the environmental history to facilitate the description and understanding of environmental settings reflected in our sedimentary record.
Zone I (from the bottom of the record to 275 cm below lake floor, ~3,950 to 3,200 BP) was characterized by an assembly dominated by littoral cladoceran species (Van Damme and Eggermont, 2011), mainly Alona ossiani, Chydorus cf. sphaericus, and Acroperus harpae (Figure 3). These species are known for their tolerance to acidic pH levels and low concentrations of dissolved oxygen in the water (Alonso, 1996; Lotter et al., 1998). They are usually pioneers in early stages of lake development, representing the first species to invade/colonize unoccupied or heavily modified environments (Zawisza and Szeroczyńska, 2007). Thus, this zone probably represents the early stages of the formation of the lake or its recovery from a total dry out. In any case, high variability of species abundance was probably associated with unstable environmental conditions and a primary successional process. Indeed, this time period was characterized by highly variable scores of DCA Axis 1 (Figure 5), although the scores remained in the positive extreme of the axis. The evidence points to a small and shallow lake with abundant macrophytes covering most of its extension.
Ca and Ti concentrations through Zone I suggest that this time period was characterized by a general drought with three wet intermissions: at the bottom of the record, between ~3,750 and 3,550 BP, and at ~3,350 BP (Figure 6). These wet episodes were evidenced by peaks of Ti antiphasing low concentrations of Ca, indicating high erosion levels (Haug et al., 2001) and low precipitation of carbonates (Hodell et al., 1995), respectively. Dry conditions have been reported for the Yucatan Peninsula from ~4,500 to 3,000 BP, with special note of a particular drought that took place at 3,500 BP (Mueller et al., 2009; Carrillo-Bastos et al., 2010), suggesting dry conditions were of regional nature. Indeed, records from the northern Neotropics suggest that the drought that characterized this time period had hemispheric scope (e.g., Curtis et al., 1999; Haug et al., 2001; Tedesco and Thunell, 2003). Particularly, our Ti and Ca records seem synchronous with the high frequency variability of the Ti record from Cariaco Basin, northern Venezuela (Figure 6). It is probable that dry conditions and/or intensive human occupation were associated with relatively open vegetation (e.g., Curtis et al., 1998; Mueller et al., 2009), causing higher erosion during time intervals associated with wetter regional climates (Anselmetti et al., 2007).
Zone II (275 to 200 cm below lake floor, ~3,200 to ~2,600 yr BC) was almost entirely barren of cladocerans. The only species found was Bosmina (E.) coregoni with abundances lower than 150 individuals (Figure 3), making interpretations based on the biological evidence unreliable. The high content of sands and silts in the sediments, and increases of Ti concentration (Figure 6) suggest a high pluvial erosion level of the basin. Indeed, the layer of fine sands between 270 and 250 cm blf could have resulted from a high water of river Nahá that runs only a few tens of meters from Lake Lacandón. According to palynological evidence from the Yucatan Peninsula, the regional vegetation showed a trend towards the dominance of disturbance and open taxa from ~4,500 to 3,000 BP (Curtis et al., 1998; Anselmetti et al., 2007; Mueller et al., 2009). This vegetation change was likely regional in nature, leading to soils more exposed to the erosive power of rainfall. Thus, when relatively humid regional conditions returned at ~3,000 BP, they produced high erosion levels in the basin of Lake Lacandón, producing the layers of rather coarse material that characterized Zone II.
Zone III (200 to 162 cm below lake floor, ~2,600 to ~2,300 BP) was characterized by a total dominance of littoral Cladocera species in a matrix of organic mud (Figure 6). DCA Axis 1 scores suggest extremely low lake levels, which together with high concentrations of C offer evidence of the initial stages of lake development. These attributes are very similar to those found in the bottom of the record, and the zone probably represents the starting of a primary successional process of recolonization following what seems to have been a major disturbance in Zone II. High and low concentrations of Ti and Ca suggest the inception of relatively wet conditions after the environmental instability that characterized zones I and II. The Cariaco Basin Ti record shows a progressive decrease of moisture in the northern Neotropics through the Holocene, with specially dry and variable conditions between 4,000 and 2,400 BP (Haug et al., 2001). This anomaly in the progressive trend was probably the result of an intensification of the El Niño activity (Moy et al., 2002), which was also probably responsible for part of the environmental instability expressed in zones I and II of our record. The evident establishment of conditions that favored the recovery of the cladoceran community in Lake Lacandón was probably the result of the stabilization of regional conditions after the aforementioned dry period.
Zone IV (160 to 100 cm below lake floor, ~2,300 to ~1,600 BP, Figure 3) was characterized by an increase of planktonic species in the cladoceran communities, showing a trend towards a larger lake with open water areas. This expansion and therefore slight deepening of the lake was likely result of higher moisture availability evidenced in high Ti concentrations (Figure 6). Bosmina longirostris, a specie associated with high trophic levels (Bērzinš and Bertilsson, 1989), dominated the planktonic component of the cladoceran assemblages in the upper part of the zone. Additionally, C and N increased whereas C:N ratios and Ca concentrations remained stable, evidencing an authigenic provenance of the C in the sediments. Thus, this time period was characterized by a highly-productive enlarging deepening lake. Relatively wet conditions have been reported during this time period for the Yucatán Peninsula (Curtis and Hodell, 1996; Mueller et al., 2009; Carrillo-Bastos et al., 2013), implying a regional pattern of mild climatic conditions.
Zone V (from 100 cm below lake floor to mud-water interface, the last ~1,600 years) was characterized by contrasting trends in the cladoceran community and the Ti concentrations (Figure 3). The cladoceran community has been progressively enriched in planktonic components, which together with a decreasing trend of DCA Axis 1 scores implies a general increasing in lake level (Figure 6). Insolation seasonality at the latitude where Lake Lacandón lies has been decreasing through the last 4,000 years (Figure 6), with decreasing and increasing summer-fall and winter-spring insolation, respectively (Berger and Loutre, 1991). The decrease of summer insolation, directly associated with summer rainfall given the seasonal northern position of the Intertropical Convergence Zone, has been associated with diminishing total annual precipitation in the northern Neotropics (Hodell et al., 1995; Haug et al., 2001). This pattern is reflected in the Ti concentration of sedimentary package of Lake Lacandón, which shows a general trend towards a drier climate (Figure 6). However, increasing insolation during the dry season would facilitate advection of water vapor and orographic precipitation causing winter and spring showers. A slight increase of precipitation during months of water deficit (Figure 1) would have a conservative effect on lake water, explaining the increasing trend of lake size in a context of decreasing total annual precipitation.
Several peaks of littoral species dominance through the last 1,600 years suggest episodes of regression to low lakes levels. Interestingly, these peaks showed coincidence with ice rafted deposits in the North Atlantic (Figure 6). Although these peaks have occurred throughout the Holocene (they configure a periodicity known as Bond Cycles, Bond et al., 2001), they seem to manifest in our record only during the last 1,600 years. Increased discharge of cold fresh water in the North Atlantic that were evidenced the oceanic sediments would have caused ralentization of the Atlantic Turnover Circulation, bringing drier than average conditions to the Yucatán Peninsula and adjacent areas (Hodell et al., 2008; Correa-Metrio et al., 2012). These dry episodes were overimposed on the long term drying trend reflected in the decreasing Ti concentrations (Figure 6), a pattern that has been documented in other records from southern Mexico (Bernal et al., 2011). From the bottom of the record to 1,600 BP regional moisture availability was higher given a more northerly position of the Intertropical Convergence Zone (Haug et al., 2001). This pattern probably counteracted precipitation decreases associated with North Atlantic fresh water discharge. It is possible that at ~1,600 BP the regional precipitation reached a level low enough as to allow the ice discharges to manifest in regional lake levels. Nevertheless, the latter statement constitutes a hypothesis that needs further testing.
CONCLUSIONS
The sedimentary record of Lake Lacandón offers evidence of a complex and highly variable climatic history through the last 4,000 years. Geochemical and biological evidence suggest a mesotrophic water body through the studied time period. Ti concentrations through the stratigraphic profile suggest that erosion, and therefore probably rainfall, in the Lacandón Forest has been closely associated with hemispheric patterns of climatic variability. Our record showed a long-term trend towards lower total annual precipitation that coincides with the southward displacement of the Intertropical Convergence Zone through the Holocene (Haug et al., 2001). Fluctuations in both the composition of the cladoceran assemblages and the concentrations of Ti and Ca suggest a higher-frequency climatic variability possibly associated with periods of intense El Niño activity (Moy et al., 2002) and/or fresh water discharges in the North Atlantic (Bond et al., 2001). Thus, our record highlights the complexity of the environmental evolution of tropical areas, where climates are the result of the confluence of diverse drivers whose relative contribution may change through time and space.
The evolution of the cladoceran community of Lake Lacandón through time suggests a lake that has been growing in size through the last 4,000 years. This pattern seems contradictory with the regional and local trends towards drier conditions through the Holocene (Hodell et al., 1991; Haug et al., 2001). However, it is possible that the decreasing amount of precipitation through the year were offset by a lowered seasonality. Increasing insolation during the dry season would cause advection of moist air from the ocean towards the mountains, where humidity would eventually precipitate given the elevation gradient. Thus, despite regional climatic trends, the sedimentary record of Lake Lacandón reinforces the idea of mountain flanks harboring disjunct climates and sheltering natural populations from rapidly changing climates (Bush, 2002; Correa-Metrio et al., 2014b). Our results call for prioritizing the conservation of mountain flanks because, as pointed by the fossil evidence, they would offer refugia to natural populations during periods of instable climates.