INTRODUCTION
The La Colorada Mine is an important gold and silver deposit located in the Sonora state, northwestern Mexico. Mineralization occurs along fault controlled veins and related breccias. High-grade veins were intermittently mined through underground works from 1740 until 1993 when a large-scale mining operation began. A first stage of open-pit mining, from 1993 to 2000, produced three large pits named Gran Central, La Colorada, and El Crestón. Geology of the La Colorada region is described in some papers and technical reports e.g.Lewis et al. (1995), Zawada (1998), Zawada et al. (2001), McMillan and Hodder (2008), McMillan et al. (2009), which are devoted to explaining the history of the mining and the geology of the area, and to characterizing the ore deposits. Although the deposit is structurally controlled, few structural data have been reported apart from the attitude of the main ore veins and some major faults. In view of the expansion of the mining works, a better knowledge of the deposit is required and a detailed structural work was carried out, taking advantage of artificial outcrops in the open pits. Detailed structural data combined with field observations and structural analysis were used to define the main fault systems in the area, the relative age of faulting, as well as the role of the geological structures in the origin and later evolution of the ore deposits. Based on comparisons with adjacent areas, a model for the tectonic evolution in the area is proposed.
REGIONAL SETTING
The study area is located in the Cortés terrane near the border of the Caborca terrane (Figure 1). The Caborca terrane is made by Paleoproterozoic (1.8 to 1.7 Ga) metamorphic basement, intruded by ~1.4 and ~1.1 Ga granites (e.g.Anderson and Silver, 2005), covered by a thick Upper Proterozoic to Lower Permian shelf succession (e.g.Stewart et al., 1984). The Cortés terrane consists of Paleozoic deep-marine rocks deposited on thinned continental crust (Poole and Madrid, 1988; Coney and Campa, 1984), which was thrust over the Caborca terrane. This tectonic limit was described near of the Cobachi and Mazatán towns located about 40 and 60 km east of the study area, respectively (Ketner and Noll, 1987; Poole et al., 1988). The Paleozoic allochthonous unit was covered in an angular unconformity by the Late Triassic-Lower Jurassic Barranca Group made up of sandstone, shale and conglomerate, with coal beds and tuffaceous layers (Radelli et al., 1987; Stewart and Roldán-Quitana, 1991; Alencaster de Cserna, 1961; Weber et al. , 1980; Weber, 1995). The Barranca Group is interpreted to have been deposited in a rift-type basin, presumably bounded by ~E-W oriented faults (Stewart and Roldán-Quintana, 1991). Intermediate to felsic volcanic flows, tuff, and agglomerate, with minor interbedded continental sedimentary rocks, making up the Tarahumara Formation unconformably overlay the Barranca Group and the Paleozoic sequences. Volcanic rocks yielded ages between 90 and 70 Ma in Central Sonora (McDowell et al., 2001), and of ~79 to 59 Ma in northeastern Sonora (González-León et al., 2011). Large composite batholiths varying from diorite to alkaline granite (e.g., Roldán-Quintana, 1991; Valencia-Moreno et al., 2001) were intruded between 90 and 40 Ma (e.g.Damon et al., 1983; Ramos-Velázquez et al., 2008; González-León et al., 2011). A major pulse of Oligocene-Early Miocene silicic volcanism known as the Upper Volcanic Supergroup followed the Eocene magmatism (McDowell and Keizer, 1977). This pulse consisting of rhyolitic ignimbrites, air-fall tuffs, silicic to intermediate lavas, and lesser mafic lavas, yield K-Ar and Ar-Ar ages between 33 and 28 Ma (e.g.McDowell and Mauger,1994; Albrecht and Goldstein, 2000; Gans, 1997). Basaltic andesites emplaced during and after the ignimbritic episode, known as the Southern Cordillera Basaltic Andesites (SCORBA) yield ages between 33 and 17.6 Ma (Cameron et al., 1989; McDowell et al., 1997; Bartolini et al., 1994; Paz-Moreno et al., 2003), although most ages are Oligocene. Volcanic rocks of this pulse reach up to 1000 m in the Sierra Madre Occidental core but become significantly thinner toward the west (McDowell and Claubaugh, 1981). In the Sierra Santa Úrsula, located 70 km southwest of the study area, a volcanic sequence of ignimbrites, andesites, and dacitic domes yield ages between ca. 23 and 15 Ma (Mora-Álvarez and McDowell, 2000).

Figure 1 Location of the study area in a tectonostratigraphic terrane map. Countries: MEX = Mexico, USA = United States of America; States: BC = Baja California, CHIH = Chihuahua, SIN= Sinaloa; SON= Sonora. Towns: H = Hermosillo, Y = Yécora.
The tectonic regime in Sonora and large part of the Cordillera changed from Late Cretaceous -early Tertiary shortening to middle Tertiary extension (e.g.Calmus et al., 2011), and then, to late Tertiary transtension related to the opening of the Gulf of California. This Tertiary tectonic regime produced major crustal extension, with the formation of low-angle faulting and associated metamorphic core complexes (MCC), as well as areas of more limited extension with high-angle normal faulting forming horst and graben or half-graben systems. The latter style of faulting was responsible of the formation of the Basin and Range physiographic province. Thick clastic sequences intercalated with minor basaltic to rhyolitic rocks filled both the MCC and Basin and Range basins. In the latter type of basins, these sequences are known as the Baucarit Formation (King, 1939; González-León et al., 2011). Volcanic rocks underlying these continental sedimentary sequences have been dated at 27 Ma (Miranda-Gasca and De Jong, 1992; McDowell et al., 1997; Gans, 1997). Volcanic rocks intercalated with clastic sequences yielded ages from ca. 25 to 18 Ma (e.g.McDowell et al., 1997). Extension in the MCC and Basin and Range province of Sonora is constrained mainly between 25 and 15 Ma (Nourse et al., 1994; Gans, 1997; Wong and Gans, 2008). The upper part of the graben-basin successions is less lithified and is intercalated with a distinctive Middle Miocene peralkaline ignimbrite dated at ~12.5 Ma (McDowell et al., 1997; Vidal-Solano et al., 2005, 2007). A post- ~10 Ma faulting phase causing tilting of the peralkaline ignimbrite and related rocks is inferred to accommodate modest amounts (~15%) of ENE-WSW extension in some areas (Gans, 1997).
LOCAL GEOLOGY
The older rocks in the area are informally named as "siliceous siltstone unit", "transitional unit", "quartzite unit", "ribbon chert unit", and "sandy dolomite unit" (Figures 2 and 3), from the structural base to the top. These units are made of thin-bedded black siliceous siltstone, meter-scale intercalations of black siltstone and quartzite, massive quartzite, grey to black ribbon chert, and dolostones, respectively (4a -4c). Bartolini et al. (1995) and Poole et al. (1995) report Middle and Late Ordovician (Caradocian and Asghillian) graptolites from the "transitional unit". A "calk-silicate siltstone unit" occurs in a fault bounded block in the central-western part of the area. White siltstones of this unit contain epidote nodules (Figure 4e) and intercalated quartz-pebble conglomerate. This unit is tentatively correlated with the Barranca Group. A unit made of andesite flows and tuffs unconformably overlies previous units (4d), which is named here "lower volcanic unit" and is lithologically correlated with the Tarahumara Formation. The previous units are intruded by different igneous bodies that caused widespread contact metamorphism. The older intrusions are coarse-grained and granitic in composition, commonly with biotite, but some facies have muscovite. Microgranite porphyry intrudes and includes xenoliths of the coarse-grained granite (4f). This porphyry is made of phenocrysts of plagioclase and quartz surrounded by white to clear-grey microcrystalline matrix. Field relationships and fabric indicate the micorgranite is a hypabyssal intrusion. In turn, the porphyry is intruded by medium-grained diorite to quartz-diorite, composed by amphibole and plagioclase with minor quartz and biotite. The diorite is emplaced below the microgranite along an irregular contact exposed in the Gran Central and La Colorada pits (Figure 4f). The diorite from these pits yielded three consistent 40Ar/39Ar biotite ages of 70 Ma (Zawada et al., 2001) that are interpreted as a cooling age after the diorite intrusion.
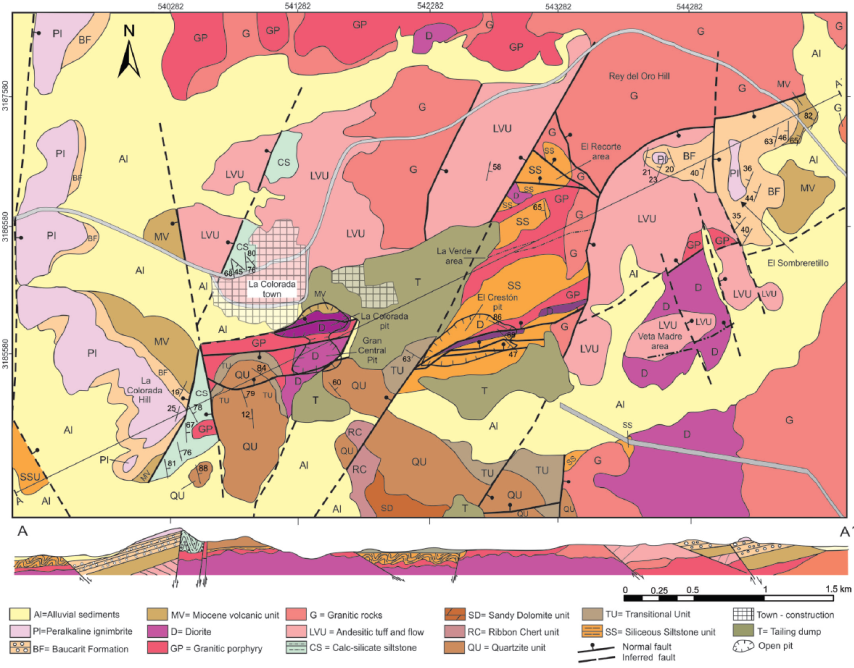
Figure 2 Geologic map of the study area and schematic section. ERF: El Recorte Fault; GCF: Gran Central fault; LCF: La Colorada fault; LAF: Las Amarillas fault; LVF: La Verde fault; NVF: North vein fault; ROF: Rey del Oro fault; SVF: South vein fault.

Figure 3 Stratigraphic column (1) McDowell et al. (1997); (2) McDowell et al. (2001); (3) Zawada et al. (2001); (4) Bartolini et al. (1995); Poole et al. (1988). Spiral indicates fossils.

Figure 4 a) Exposure in the El Crestón pit (view to E); b) Dark siltstones of the transitional unit, Las Amarillas hill; c) Panoramic view of hills made of the Quartzite unit (view to E); d) Exposure of the Lower Volcanic unit; e) Epidote nodules of the calc-silicate siltstone unit; f) Exposure between the Gran Central and El Crestón pits (view to E); g) Panoramic view of the La Colorada hill (view to N); h) Sedimentary breccia of the Baucarit Formation. BF = Baucarit Formation; CSU = Calc-silicate unit; D = Diorite; GP = Granite Porphyry; MVU = Miocene volcanic unit; Pl = Peralkaline Ignimbrite; QU = Quartzite unit; SK = Skarn; SSU = Siliceous Siltstone unit.
Volcanic and sedimentary rocks are exposed inside fault-bounded basins (Figure 4g). The lower part is mainly made by porphyritic andesite flows with hornblende and/or plagioclase phenocrysts, which is here informally named the "Miocene volcanic unit". Hydrothermal alteration is scarce and metamorphism absent in this unit. A correlative amphibole-bearing lava collected 4 km south of the La Colorada town yielded a 24 Ma K-Ar age (McDowell et al., 2001). Based on its lithology and age, this unit may be correlated with the Oligocene-Early Miocene SCORBA event that accompanied and followed the Sierra Madre Occidental ignimbrite flare-up.
A unit made by sedimentary breccias, conglomerate, and minor tuff (Figure 4g and 4h), covers the Miocene volcanic unit. Clasts in breccias mainly derive from the underlying unit. The sedimentary sequence is capped by a ~30 m thick peralkaline rhyolite ignimbrite (Figure 4g; Vidal-Solano et al., 2008), which yielded a 12.3 Ma K-Ar age (McDowell et al., 2001). The ignimbrite is part of a huge pyroclastic eruption that covered part of the Baja California and Sonora states (Vidal-Solano et al., 2005; 2007; 2013; and references therein).
Mineralization
Mineralization in the La Colorada district mainly occurs along veins, splitting veinlets, and tension gashes. Host rocks are Paleozoic metasediments and the Upper Cretaceous plutonic and volcanic rocks. Veins are all fault controlled, with faulting preceding the veining. Veinlets are developed along breccia zones, anastomosing fractures, and tension joints, all of them related with faulting. McMillan et al. (2009) indicate that the veins commonly flared upwards into stockwork zones, and are commonly displaced a few meters by post-ore faults. Three samples from veins in the La Colorada and Gran Central pits yield 40Ar/39Ar sericite ages of 27 Ma, 24 Ma, and 22 Ma (Zawada et al., 2001).
According to Zawada et al. (2001), intrusive rocks exhibit a low degree of competence and host much broader zones of fracture veining and silicification. This results in typically continuous low-grade zones of ore adjacent to high-grade veins. The sedimentary units generally display a higher degree of competency with more restricted quartz-filled fractures in close proximity to the veins. Historically mining was focused on high-grade veins, along which deep shafts and large adits were excavated. Veins are of microcrystalline quartz, although prismatic crystals occur in vein cavities and tension joints. Besides quartz, potassium feldspar and carbonated minerals as calcite, ankerite, and dolomite, occur. The ore consists of gold and silver, which in the El Crestón deposit have an average Au/Ag ratio of 1 to 37 (Zawada et al., 2001). Veins under the oxidation zone include galena, pyrite, chalcopyrite, sphalerite, argentite, molybdenite and perhaps tetrahedrite (Ball, 1911), which are believed to have constituted less than 2% of the vein. Near surface, veins and host rocks are more oxidized displaying a maroon to ochre color. Supergene alteration yields iron and manganese oxides, argentite, malachite, azurite, chrysocolla, cerussite, as well as enrichment of native gold and silver (Zawada et al., 2001). Silicification and potassic alteration consisting of quartz, K-feldspar, and sericite, are found adjacent to veins; while propylitic alteration made of sericite, clay, chlorite, epidote, and carbonate minerals, is found more distally.
METHODOLOGY
The aim of this work is to study the geological structures in the area, in particular those related with the mineralization, as well as the analysis and interpretation of these structures. In order to reach these goals, the strike, dip, and location of each fault was obtained, as well as the pitch of slickenside lineation where present. Shear sense was defined with kinematic indicators such as displacement of markers, tectogliphs, tension veins, and Riedel fractures. In addition to structural data, crosscutting relationships and mineralization-alteration occurrence were described in each fault. Data were plotted and analyzed with the Stereonet 8 software (Allmendinger et al., 2012), while definition of the stress ellipsoid was estimated from the model of faulting of Anderson (1951), and with the Win-Tensor software of Delvaux and Sperner (2003) and the Faultkin software of Allmendinger et al. (2012).
STRUCTURAL GEOLOGY
Folding
Older structures in the area are folds of the Paleozoic metasediments and the "siliceous siltstone unit". Mesoscopic folds are visible locally along the Hermosillo-Yecora highway and roads inside the mine area (Figure 5). Folds can be classified as open to thigh, based on the interlimb angle; some are overturned folds with NE vergence based on the axial plane attitude. The stratification strikes mainly N50-60°W with dips both to NE and SW (Figure 6). Attitude of the Piaxis obtained with the stereonet software is N39°W/28° (trend/ plunge) and the mean axial plane attitude is S37°E 85°SW. Zawada et al. (2001) report inverted strata in the Paleozoic sequence that can be related to large-scale overturned folds in these rocks.

Figure 5 a) Open fold in the Calc-silicate Siltstone unit (view to N); b) Close folds in the Transitional unit (view to NW).
Faulting
The most common structures in the study area are normal faults. A total of 370 faults were measured throughout the area and grouped in six zones in order to perform a detailed structural analysis. These zones are: 1) Gran Central pit; 2) La Colorada pit; 3) El Crestón pit; 4) La Verde area; 5) El Recorte range; and 6) Eastern zone (Figure 2).
Gran Central pit
In this pit, diorite intrudes the microgranite porphyry along an irregular contact (Figure 7a). To the west, the diorite also intrudes quartzite and pelitic hornfels. The main structure in this pit is the Gran Central fault (Figure 8a), which was mined by ~700 m along the strike and ~330 m depth, according to an old unpublished map and section. The fault strikes ~N80°E and dip ~60° N, and the slickenside striations have a pitch of 85°N. The main fault and at least five secondary subparallel faults were target of the open cut mining. West of the Gran Central pit, the main fault juxtaposes a diorite of the footwall against Paleozoic metasediments of the hanging wall. In the eastern side of the pit the fault juxtaposes Paleozoic metasediments of the footwall against andesitic flows of the Miocene volcanic unit in the hanging wall. This fault zone is made of anastomosing fractures filled with cataclasite, Fe-oxides and gouge. Most of the structures in this area are normal faults, with relatively steep dips, although some are clearly listric. The faults displace the contact between the diorite and the microgranite porphyry, which is a clear but non-planar marker. The total displacement of the Gran Central fault cannot be quantified but may be several tens of meters, while in secondary faults the displacement is in the order of meter or less.

Figure 7 Panoramic view of: a) Gran Central pit (view to E); b) La Colorada pit (view to W); c) El Crestón pit (view to ENE). Main faults are highlighted. GP= Microgranite Porphyry, D= Diorite, MV= Miocene Volcanic unit.

Figure 8 Some aspects of faults in the study area. a) Normal fault that juxtaposes the Quartzite unit against the Miocene Volcanic unit (view to E); b) Mineralized vein along a normal fault in the El Crestón pit (view to ENE); c) Crosscuting faults in the La Colorada pit, a mineralized NE striking fault (F1) is displaced by a non-mineralized-brecciated NW striking fault (F2) (view to N); d) Slickenside lineations with oblique displacement along a normal fault near to the Gran Central pit; e) Mineralized tension gashes between two low-angle normal faults, Gran Central pit (view to W); f) Riedel fractures (R) in a normal fault zone (view to W).
La Colorada pit
Along the pit wall, diorite intrudes the microgranite porphyry. La Colorada fault is the main structure in this pit, which strikes N75°E and dips ~50° NW (Figure 7b). The main fault zone includes about 20 m of anastomosing fractures with fault breccia, cataclasite, and gouge, as well as thin quartz veinlets and extensive oxidation. In the eastern side, the fault places andesitic rocks of the Miocene volcanic unit in the hanging wall against diorite and microdiorite in the foot-wall. Old underground adits followed this structure for ~580 m along strike, although the present pit has only 370 m of length. Drill holes by Eldorado Exploration found the mineralization about 200 m down-dip under the actual pit bottom (McMillan et al., 2009).
El Crestón pit
Geology in this pit is complex including different lithologies of the Paleozoic metasediments that are intruded by dikes and apophyses of coarse-grained granitic rocks, which are displaced by a set of normal faults (Figure 7c and 8b). Contact metamorphism-metasomatism transformed the sedimentary rocks into fine-grained hornfels and local garnet -epidote skarn. Main veins strike ~N70°E with dips between 70° to 50° N. Old mining adits extended ~580 m along the strike and the shafts reach about 260 m depth. According to Ball (1911) the veins converged upwards creating a wide zone of stockwork, which allowed mining by "open cut methods". The present open pit has a surface length of about 800 m along the vein strike, is 366 m wide, and ~100 m deep. At least, five large veins can be seen in the northeastern wall, each one hosting subparallel quartz veinlets with oxidation (Figure 8b).
La Verde Mine zone
According to Ball (1911) the Verde Mine was the first gold mine in the La Colorada District. Anastomosing quartz veins with pyrite and copper carbonates outcrop along 2 to 8 m width and ~100 m length. Old adits in this zone extended about 230 m along the strike. The main mineralized structure is the La Verde vein, with an average width of 2.4 m. This vein strikes N75°E and dips 70° N. Host rocks are micro-granite porphyry, granite and siliceous siltstones.
El Recorte range
This range is a fault bounded horst mainly consisting of coarse-grained granitic rocks. To the north, a NE striking normal fault juxtaposes the granite and Paleozoic metasedimentary rocks against the Upper Cretaceous (?) "Lower volcanic unit". To the south, an ENE striking fault juxtaposes the granite against Upper Cretaceous (?) volcanics, as well as with the Baucarit Formation and its cap made of the peralkaline ignimbrite. Numerous quartz veinlets, subparallel to the south boundary fault, occur in the south slope but have not been mined.
Eastern zone
This zone is located south of the El Recorte range and east of the normal fault that juxtaposes Paleozoic metasediments in the east against the Lower Volcanic unit in the west. The Upper Cretaceous dioritic intrusive is also exposed in this block. Tertiary volcanic and sedimentary rocks cover the Lower Volcanic unit.
Structural analysis
Structural data of faults in the six studied zones are displayed as pole to fault stereogram, statistical contours, and rose diagrams in Figure 9. In order to separate different populations of faults, distribution of the fault azimuths is displayed as a frequency histogram in Figure 10, which also includes a Kernel density estimation (Zucchini, 2003), both using the Excel program. In the Kernel plot, troughs occur in ~N51°E, N54°W, N25°W, and N15°E (Figure 10), separating four fault sets, each one consisting of two families of conjugate faults.

Figure 9 Lower hemisphere, equal area projections of poles to fault planes with statistical contours and rose diagram of the fault azimuths (right-handed rule). a-b) Gran Central pit and nearby areas; c-d) El Crestón pit and nearby areas; e-f) La Colorada pit; g-h) La Verde area; i-j) El Recorte area; r-l) Eastern Zone.
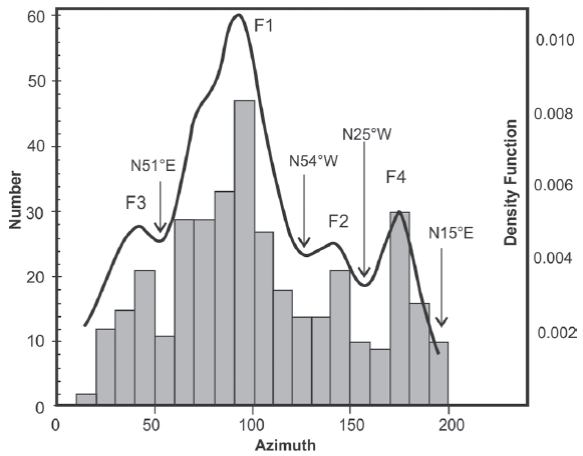
Figure 10 Frequency histogram of the fault plane azimuths, the azimuth range was selected to encompass all sets in the area (F1 to F4). The black line is a Kernel density estimation function with an H parameter of 8 (Zucchini, 2003); troughs indicate the limit between different populations. Number of faults = 369.
The main set in all zones strikes between N51°-90°E and N54°-90°W with peak at ~N88°E (Figure 9, 10, and 11A, Table A of the electronic appendix). Strikes between N51°-90°E predominate in the Gran Central and El Crestón areas; while strikes between N54°-90°W predominate in the La Colorada, La Verde, and El Recorte areas (Figure 9). Faults of this group are the main host of the mineralization. This group is represented for 196 faults, 40 of which dip to the south (SE-SW) and the rest to the north (NE-NW), forming a conjugate fault system (Figure 11a). Distribution of fault poles along the N-S axis indicates the listric character of faults in this set, which was also observed in exposures. The second fault set strikes between N25°-54°W with an average of N40°W. This group is represented by 67 faults 15 dipping to the SW and the rest to the NE yielding a conjugate fault system (Figures 9, 10, and 11b). The third group of faults strikes between N15°-51°E with an average of N34°E (Figures 9, 10, and 11c). This group includes 49 faults, 26 dipping to the SE and the rest to the NW yielding a conjugate fault system. The fourth fault set strikes between N25°W and N15°E with a mean at N3°W (Figures 9, 10 and 11d). This group encompasses 52 faults, 22 dipping to the E and the rest to the W, yielding a conjugate fault system.

Figure 11 a to b: Statistical 1% area contours of the poles to fault planes of the four sets, lower hemisphere, equal-area projection. Segmented line indicates the average strike of each group. Below each stereogram the rose diagram displays the cumulate number of faults by azimuth; arrow indicates the average strike of each set of faults.
The inferred relative age of the different fault sets is as follow: the ~E-W fault set is the oldest in the area, followed by the NW-SE fault set, then the NE-SW set, and finally the ~N-S set. The criteria used for the definition of the relative age are: a) direct observation of crosscut-ting relationships between faults of different sets (e.g.Figure 8c, Table A electronic appendix), which is also noted in the geologic map; for example, the NE-SW fault located east of the El Crestón pit and the La Verde area (Figure 2) crosscut all the mineralized ENE-WSW fault veins. Besides, neither the Miocene volcanic or sedimentary units are cut by this set or contain mineralized ores; b) the main mineralized event postdate the ENE-WSW fault set conforming the main ore bodies in the area, and predate the NW-SE, NE-SW and N-S sets; c) Miocene clastic sediments are mainly deposited along NW-SE fault basins as occurred with the Baucarit Formation in central Sonora (e.g.Bartolini et al., 1994; McDowell et al., 1997; Gans, 1997); d) a NE-SW normal fault limits and predates the Middle Miocene (12.3 Ma) peralkaline ignimbrite in the La Colorada hill (Figure 2); e) the ~N-S set tilt the peralkaline ignimbrite being the younger in the study area.
Dynamic analysis
Dynamic analysis seeks to define the stress field in a region following the model of faulting by Anderson (1951), which is based on the assumption that in the shallow crust one principal stress is always vertical. For normal faults the main stress σ1 is vertical while σ2 and σ3 stresses are horizontal. Theoretically, a population of normal faults occurs as two families, each one dips steeply in a direction opposite to those in the other family, with σ1 bisecting the acute angle between faults, σ2 located parallel to the intersection line of the two faults, and σ3 bisecting the obtuse angle between faults (see Angelier, 1994; Rowland et al., 2007; Célérier et al., 2012). In the study area, the first fault set (F1) has a mean strike value of N88°E (Figure 11a, Table A), then the extension direction must be ~N2°W. The second fault set (F2) has an average strike of N40°W (Figure 11b), and then extension direction must be N50°E. The third fault set (F3) has a mean strike of N34°E (Figure 11c), and then extension direction must be ~N56°W. The fourth fault set (F4) has an average strike of N3°W (Figure 11d), and the extension direction must be N87°E. In the Anderson´s model, if slickenside lineations are developed on a conjugate normal fault system, they must be mostly dip-directed. However, if a subsequent stress field occurs in a region, the old faults are often reactivated typically with an oblique-slip movement (Rowland et al., 2007), because strain compatibility may control the slip directions of different fault sets during the later phases of deformation (Marrett and Allmendinger, 1990). Dynamic analysis does not account for possible tilting of former faults caused by latter faulting phases, which can variably modify the attitude of faults and then, the original extension directions. In order to obtain the original extension directions, tilting caused by each subsequent fault phase must be eliminated in each fault, which is impractical or unworkable.
Kinematic analysis
The model of faulting by Anderson (1951)assumes that the stress field causing deformation in the shallow crust is uniform over a large area, and then, that multiple fault types require multiple deformation episodes. However, nonuniform stress fields may occur during a single tectonic episode originating different types of faults with diverse orientations simultaneously (e.g.Molnar and Tapponnier, 1975; Reches, 1978; Marret and Allmendinger, 1990; Nieto-Samaniego and Alaniz-Alvarez, 1997). Kinematic analysis is a technique for analyzing fault data (e.g.Marrett and Allmendinger, 1990), which permits define the overall strain pattern in an area as well as testing for kinematic compatibility of faults sets. This analysis can only be performed in faults with slickenside lineation and definite shear sense; in the study area, 26 faults fulfill these requirements (Figure 12, Table 1). With regard to the number of faults, Arlegui-Crespo and Simón-Gómez (1998) argue that some methods produce very stable standard solutions from fault samples made up of about 25-30 faults. Inversion of fault data of the study area was done with the FaultKin software by Allmendinger et al. (2012) and the Win-Tensor software by Delvaux and Sperner (2003). For analysis, fault data were separate in the four sets defined above, considering that, as indicated by Célérier et al. (2012), establishing the chronological order of faulting is the first and most important step in dealing with polyphase data, which can efficiently guide the sorting process. Faults, slickenlines, and movement directions are displayed in Figure 12a.

Figure 12 Results of the inversion of fault data with slickenside lineations with the Faultkin software (Marrett and Allmendinger, 1990; Allmendinger et al., 2012). a) Faults with striae and slip direction; b) Shortening and extension axes; c) Kamb contours of extensional (T) axes; d) Kamb contours of the shortening (P) axes; e to h) Kinematic axes for the different fault sets calculated with the Linked Bingham distribution statistics Faultkin software (Allmendinger et al., 2012); i to l) Kinematic axes obtained from the inversion of fault data with the Win-Tensor software (Delvaux and Sperner, 2003). In figures e to l circle indicates the shortening axes (P); square indicates the extension axes (T), and the triangle indicates the intermediate axes (B).
Plot of the extension (T), shortening (P), and intermediate (B) axes of the four fault sets is displayed in Figure 12b. Extensional axes contouring with Kamb method yield two clear groups one WNW-ESE and other NNE-SSE (Figure 12c). Most of the shortening axes make a wide E-W girdle (Figure 12d). Two fault sets are compatible if the respective extension and shortening axes coincide. In this sense, faults of the first set are kinematically incompatible with faults of the other three sets, while faults of the fourth set are compatible with faults of the third set, and partially compatible with faults of the second set.
Marret and Allmendinger (1990) indicate that kinematically heterogeneous faulting, represented by girdle or multi-modal patterns of shortening and/or extension axes, can be produced by triaxial deformation, anisotropy reactivation, strain compatibility or multi-phase deformations. A criterion supporting multiple deformations is that a single fault set displays widely varying slip directions, as in the case of F1 in the area (Figure 12a). Slip direction of F1 has highly variable slip directions, while slip directions of F4 are mostly coherent; indicating that the F1 has been reactivated whereas F4 has not been. Additional evidence for multiple deformations in the area is systematic cross-cutting relationships between fault sets (Table A) and mutually exclusive chronologic constraints on the fault sets, which have been explained above. However, kinematic compatibility exists mainly between the third and fourth sets (Figure 12b) suggesting that they can be generated by a similar stress field.
DISCUSSION AND TECTONIC MODEL
By the end of the Mesozoic Era, the Laramide orogeny folded, faulted, and locally foliated Upper Cretaceous sedimentary and volcanic sequences (González-León et al., 1992; García-Barragán and Jaques-Ayala, 2011) . Folds related with this phase have NNW-SSE axis and NE vergence (González-León et al., 1992), similar to those in the study area, which fold the "calk-silicate siltstone unit".
The lower volcanic unit, granite and diorite intrusions, and the Miocene volcanic unit are all related to subduction of the Farallon plate along the western margin of the North American plate (e.g.Damon et al., 1983; Roldán-Quintana, 1991; Valencia-Moreno et al., 2006). Continued subduction resulted in the oblique interaction of the East Pacific Rise (EPR) with the North American plate. Transform faults fragmented the EPR causing diachronic arrival of the ridge to the trench. According to Atwater and Stock (1998) and Wilson et al. (2005) the EPR arrived at the trench offshore southwestern California by 28.5 Ma. This arrival coincides with ages of extension in Arizona and Sonora (e.g.Cameron et al., 1989; Armstrong, 1990; Dickinson, 1990; Miranda-Gasca and De Jong, 1992; Gans, 1997; Wong and Gans, 2008). The first extensional phase produced normal faults striking ~E-W. Some of these faults display dip-parallel slickenside lineations (Figure 13a). Dynamic and kinematic analysis indicates that F1 was caused by a ~vertical σ1, and a σ3 striking ~N-S (Figures 11a, 12e and 12i). Similar direction of extension has not been mentioned, although Gans (1997) mention ENE-WSW strike-slip faults in the Santa Rosa region that he regards as accommodation faults related to NW-SE normal faults, which were active between 26 and 20 Ma. Similar E-W mineralized structures occur in the San Dimas District (Tayoltita, Durango), where the veins are hosted in rocks dated between 40 and 36 Ma, and precede rocks dated between 25-20 Ma (Horner and Enríquez, 1999). These authors regard the veins as tension gashes associated with an E-W striking σ1 and ~N-S striking σ3, derived from the latest compression stages of the Laramide Orogeny. Ferrari et al. (2007) suggest that the San Dimas veins and other mineralized veins (e.g.Staude and Barton, 2001) could be generated during a deformation phase occurring between the Laramide Orogeny and the Oligocene-Miocene extension, which these authors name "pre-Oligocene deformation". In Nayarit, similarly oriented structures are considered as predating a 23 Ma dike (Duque-Trujillo et al., 2014). Considering the Late Oligocene-Early Miocene (27-22 Ma) ages obtained in sericite of the La Colorada mineralized veins (Zawada et al., 2001), the F1 set may be accommodation zones as those of the Santa Rosa region, or reactivation of older (Late Triassic?) structures as those proposed by Stewart and Roldán-Quintana (1991). Alternatively, the F1 set could be generated by intrusion of a laccolithic diorite body, which would produce a local strain. However, faults in the mine area postdate the diorite, and faulting related to shallow intrusions commonly displays a concentric or radial pattern (e.g.Cole et al., 2005; Acocella et al., 2000), which is not observed in the study area.

Figure 13 Interpretative model for the evolution of faulting in the study area (drawings are schematic).
The second extensional phase produced NW-SE striking faults (F2) similar to those of the Basin and Range Province of Sonora (Figures 11b and 13e). Kinematic analysis indicates the F2 faults were originated by NE60-80°SW directed extension (Figure 12f). The same direction of extension characterizes the MCCs of Sonora and Arizona (e.g.Dickinson, 1991; Nourse et al., 1994; Gans, 1997; McDowell et al., 1997; Vega-Granillo and Calmus, 2003). The F2 faults may reactivate the F1 faults, generating oblique slickenside lineations on the later (Figure 13a, 13b). This extensional event has been related to gravitational instability caused by thickened crust combined with a high geothermal gradient related with the Eocene-Oligocene magmatism (e.g.Liu and Shen, 1998; Calmus et al., 2011). The age of the main extensional phase in the Mazatán MCC is constrained between 25 and 16 Ma (Wong and Gans, 2008); while in the Santa Rosa region, is constrained to between ~26 and 20 Ma (Gans, 1997) . In both, the MCC and the Basin and Range basins, volcanic rocks dated at ~27 Ma (Miranda-Gasca and De Jong, 1992; McDowell et al., 1997; Gans, 1997) underlies the clastic rocks, which support a Late Oligocene inception of this extensional phase. Ferrari et al. (2013) and Murray et al. (2013) found a similar extensional phase in Sinaloa-Nayarit and in the border of Chihuahua-Sonora, respectively. Andesitic volcanism of 24 Ma (McDowell et al., 2001) is coeval to the second extensional phase and may be related to the thermal source that produced the main hydrothermal event in the area, which flowed along the F1 faults generating the most important mineralized veins.
The third extensional phase originated faults striking N15°-51°E (Figure 11c). Pole to fault clusters (Figure 11) indicate that NW dipping faults are steep, while SE dipping faults have low -angle dips, which can be caused by rotation of this family during the fourth extensional phase. No dip-parallel slickenside lineations were observed in this set, maybe due to the nature of the faults, which are formed by anostomosing, brecciated, cataclastic zones, and gouge. However, faults of the F1 and F2 sets must be reactivated during this phase generating NE and SW trending lineations (Figure 12a, Figure 13c and 13f). According to the kinematic analysis, this phase may be originated by NW-SE shortening direction (Figure 12g) or by NW-SE extension direction (Figure 12k). These apparently contradictory results can be explained by the activation of a transtensional regime with σ1 ~N25°W similar to the present main stress in the San Andreas-Gulf of California system, which also produces local field stress with NW-SE extension in relief zones. As discussed above the NE-SW faulting must be slightly older
than, or coeval with the emplacement of peralkaline ignimbrite at 12.3 Ma. Faults striking ~N35°E have been scarcely described in Sonora, although a similarly orientated system is the Empalme graben (Roldán-Quintana et al., 2004). Based on chemical correlations and geological relationships of the peralkaline ignimbrite, Vidal-Solano et al. (2013) propose that dextral faults between Bahia Kino and the Sierra Libre displaced crustal blocks to the NW during a period of strong tectonic activity related to a proto-Gulf transtensional episode. Then, the stress responsible for the displacement of the Gulf of California, can origi-nally be active over a large area of Sonora and Baja California, creating the Gulf Extensional Province of Karig and Jensky (1972), which is considered to have been active sometime between ~12 and 6 Ma (e.g.Henry and Aranda-Gómez, 2000; Bennett et al., 2013; Bennett and Oskin, 2014). A stress field with a ~N25°W oriented σ1 and ~N65°E oriented σ3, may have produced dextral strike-slip faults striking ~N55°W, parallel to the present Gulf Fault System, as well as normal faults striking ~N35°E in relief zones, as those in the pull-apart basins of the Gulf of California.
The fourth extensional phase originated conjugate normal fault system (F4) striking ~N- S, with dip- parallel slickenside lineations (Figure 12a, Figure 13j). This phase reactivate previous fault sets producing ~E-W trending slickenside lineations (Figure 12a; Figure 13d, 13g, 13i). Kinematic analysis indicates this phase was produced by ~E-W extension with ~vertical shortening direction (Figure 12h, 12l). Faults with similar orientation displacing NW-SE oriented faults have been described in many localities of Sonora (e.g.Gans, 1997). In the MCC of Sonora, late N-S faults create basins along which clastic sediments overlie older Tertiary strata in angular unconformity (e.g.Miranda-Gasca and De Jong, 1992; Vega-Granillo and Calmus, 2003).
We proposed that the NNW-SSE faults in the coastal and inner region of Sonora may have been caused by a permutation of σ2 by σ1, which occurred ~6 Ma ago once the transtensional regime was released from the continent and concentrated in the Gulf of California fault system (Bennett et al., 2013; Bennett and Oskin, 2014).
CONCLUSION
Detailed structural study in the La Colorada mine area indicates a complex tectonic history since the Late Cretaceous. Rocks intruded by a Late Cretaceous (~70 Ma) diorite body are affected by a folding phase related to the Laramide Orogeny. Four phases of normal faulting were recognized and its relative ages defined based on crosscutting relationships, rock ages, field observations, and structural analyses. Faults of the first phase strike ENE-WSW to E-W, dip to N or S, and host the main mineralized veins in the area. This phase must have occurred prior to the age of the hydrothermal event at 27-22 Ma. The inception of this phase as well as its regional tectonic significance remains to be precisely defined. The second extensional phase produced faults with NW-SE strikes and SW to NE dips, reactivating the F1 faults. We associate this phase with the formation of the Basin and Range province and the MCCs in Sonora and Arizona, between ~ 26 and 15 Ma ago (Dickinson, 1991; Nourse et al., 1994; Gans, 1997; Vega-Granillo and Calmus, 2003; Wong and Gans, 2008). The inception of this phase coincides with the arrival of segments of the East Pacific Rise to the trench. The third extensional phase produces faults with NE-SW strike and NW or SE dips, and reactivated the F1 and F2 sets. The stress field responsible for the normal faults must have a ~vertical σ1 and σ3 ~N35°W. Based on available data, we interpret that between 12.5 and 6 Ma a transtensional regime was imposed in a region including part of Sonora and Baja California, producing the Gulf Extensional Province. That regime was caused by a field stress with σ1 ~horizontal and oriented ~N25°W and with σ2 vertical. We infer that the NW-SE trending extension of this phase can be related with step-over between the NW- SE strike-slip faults. About 6 Ma ago, concentration of right-lateral movement in the present Gulf of California fault system may have released the ~N25°W horizontal σ1 stress in the continent, causing a change in the field stress, which may have originated the fourth extensional phase in the area.