Introduction
The growing consumer interest in “healthy living” has boosted the market for more natural and functional food claims; additionally, producing foods that contain a high content of phytochemicals and high protein quality that are beneficial to health and have a relatively low cost has been considered (Medina et al., 2020). The population of Americans suffering from celiac, gluten intolerance, and wheat allergy is 1 in every 14 people. Also, many choose gluten-free (GF) diets nowadays because of the perception that it is a healthier option for them. Therefore, in the last decade, the GF market in the US and all over the world has seen a significant growth. Globally, GF product sales reached 4.63 billion USD in 2017 and are expected to reach 6.47 billion USD by 2023, a projected compound annual growth rate of 7.6% (Woomer & Adedeji, 2021). Celiac disease (CD), or gluten-sensitive enteropathy, is a generalized autoimmune disease characterized by chronic inflammation and atrophy of the small intestine’s mucosa by exposure to dietary gluten that affects genetically predisposed individuals (Kamel et al., 2020; Lebwohl & Rubio-Tapia, 2021; Wieser et al., 2021). Gluten refers to a family of proteins known as prolamins (primarily glutenin and gliadin) that constitute the storage protein in the starchy endosperm of many cereal grains such as wheat, barley, and rye (Niland & Cash, 2018). The treatment for CD is a strict gluten-free (GF) diet for life, excluding dietary wheat, rye, barley, and hybrids like Kamut and triticale. A strict GF diet improves histological lesions, blood biochemistry, clinical manifestations, and CD-related complications (Sharma et al., 2020).
GF food products existing in the market today are more expensive than gluten-containing food products. Also, these are known to have lower nutritional value than the critical needs of celiac patients. It is necessary to develop cheap, nutritionally rich gluten-free food products to promote healthy living in people who have the CD (Gómez-Favela et al., 2021). Some of the GF and nutritious grains include cereals (corn, rice), pseudo-cereals (buckwheat, amaranth, chia, quinoa), millets (pearl millet, finger millet, Kodo millet, foxtail millet, pearl millet), and pulses (soybean, common bean, pea, chickpea, Bengal gram), which can be used for the preparation of gluten-free functional foods (Gómez-Favela et al., 2021; Kumar, 2018).
Quinoa (Chenopodium quinoa Willd.) is a crop native to the Andean region. It is an annual dicotyledonous herb belonging to the genus Chenopodium, which has been cultivated for 5000-7000 years (Tan et al., 2020). Due to its increasing popularity, the grain has been recently introduced as a cereal crop in non-indigenous regions such as Europe, North America, Australia, China, and Japan. Quinoa is a member of the family Amaranthaceae rather than Poaceae (Gramineae), but because the seeds are mostly milled into flour and used as a cereal crop, it is referred to as a pseudo-cereal (Li et al., 2021). Quinoa seeds reveal high levels of essential fatty acids, vitamins, minerals, dietary fibers (8%-13%), and proteins (12%-23%) with a balanced amino acid spectrum, with high contents of lysine and methionine (Angeli et al., 2020; Aziz et al., 2018; Montemurro et al., 2019; Pellegrini et al., 2018). In addition to this rich nutritional composition, these seeds have been found to contain a large variety of bioactive compounds (phenolic compounds, phytosterols, squalene, phytoecdysteroids, carotenoids), which have proved protective against a variety of diseases, particularly cancer and inflammatory diseases, and they may reduce the risk of cardiovascular diseases, considering quinoa seeds a functional food (Montemurro et al., 2019; Tang & Tsao, 2017). Moreover, quinoa is considered gluten-free and suitable for celiac patients and people who have a wheat allergy (Caratini & Rosentrater, 2019; Demir & Bilgicli, 2021).
Chia (Salvia hispanica L.) seeds were used together with bean, corn, and amaranth by Precolumbian Mesoamerican cultures (Mayas and Aztecs) to prepare foods and medicines. Chia was the second main crop after beans. In the Aztecs communities, chia was used for food, cosmetics, and religious rituals (Hrncic et al., 2020). In Mexico, chia seeds are currently used for their nutritional and medicinal properties, i.e., endurance for athletes, appetite suppressor, blood glucose control, weight loss agent, and intestinal regulation (Martínez-Cruz & Paredes-López, 2014). Chia seed has approximately 15%-24% protein, 26%-41% carbohydrates, and 25%-40% fat. It has been studied principally due to its oil quality content, possessing almost 55%-60% linolenic acid (ω-3), 18%-20% linoleic acid (ω-6), 6% monounsaturated fat (ω-9), and 10% saturated fat. On the other hand, the seed has highly soluble and insoluble dietary fiber, over 35% of the total weight, and a rich source of the B vitamins and minerals. It also contains six more-fold calcium, 11 more-fold phosphorus, and four more-fold potassium than 100 g of milk; besides this, it possesses magnesium, iron, zinc, and copper (León-López et al., 2019; Masood, 2022; Orona-Tamayo et al., 2017). On top of that, it has many natural antioxidants, such as phenolic compounds (chlorogenic and caffeic acids, and flavonols [quercetin, kaempferol, myricetin]), which protect against some adverse conditions (Hrncic et al., 2020). Another important characteristic of this seed is that it does not contain gluten. Persons with celiac disease can consume it, thus, making seeds a safe ingredient for gluten-free diets (Menga et al., 2017). Recently, Rubavathi et al. (2020) reported that chia seeds extracts possess anti-obesity activity.
Extrusion process is an adequate technology to generate functional foods. This process increases the digestibility and biological value of proteins and maintains or increases the content of bioactive compounds and nutraceutical properties of foods, due to the use of high-temperature, high-pressure, and high shear conditions in a short period. These accelerate process conditions, used during the extrusion, cause important molecular transformation and chemical reactions (modification in starch, proteins, dietary fiber and lipids, inactivation of enzymes and microorganisms, and formation of color polymers and volatile flavor components) on the food materials. In addition, this process is a highly efficient alternative technology that minimizes energy and water consumption, and does not produce polluting effluents (León-López et al., 2019; Ortiz-Cruz et al., 2020; Ramos-Enríquez et al., 2018; Reyes-Moreno et al., 2018).
In the functional food market, the functional drinks market is one of the fastest-growing segments (Tolun & Altintas, 2019). Consumer demand for “healthy” foods and beverages is seen as a driving force behind the functional foods sector (Kim & Adhikari, 2020). An expert report on science from the Institute of Food Technologists (IFT) defined functional food and food components as products that provide a health benefit beyond basic nutrition (for the intended population). The functional drinks are considered as a most active and effective sector because of (1) the possibility to cover consumer demands for appearance, container contents, shape, and size; (2) the ease of distribution and better storage for low temperatures and shelf-stable products; and (3) the opportunity to incorporate bioactive compounds (peptides, antioxidants, omega three fatty acids, dietary fiber, prebiotics) and nutrients (good quality protein, with a good balance of EAA, lipids -essential fatty acids-, vitamins, minerals) (Argüelles-López et al., 2018).
In Mexico, 76.6% of adults, 36.3% of adolescents, and 33.2% of children suffer from overweight and obesity, and 25.5% of adults suffer from arterial hypertension, as reported by Encuesta Nacional de Salud y Nutrición de Medio Camino (ENSANUTMC) (Instituto Nacional de Salud Pública [INSP], 2016). In the initiation and progression of hypertension, oxidative stress is a significant factor. An essential factor in blood pressure regulation is the angiotensin-converting enzyme (ACE). ACE inactivates Bradykinin (vasodilator) and catalyzes the conversion of angiotensin I into angiotensin II (vasoconstrictor). One of the strategies for combating hypertension is searching for ACE inhibitors from natural products such as peptides, triterpenes, and phenolic compounds (Argüelles-López et al., 2018). Some researchers (Argüelles-López et al., 2018; Masood, 2022; Montoya-Rodríguez et al., 2015; Pellegrini, et al., 2018) have reported that phenolic compounds derived from pseudo-cereals (quinoa and amaranth) and chia have antioxidant and antihypertensive effects.
In this context, public policies must be implemented to reverse the obesogenic environment, characterized by increased access to high-calorie food and beverages, a sedentary lifestyle, and constant promotion of non-healthy product consumption.
In this sense, the objectives of this work were: (1) to optimize the extrusion process of whole quinoa seeds to find the best combination extrusion temperature (ET)/screw speed (SS) for producing optimized extruded quinoa (OEQ) flour with high antioxidant activity (AoxA), total phenolic content (TPC), and in vitro protein digestibility (IVPD); and (2) to develop a functional gluten-free beverage (GFB) high nutritional/nutraceutical value from a mixture of optimized extruded quinoa and extruded defatted chia (OEQ, EDC) flours.
Materials and Methods
Materials
Commercial varieties of quinoa (Chenopodium quinoa Willd) and chia (Salvia hispanica L.) seeds were purchased in a local market (Mercado Rafael Buelna, Culiacán, Sinaloa, México). Seeds were cleaned and kept in tightly sealed containers at 4 °C until used.
Production of extruded quinoa (EQ) flours
The EQ flours were prepared as suggested by León-López et al. (2019). Response surface methodology (RSM) was applied to find the best combination of process variables (Extrusion temperature [ET = 60 °C-140 °C]/screw speed [SS = 60 rpm-240 rpm]) for producing optimized extruded quinoa (OEQ) flour with maximum values of AoxA, TPC, and IVPD. The central composite experimental design generated 13 treatments (Table 1). Portions of 250 g of whole quinoa seeds were mixed with lime (0.21%) and water (28% humidity), and they were allowed to rest (4 °C-8 °C/12 h) and tempered (25 °C/1 h). After tempered, the portions of quinoa flours were fed to the extruder and underwent various extrusion temperature/screw speed (Table 1). The extrudates coming from each of the 13 treatments were cooled, ground, packed in plastic bags, and stored (4 °C). AAox, TPC, and DPIV were evaluated to the 13 different EQ flours.
Table 1 Experimental design1 used to obtain different combinations of extrusion temperature/screw speed for producing extruded quinoa (EQ) flours, and experimental results for response variables.
Assay | Process variables | Response variables | |||
Extrusion temperature (ºC) | Screw speed (rpm) | AoxA2 | TPC3 | IVPD4 | |
1 | 71.72 | 86.36 | 14 150 | 485.4 | 80.5 |
2 | 128.28 | 86.36 | 15 730 | 609.9 | 86.3 |
3 | 71.72 | 213.64 | 14 891 | 503.7 | 79.7 |
4 | 128.28 | 213.64 | 16 470 | 640.0 | 87.2 |
5 | 60.00 | 150.00 | 13 230 | 475.0 | 78.2 |
6 | 140.00 | 150.00 | 16 224 | 620.6 | 88.6 |
7 | 100.00 | 60.00 | 15 460 | 580.3 | 83.3 |
8 | 100.00 | 240.00 | 16 120 | 608.2 | 80.3 |
9 | 100.00 | 150.00 | 15 318 | 539.7 | 86.6 |
10 | 100.00 | 150.00 | 15 386 | 550.1 | 84.6 |
11 | 100.00 | 150.00 | 15 251 | 543.3 | 85.3 |
12 | 100.00 | 150.00 | 15 072 | 530.2 | 86.4 |
13 | 100.00 | 150.00 | 14 956 | 538.4 | 85.8 |
1 Central composite experimental design with two factor and five variation levels (13 assays); 2 AoxA = Antioxidant activity (µmol Trolox equivalents [TE]/100 g, DW); 3 TPC = Total phenolic content (mg Galic acid equivalents [GAE]/100 g, DW); 4 IVPD = in vitro protein digestibility (%).
Source: Author's own elaboration.
Optimization of the extrusion process to obtain optimized extruded quinoa (OEQ) flour
Predictive models of second order were generated for each response variable from the data experimentally obtained from the extrusion process for whole quinoa seeds (Table 1). The models were adjusted, and, subsequently, the non-significant terms (p > 0.1) were eliminated from the second-order polynomials, and new prediction models were calculated for each response variable (Yolmeh & Jafari, 2017). The program Design-Expert version 7.00 was used to carry out these analyses.
The RSM’s conventional graphical method was applied to obtain maximum values (optimization) of AoxA, TPC, and IVPD in optimized extruded quinoa (OEQ) flour. The prediction models were used to graphically represent the system (Figure 1a, 1b, 1c). The overlay of each one of the response variables contour plots allowed to obtain a unique contour plot (Figure 1d) for observation and selection of the best (optimum) combination ET/SS for producing OEQ flour (Figure 1d) with high AoxA, TPC, and IVPD. Once the optimized extrusion conditions were obtained, OEQ flour was prepared; it was packed in plastic bags and stored at 4 °C. After that, OEQ flour was analyzed for chemical composition, nutritional and nutraceutical properties.
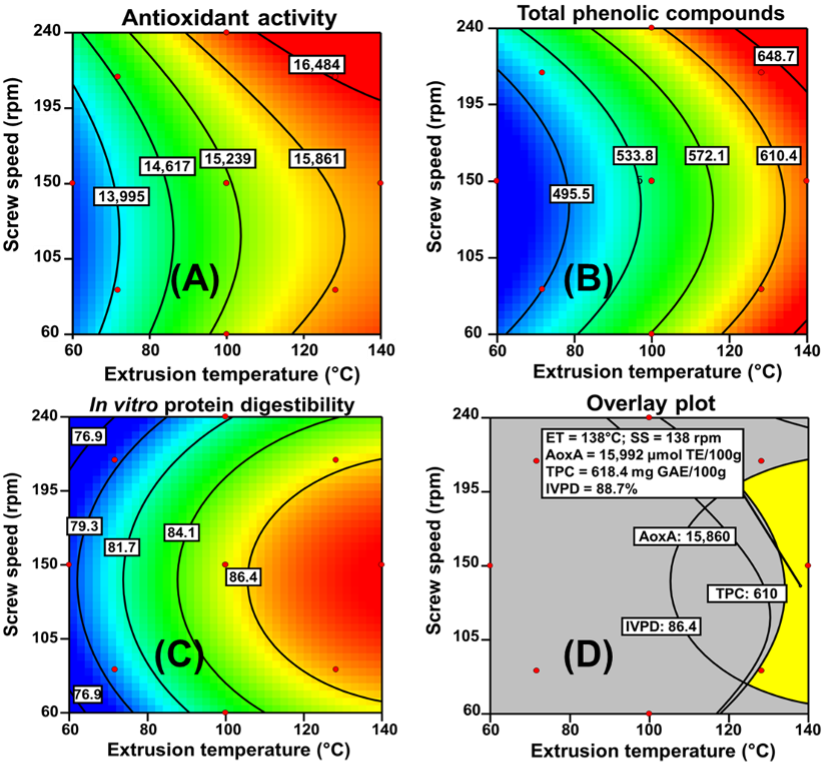
Source: Author's own elaboration.
Figure 1 Contour plots showing the effect of extrusion temperature (ET) and screw speed (SS) on (A) Antioxidant activity, (B) Total phenolic content, and (C) in vitro protein digestibility. (D) Region of the best combination of extrusion process variables for producing optimized extruded whole quinoa flour (OEQ)
Antioxidant activity and total phenolic content (AoxA, TPC)
The ORAC assay carried out by dilution of free and bound phenolic extracts were diluted in 75 mM phosphate buffer (pH 7.4). Aliquots of 25 μL of diluted extracts were mixed with 150 ml of fluorescein (0.1 mM) and 25 μL of the peroxyl radical AAPH (200 mM). After 30 minutes, fluorescence (485 nm for excitation and 538 nm for emission) was measured (37 °C) at 2 min intervals for 60 min, using a Synergy Microplate Reader (SynergyTM HT Multi-Detection, BioTek, Inc., Winooski, VT) (León-López et al., 2019). The results ORAC assay were expressed as micromol of Trolox equivalent (TE) per 100 g of dry weight sample. The TPC of free and bound extracts were determined using 20 ml of appropriate dilutions of extracts, and they oxidized with 180 ml of Folin-Ciocalteu reagent (Gámez-Valdez et al., 2021). The resulting blue color’s absorbance was measured after 20 min, at 750 nm, using the Synergy Microplate Reader and expressed the TPC in mg gallic acid equivalents (GAE) / 100 g DW. All measurements were made in triplicate.
In vitro protein digestibility (IVPD)
The IVPD was evaluated according to Salas-López et al. (2018), using a modified multienzyme system. Fifty milliliters of a multienzyme solution were prepared (80 mg of pancreatic trypsin [15 600 U/mg], 155 mg of bovine pancreatic chymotrypsin [83.9 U/mg], 65 mg of porcine intestinal peptidase [102 U/g] and distilled water). Also, a protein-water suspension was prepared (6.25 g of protein/L). The pH of multienzyme solution and protein suspension was adjusted to 8.0 using 0.1 N HCl and 0.1 N NaOH solutions. Also, 5 ml of multienzyme solution were mixed with 50 ml of the protein suspension employing constant stirring in a water bath at 37 °C. The extent of hydrolysis was determined by pH-drop after a 10 min incubation period (X), and the percentage of protein digestibility (IVPD) was calculated by the regression equation:
where X is the pH of protein suspension at 10 min of digestion with the multi-enzymatic system.
Preparation of extruded defatted chia (EDC) flour
Production of EDC flour was carried out according to the methodology proposed by León-López et al. (2019). For this, 500 g lots of partially defatted chia seeds (lipids content = 5%-6%, DW) were placed in a domestic blender at low velocity, the grits obtained were ground in the same blender to obtain grits that passed through a 40-US mesh (0.425 mm) screen. The extrusion cooking was carried out on a single screw laboratory extruder, model 20 DN (CW Brabender Instruments, Inc, NJ, USA), at extrusion temperature (ET) and screw speed (SS) of 147 °C and 237 rpm, respectively. Extrudates were cooled and dried at environmental conditions (25 °C, RH = 65%), milled to pass through an 80-US mesh (0.180 mm) screen, packed in plastic bags, and stored at 4 °C. EDC flour was analyzed for chemical composition, nutritional and nutraceutical properties.
Functional gluten-free beverage (GFB) preparation. Sensorial evaluation
The GFB was developed from the mixture: 70% OEQ flour + 30% EDC flour. The mixture (25 g) was added with 2.5 g of sweetener SteviaTM (no-calorie), vanillin powder (1 g), and water (170 ml). The suspension was stirred with a domestic shaker (medium velocity), refrigerated (8 °C-10 °C), and sensory evaluated for acceptability. The sensorial evaluation was carried out by 80 non-trained panelists using a labeled affective magnitude (LAM) scale. These values were transformed to a 0-100 scale (Cardello & Schutz, 2004).
Chemical composition
The official methods (Association of Official Analytical Chemists [AOAC], 2012) were used to determine protein (micro-Kjeldahl method 960.52), lipids (920.39), and moisture (method 925.09B) contents. Soluble and insoluble dietary fiber (SDF/IDF) were evaluated according to the enzymatic-gravimetric method for total dietary fiber (TDF) (method 985.29) (AOAC, 2012).
Nutritional properties: essential amino acid (EAA), chemical score (CS), and calculated protein efficiency ratio (C-PER)
The EAA composition was determined using an analytical scale (4.6mm × 250mm) hypersil ODS C18 column kept at 38 °C and connected to an HPLC system (León-López et al., 2019). The HPLC system (GBC, Dandenong, Australia) was equipped with a fluorescence detector >LC 5100 set at 270 nm (excitation) and 316 nm (emission). We used an ultraviolet detector to detect tryptophan at 280 nm.
The chemical score (CS) was calculated as CS = (Content of the most limiting EAA/REAAR) x 100; EAA = Essential amino acid and REAAR = Recommended EAA requirements for three-year-old children and older, adolescents, and adults (Food and Agriculture Organization of the United Nations [FAO], 2013).
C-PER was calculated as described by Satterlee et al. (1982) and summarized by the AOAC (2012). This procedure is based on the IVPD and the EAA composition of the optimized mixture. All determinations were made in triplicate.
ACE inhibitory potential
The angiotensin-converting enzyme (ACE) inhibitory activity in free and bound phenolic extracts was determined using the Dojindo ACE Kit-WST test kit (Dojindo Laboratories, Kumamoto, Japan). Briefly, 20 µL of sample solution was added to a sample well, and 20 µL of deionized water was added to blank 1 and blank 2 wells. 20 µL of substrate buffer was added to each well. 20 µL of deionized water were added to blank 2 well, while 20 µL of enzyme working solution (ACE 0.2 U/ml, aminoacylase 172 kU/ml, and 3HBGGG 3.4 mg/ml) was added to each sample and blank 1 well. All wells containing above solutions were incubated at 37 °C for 1 h. Afterwards, 200 µL of the indicator working solution (0.333 mM NAD+, 0.333 mM WST-1, 0.1 mM EDTA, 0.633 U ml-1 diaphorase, and 0.700 U ml-1 3-hydroxybutyrate dehydrogenase) was added to each well with further incubation for 10 min. The yield of 3HB was monitored indirectly through formazan concentration, which was measured at 450 nm using a Microplate Reader (SynergyTM HT Multi-Detection, BioTek, Inc., Winooski, VT, USA). ACE inhibition was calculated from the equation:
where Ablank1 represented positive control (without ACE inhibition), Ablank2 was the absorbance of the reagent blank (negative control), and Asample was the absorbance of the sample (extract). The concentration of extract inducing 50% inhibition (IC50) of ACE was determined with non-linear regression using the Prism v5 software (GraphPad Prism) (Gámez-Valdez et al., 2021).
Statistical analyses
The experimental results on the chemical composition, nutritional properties, antioxidant activity, phenolic compounds content, and ACE inhibitory potential (IC50) of flours were subjected to analysis of variance using the statistical software MINITAB 17.0; the multiple range Duncan’s test at p ≤ 0.05 was used to compare differences among means.
Results
Prediction models for antioxidant activity (AoxA), total phenolic content (TPC), and in vitro protein digestibility (IVPD) for extruded quinoa (EQ) flours
The experimental values for AoxA, TPC, and IVPD of EQ varied from 13 230 µmol TE/100g sample (DW) to 16 470 µmol TE/100g sample (DW), from 475 mg GAE/ 100g sample (DW) to 640 mg GAE/ 100g sample (DW), and from 78.2% to 87.2 %, respectively (Table 1). The analysis of variance (Anova) showed that AoxA, TPC, and IVPD depend significantly (p < 0.1) on ET and SS linear and quadratic terms. The prediction models, using decoded variables, for the response variables were:
The regression models explained 94.7%, 96.4%, and 91.7% of the total variability (p < 0.001) in AoxA, TPC, and IVPD. The lack of fit was not significant (p > 0.05). The relative dispersion of the experimental points from the predictions of the models (CV) was < 10%. These values indicated that the experimental models were adequate and reproducible. In general, AoxA and TPC of extruded quinoa (EQ) flours increased with ET until reaching maximum values around 140 °C and 240 rpm (Figure 1a, 1b), while the IVPD of EQ increased with ET showed maximum values at high ET (140 °C) and intermediate SS (150 rpm) (Figure 1c). The potential of pseudo-cereals seeds as functional food has been related to their antioxidant activity and other nutraceutical properties (antihypertensive, antidiabetic, anti-inflammatory, antiatherosclerotic) associated with phenolic compounds and bioactive peptides (Martínez-López et al., 2020; Orona-Tamayo et al., 2018; Tan et al., 2020).
Optimization of the whole quinoa seeds extrusion process
Figure 1d, which was obtained from the superimposition of response variables contour plots (Figure 1a, 1b, 1c), was utilized to determine the best combination of process variables for the production of optimized extruded quinoa (OEQ) flour. The central point of the optimization region in Figure 1d corresponds to the optimum combination of process variables (ET = 138 °C/SS = 138 rpm) for producing OEQ flour with the highest AoxA, TPC, and IVPD values. The predicted values of AoxA, TPC, and IVPD, using the predictive models of each response variable and the optimal conditions of extrusion, were 15 992 µmol TE/100 g sample (DW), 618.4 mg GAE/100 sample (DW), and 88.7%, respectively (Figure 1d). The experimental values of AoxA, TPC, and IVPD of OEQ flour (Table 4) were similar to the predicted values, meaning that the extrusion process’s optimal conditions were appropriate and reproducible.
Table 2 Phenolic content and antioxidant activity of quinoa and chia flours.
Property | Unprocessed whole quinoa flour |
OEQ1 flour | Unprocessed defatted chia flour |
EDC1 flour |
Phenolic compounds2 | ||||
Free | 174.5 ± 15.2A | 176.1 ± 14.2A | 248.94 ± 2.97B | 302.44 ± 4.81A |
Bound | 312.6 ± 10.1B | 452.7 ± 10.2A | 252.32 ± 4.19A | 339.40 ± 3.61B |
Total | 487.1 ± 17.6B | 628.8 ± 14.6A | 501.26 ± 12.70B | 641.84 ± 14.2A |
Antioxidant activity3 | ||||
Free phytochemicals | 3570 ± 198A | 3673 ± 183A | 8456 ± 680B | 10 781 ± 898A |
Bound phytochemicals | 10 710 ± 710B | 12 258 ± 872A | 14 268 ± 1102B | 15 986 ± 975A |
Total phytochemicals | 14 280 ± 927B | 16 129 ± 933A | 22 724 ± 1132B | 26 767 ± 1076A |
A-B Means with the same letter, in first and second columns, are not different (Duncan, p > 0.05 - no significant difference); A-B Means with the same letter, in third and fourth columns, are not different (Duncan, p > 0.05 - no significant difference); 1 OEQ = Optimized extruded whole quinoa, EDC = Extruded defatted chia; 2 mg Gallic acid equivalents (GAE)/100 g sample (DW); 3 µmol Trolox equivalents (TE) / 100 g sample (DW).
Source: Author’s own elaboration.
Table 3 Chemical composition and nutritional/nutraceutical properties of quinoa and chia flours.
Property | Unprocessed whole quinoa flour |
OEQ1 flour | Unprocessed defatted chia flour |
EDC1 flour | Standard FAO2 |
Chemical composition (%, DW) | |||||
Protein | 15.95 ± 0.07A | 15.99 ± 0.07A | 31.09 ± 0.37A | 30.66 ± 0.29A | --- |
Lipids | 6.02 ± 0.07A | 5.63 ± 0.07B | 5.27 ± 0.06A | 5.12 ± 0.07B | --- |
Minerals | 3.50 ± 0.07A | 3.54 ± 0.07A | 6.88 ± 0.07A | 6.91 ± 0.08A | |
Dietary fiber | --- | ||||
Soluble | 2.46 ± 0.05B | 3.22 ± 0.03A | 6.71 ± 0.09B | 7.36 ± 0.07A | --- |
Insoluble | 8.03 ± 0.04A | 7.34 ± 0.07B | 41.21 ± 0.13B | 43.01 ± 0.13A | --- |
Total | 10.49 ± 0.08A | 10.56 ± 0.07A | 47.92 ± 0.21B | 50.37 ± 0.25A | --- |
Carbohydrate | 64.04 ± 0.19B | 64.28 ± 0.22B | 8.84 ± 0.03A | 6.94 ± 0.03B | |
Nutritional | |||||
EAA3 (g/100 g protein) | |||||
His | 2.82 + 0.02A | 2.76 + 0.02A | 1.99 + 0.04A | 2.01 + 0.03A | 1.60 |
Ile | 4.57 + 0.03A | 4.50 + 0.04A | 3.37 + 0.03A | 3.29 + 0.04A | 3.00 |
Leu | 7.03 + 0.04A | 6.88 + 0.03A | 6.17 + 0.03A | 6.08 + 0.05A | 6.10 |
Lys | 5.83 + 0.03A | 5.72 + 0.03A | 4.48 + 0.04A | 4.40 + 0.05A | 4.80 |
Met+Cys | 4.60 + 0.05A | 4.59 + 0.02A | 2.69 + 0.03A | 2.48 + 0.02B | 2.30 |
Phe+Tyr | 7.17 + 0.03A | 7.01 + 0.05A | 5.18 + 0.02A | 5.02 + 0.03B | 4.10 |
Thr | 3.59 + 0.04A | 3.61 + 0.04A | 2.58 + 0.04A | 2.51 + 0.04A | 2.50 |
Trp | 0.91 + 0.01A | 0.94 + 0.02A | 1.63 + 0.02A | 1.54 + 0.05B | 0.66 |
Val | 4.38 + 0.04A | 4.41 + 0.03A | 4.23 + 0.02A | 4.25 + 0.04A | 4.00 |
Total | 40.90 | 40.42 | 32.32 | 31.58 | 29.06 |
Chemical score | 100 | 100 | 93.33 | 91.66 | |
Limiting EAA | None | None | Lys | Lys | --- |
IVPD4 (%) | 68.2 ± 0.17B | 82.1 ± 0.12A | 77.9 ± 0.13B | 84.05 ± 0.18A | |
C-PER5 | 1.94 | 2.37 | 1.69 | 2.15 | --- |
Nutraceutical | |||||
AoxA | 14 280 ± 927B | 16 129 ± 933A | 22 724 ± 1132B | 26 767 ± 1076A | |
ACE7 Inhibitory potential (IC50)8 | 0.907 | 0.150 | 0.871 | 0.210 | --- |
A-B Means with the same letter, in first and second columns, are not different (Duncan, p > 0.05 - no significant difference); A-B Means with the same letter, in third and fourth columns, are not different (Duncan, p > 0.05 - no significant difference); 1 OEQ = Optimized extruded quinoa, EDC = Extruded defatted chia; 2 EAA requirements for children, adolescents and adults (3 years old and older) according to FAO (2013); 3 EAA = Essential amino acid, 4 IVPD = in vitro protein digestibility, 5 C-PER = Calculated protein efficiency ratio, 6 µmol Trolox equivalents (TE)/100 g sample (DW); 7 angiotensin converting enzyme; 8 mg extract/ml suspension.
Source: Author's own elaboration.
Table 4 Chemical composition and nutritional/nutraceutical properties of the mixture.
Property | Mixture (70% OEQ +30% EDC)1 |
Standard FAO2 |
Chemical composition (%, DW) | ||
Protein | 20.39 | --- |
Lipids | 5.48 | --- |
Dietary fiber | ||
Soluble | 4.46 | --- |
Insoluble | 18.04 | --- |
Total | 22.50 | --- |
Nutritional | ||
EAA3 (g/100 g protein) | ||
His | 4.24 | 1.60 |
Ile | 4.14 | 3.00 |
Leu | 6.64 | 6.10 |
Lys | 5.40 | 4.80 |
Met+Cys | 3.96 | 2.30 |
Phe+Tyr | 6.41 | 4.10 |
Thr | 3.28 | 2.50 |
Trp | 1.09 | 0.66 |
Val | 4.36 | 4.00 |
Total | 39.52 | 29.06 |
Chemical score | 100 | --- |
Limiting EAA | None | --- |
In vitro protein digestibility (%) | 81.12 | --- |
C-PER4 | 2.28 | --- |
Nutraceutical | ||
Antioxidant activity5 | 18 943 | --- |
ACE6 Inhibitory potential (IC50)7 | 0.271 | --- |
1 OEQ = Optimized extruded quinoa flour, EDC = Extruded defatted chia flour; 2 EAA requirements for children, adolescents, and adults (3 years old and older) according to FAO (2013); 3 EAA = Essential amino acid, 4 C-PER = Calculated protein efficiency ratio, 5 µmol Trolox equivalents (TE)/100 g sample (DW); 6 Angiotensin converting enzyme; 7 mg extract/ml suspension.
Source: Author's own elaboration.
Antioxidant activity (AoxA) and total phenolic content (TPC) of optimized extruded quinoa (OEQ) flour
The extrusion of quinoa seeds increased (p < 0.05) AoxA (12.95%) and bound (44.81%) and total (29.09%) phenolic compounds when compared with unprocessed whole quinoa seeds (Table 2). These results show that the bound phenolics were the primary contributors (64%-72%) to the antioxidant activity in unprocessed and optimized extruded quinoa flours (Table 2).
Antioxidant activity (AoxA) and total phenolic content (TPC) of extruded defatted chia (EDC) flour
The extrusion cooking of defatted chia seeds increased (p < 0.05) AoxA in 17.80%, when compared with unprocessed defatted chia seed (26 767 µmol TE/100 sample, DW vs. 22 724 µmol TE/100 sample, DW) (Table 2). The AoxA of free, bound, and total phenolic compounds increased (p < 0.05) by 21.49%, 34.51%, and 28.04%, respectively, after the optimized extrusion cooking process (Table 2).
Chemical composition, essential amino acid (EAA) content, in vitro protein digestibility (IVPD), and C-PER of optimized extruded quinoa (OEQ) and extruded defatted chia (EDC) flours
The extrusion process caused slight changes on chemical composition of quinoa and chia seeds during production of OEQ and EDC flours (Table 3). Milán-Carrillo et al. (2012), Argüelles-López et al. (2018), and León-López et al. (2019) reported similar results to the extrusion of the pseudo-cereals amaranth and chia.
Table 3 shows the essential amino acid content of unprocessed and processed flours. In general, the essential amino acid content of unprocessed and extruded quinoa/chia flours were higher than those suggested by FAO (2013) for the essential amino acid requirement for children (3 years old and older), adolescents, and adults. Lys was the only one presented as limiting amino acid in unprocessed and extruded defatted chia flours. This tendency is similar to literature results of essential amino acid content in amaranth (Argüelles-López et al., 2018) and chia (León-López et al., 2019) seeds. The extrusion process caused only a slight decrease (p < 0.05) in the content of some EAA (Met+Cys, Phe+Tyr, and Trp) in EDC flour. Unprocessed and extruded quinoa/chia flours present a high EAA chemical score (91.66-100.00). On the other hand, the extrusion process increased IVPD of OEQ and EDC flours in 20% and 8%, respectively. Results in Table 3 also indicated that C-PER increased during the OEQ and EDC flours preparation.
ACE Inhibitory potential of optimized extruded quinoa (OEQ) and extruded defatted chia (EDC) flours
The phenolic extracts of OEQ and EDC flours had better ACE inhibitory potential (IC50 = Concentration of phenolic extracts in mg extract/ml suspension, required to produce an inhibition of 50% of the activity of ACE) than phenolic extracts from unprocessed quinoa (0.150 mg extract/ml vs. 0.907 mg extract/ml) and defatted chia (0.210 mg extract/ml vs. 0.871 mg extract/ml) flours, respectively (Table 3).
Chemical composition and nutritional/nutraceutical properties of the mixture of flours (70% OEQ + 30% EDC)
The mixture contains 20.39% proteins, 5.48% lipids, and 22.50% total dietary fiber (TDF) on a dry basis. The EAA content in the mixture was greater than that recommended (FAO, 2013) for three-year-old children, adolescents, and adults (39.52 g/100 g protein vs. 29.06 g/100 g protein); their chemical score was 100. It did not present limiting EAA (Table 4). The mixture presented IVPD and calculated protein efficiency ratio (C-PER) of 81.12% and 2.28, respectively (Table 4). A mixture of nixtamalized maize (26.7%) and extruded chickpea (73.3%) flours had IVPD and C-PER of 54.6% and 1.86, respectively (Alarcón-Valdez et al., 2005).
The mixture had an antioxidant activity of 18 943 µmol TE/100 g (DW) and an antihypertensive potential, expressed as IC50, of 0.271 mg extract/ml suspension (Table 4). This IC50 value was lesser than those reported for soybean protein hydrolysates (80 µg/ml-360 µg/ml) (Pyo & Lee, 2007) and for protein fractions of amaranth (albumins: 0.35 mg/ml; globulins: 15 mg/ml) (Tovar-Pérez et al., 2009).
Formulation, nutrimental composition, energy content, and antioxidant activity of the functional gluten-free beverage (GFB) are prepared from the mixture
The formulation of the GFB (main ingredient: mixture of flours [70% OEQ + 30% EDC]) was based on the preparation of traditional drinks consumed in Mexico made from grains (e.g., rice, barley, maize). Sensory evaluations were carried out to define the quantities of the ingredients. The formulation originated a beverage containing 12.6% of the main ingredient (mixture) and a protein content > 1.5%, with quality similar to casein. SteviaTM (Non-caloric vegetable sweetener) was used to comply with the Ministry of Health recommendation: “A 200 ml portions of the beverage (food) should not contain more than 140 kcal”.
A portion of 200 ml of GFB prepared with 25 g of the mixture of flours (70% OEQ + 30% EDC) contains 5.61 g good protein quality, 1.50 g lipids, 12.92 g carbohydrates, and dynamic content of 88 kcal (Table 5). This portion of beverage covers between 35% and 23.3% of the daily protein requirements for children of 1-3 and 4-8 years of age. Functional beverage (200 ml) had the total hydrophilic antioxidant activity of 5091 µmol TE, which contributes 100%-169% of the recommended daily consumption of antioxidants (from 3000 µmol TE to 5000 µmol TE) (United States Department of Agriculture [USDA], 2010). Untrained panelists assessed with an average value of 83 the acceptability of the GFB (satisfaction level between “I like it very much” and “I really like it”). It is expected that this acceptability of beverages will allow for adequate consumption and, thus, can provide benefits to consumers’ health.
Table 5 Nutrimental and energy contents, antioxidant activity and acceptability of a portion of 200 ml of the functional gluten free beverage (GFB).
Property | Functional gluten free beverage (GFB)1 |
Nutriment (g) | |
Protein | 5.61 |
Lipids | 1.50 |
Dietary fiber | |
Soluble | 1.25 |
Insoluble | 4.78 |
Total | 6.13 |
Carbohydrates | 12.92 |
Energy (kcal) | 88 |
AoxA2 ((mol TE) | 5091 |
Acceptability3 | 83 |
1 Main ingredient: Mixture = 70% optimized extruded whole quinoa flour + 30% extruded defatted chia flour; 2 AoxA = Antioxidant activity; 3 A value between “I like it very much” and “I really like it” on a hedonic scale of 11 points.
Source: Author's own elaboration.
Discussion
Antioxidant activity (AoxA) and total phenolic content (TPC) of optimized extruded quinoa (OEQ) flour
These results coincide with the reported by Milán-Carrillo et al. (2012), Argüelles-López et al. (2018), and Song et al. (2020). They observed that the extrusion of a pseudo-cereal (amaranth, chia, and quinoa) increased AoxA and TPC when compared with crude grains, which improves its functionality and therapeutic potential. This behavior could be attributed to (1) the breaking of conjugated phytochemicals and release free of phytochemicals (Dewanto et al., 2002), (2) the prevention of enzymatic oxidation, and (3) the darker colors of the extruded flours indicating the formation of Maillard reaction products having antioxidant properties (Fares & Menga, 2012).
Bioactive phytochemicals exist in free, soluble-conjugated, and bound forms. Bound phytochemicals, mostly in cell wall materials, are difficult to digest in the upper intestine and may be digested by bacteria in the colon to provide health benefits and reduce colorectal cancer incidence. Dietary polyphenols significantly impact cancer stem cells (CSC), which may be applied in cancer prevention and treatment (Liskova et al., 2019). Colorectal cancer (CRC) is a major cancer type and a leading cause of death. Current medical treatments are not sufficient due to the lack of effective therapy, chemoresistance, adverse side effects, and disease recurrence. Recently, epidemiologic observations have highlighted the association between the ingestion of foods enriched with phytochemicals and nutrients and the lower CRC risk. According to preclinical studies, dietary phytochemicals exert chemopreventive effects on CRC by regulating different markers and signaling pathways. Additionally, gut microbiota plays a strong effect on CRC onset and progression (Afrin et al., 2020).
Antioxidant activity (AoxA) and total phenolic content (TPC) of extruded defatted chia (EDC) flour
Gómez-Favela et al. (2017) and León-Murillo et al. (2021) reported similar values of AoxA to free, bound, and total phenolic compounds in chia seeds. León-Murillo et al. (2021) and Argüelles-López et al. (2018) reported that the application of the extrusion process to pseudo-cereals such as chia and amaranth, at optimal conditions, increased the AoxA and bound and free phenolic content when compared to unprocessed seeds.
Antioxidant activity increase could also be a result of the release of antioxidant phenolic compounds during extrusion process. Prevention of oxidation could be could be caused by phenolic compounds in the extruded product, enzymatic inactivation during the processing and due to the presence of Maillard reaction products with antioxidant activity, generated during extrusion of raw material that contains amino acids and reducing sugars (Argüelles-López, et al., 2018).Félix-Medina et al. (2021) stated that the extrusion process conditions (humidity, temperature, screw speed) have a crucial impact on the levels of bioactive compounds (phenolic acids, flavonoids) by releasing, by degrading, or by composition. The increase in the AoxA might be due to the products formed during the Maillard reaction. These non-enzymatically formed products have affinities for unstable chemical radicals, thus inflating the value of AoxA. However, in some cases, the level of bioactive compounds in the extruded products may have elevated certain phenolic acid levels due to their release from the cell wall matrix (Félix-Medina et al., 2020; Ramos-Enriquez et al., 2018)
Chemical composition, essential amino acid (EAA) content, in vitro protein digestibility (IVPD), and C-PER of optimized extruded quinoa (OEQ) and extruded defatted chia (EDC) flours
According to literature reports, extrusion is a benevolent process with the proximal chemical composition and essential amino acid content of grains such as cereals and pseudo-cereals because it is a high-temperature, short-time (HTST) process (Argüelles-López et al., 2018; Gámez-Valdez et al., 2021; León-Murillo et al., 2021; León-López et al., 2019; Reyes-Moreno et al., 2018).
During food processing by extrusion, both an IVPD increase and an IVPD decrease may occur. This increase of IVPD occurs due to the destruction of labile anti-nutritional factors (lectins, trypsin and chymotrypsin inhibitors) and protein denaturation, as a result of the applied conditions (shearing forces, temperature, and humidity) during processing (Argüelles-López et al., 2018; Félix-Medina et al., 2021; León-López et al., 2019; Reyes-Moreno et al., 2018). The decrease of IVPD in extrusion occurs because the components of dietary fiber, phenolic compounds, and the products of the Maillard reaction (reaction carried out between reducing sugars and amino acids of the proteins) can bind to protein, partially unavailing it for its digestion (Reyes-Moreno et al., 2018). However, the increase of IVPD during food processing by extrusion is the most reported phenomenon in literature (León-López et al., 2019), which agrees with the results obtained in the present investigation.
The nutritional parameter C-PER of unprocessed and extruded flours was calculated using the IVPD and the EAA composition of the samples, so its increase was mainly due to the increase in IVPD, since the EAA content only slightly decreased in EDC flour, while in OEQ flour this EAA content did not undergo significant changes (p > 0.05) (Félix-Medina et al., 2021; León-López et al., 2019).
ACE inhibitory potential of optimized extruded quinoa (OEQ) and extruded defatted chia (EDC) flours
The IC50 values to ACE inhibitory potential obtained in this research are in concordance with reported results for phenolic extracts of extruded blue maize and extruded defatted chia flour (0.51 extract mg/ml and 0.38 extract mg/ml, respectively) and extruded amaranth flour (0.42 mg/ml) (Argüelles-López et al., 2018; Gámez-Valdez et al., 2021; León-Murillo et al., 2021). During the extrusion of whole quinoa seeds and defatted chia seeds, the release of phytochemicals with biological activity (e.g. ACE inhibitory activity) occurs; in this study, an increase in the content of bound and total phenolic compounds was observed during the production of OEQ and EDC by extrusion (Table 2). Also, during the gastrointestinal digestion other bioactive compounds with ACE inhibition capacity are released. The phytochemicals with ACE inhibitory potential released during both processes above mentioned have the potential to reduce the content of angiotensin II (vasoconstrictor) and increase the levels of Bradykinin (vasodilator) in hypertensive people, resulting in a decrease in their blood pressure. Phenolic compounds (phenolic acids, flavonoids, tannins, stilbenes) can inhibit the in vitro ACE activity. In the literature, some hypotheses on the mechanisms of inhibition of phenolic compounds on ACE have been posed: competitive inhibition, by the structure of phenols that alter their function by agglutination; noncompetitive inhibition, both substrate and inhibitor bind to the enzyme simultaneously and reversibly; metal sequestration, ACE is a Zn (II)-dependent metalloproteinase, and phenolic compounds can chelate non-specific metals exhibiting an ACE inhibitory effect; and the interactions between phenols and the disulfide bridges (oxidized cysteines) reside on the surface of the ACE, causing slight modifications in the structure of the ACE (Al Shukor et al., 2013; Gámez-Valdez et al., 2021; León-Murillo et al., 2021). Also, peptides released during enzymatic digestion has shown ACE inhibitory activity. Montoya-Rodríguez et al. (2015) reported that extruded amaranth flours were sources of peptides with potential biological activity, such as ACE-inhibitor. The extrusion process had a higher impact on the peptide profile, producing more peptides with lower molecular mass and biological activity. Previous studies have shown that the amino acid composition of a peptide has a significant effect on its ACE inhibitory activity, and it has been reported that ACE prefers to bind to a polypeptide with a high content of hydrophobic amino acids. Hydrophobic peptides can coordinate to the Zn (II) ion. This interaction may have caused distortion of the tetrahedrally coordinated Zn (II), which further resulted in the loss of ACE activity (Sun et al., 2019).
In the literature, it has also been reported that Maillard reaction products (MRP) exhibit ACE inhibitory activity in vitro. The in vitro ACE inhibitory activity of melanoidins (end products of the Mallard reaction) from food is similar to that reported for well-known antihypertensive peptides. Bounded melanoidin compounds (BMC), e.g., polyphenols, and low molecular weight MRP, which are non-covalently bound to the melanoidin backbone, also contribute to ACE inhibitory activity. On the other hand, it has also been reported that the in vitro ACE inhibitory activity of melanoidins is related to the structure of melanoidin, and that the melanoidin chromophores are not involved. Melanoidins are low molecular weight pigments or high molecular weight compounds with low molecular weight chromophore (Wang et al., 2011).
Chemical composition and nutritional/nutraceutical properties of the mixture (70% OEQ + 30% EDC)
TDF is an important functional component in the human diet. It possesses recognized health properties and can reduce the risk of specific chronic diseases, such as diabetes type-2 and coronary heart disease. It has been proven that TDF is an ingredient of commercial functional foods designed for consumers who are concerned about elevated circulating blood glucose and cholesterol levels (Yegin et al., 2020).
According to León-López et al. (2019), the C-PER technique predicted the same differences observed in the rat bioassay (PER); both techniques C-PER and PER can detect significant differences among samples of different origin. Therefore, it is recommended to use the in vitro techniques as fast, accurate indicators of protein digestibilities and PER.
Formulation, nutrimental composition, energy content, and antioxidant activity of the functional gluten-free beverage (GFB) are prepared from the mixture
The Mexican norm NMX-F-439-1983 for food and non-alcoholic beverages (Secretaría de Comercio y Fomento Industrial, 1983) defines a beverage as nutritional when it contains at least 1.5% protein or hydrolyzed proteins with a quality equivalent to that of casein; beverages must contain between 10% and 25% main ingredient. These may contain about 2% ethanol, carbon dioxide, flavoring agents, juices, fruit pulp, sweeteners, vegetables or legumes, and other additives authorized by the Mexican Ministry of Health.
Conclusions
The extrusion process is an excellent technological strategy that increased the nutritional value, antioxidant activity, total phenolic content, and inhibitory ACE potential of whole quinoa and chia seeds. Extruded defatted chia flour added to extruded whole quinoa flour enhanced nutritional, antioxidant, and antihypertensive potential of the mixture used to produce a functional gluten-free beverage. Therefore, this functional beverage can be used for health promotion and as an alternative to beverages with high caloric content and low nutritional value, which predominate in a market of consumers with significant trends or incidences of overweight/obesity and chronic degenerative diseases such as hypertension. Additionally, the novel functional gluten-free beverage is an excellent alternative to promote healthy living in people with celiac disease.
Conflicts of interest
The authors declare no conflict of interest.