INTRODUCTION
Hydrogels are highly hydrophilic polymers that are capable of absorbing and releasing substantial amounts of fluids because of their elastic three-dimensional networks and the presence of functional groups such as OH-, COOH-, and CONH- (Ahmed 2015). The degree of swelling, absorption capacity, and permeability are qualities of hydrogels, and these abilities depend on the type of material with which the hydrogel has been manufactured (Bai et al. 2010, Ekebafe 2011, Asgher et al. 2012, Ahmed 2015). Some factors can affect the degree of swelling in hydrogels, such as pH, ionic strength, temperature, and electromagnetic radiation (Chen and Zhao 2000, Ekebafe 2011, Ahmed 2015). Most applications of hydrogels are focused on the field of medicine and the controlled release of drugs. Currently, research has been developed for the application of hydrogels in agriculture, for the partial release of pesticides, in bioremediation by creating fungal inoculums, and in the capture of metal ions for automotive and industrial use waters (Galhaup et al. 2002, Escobar et al. 2003, Farrel et al. 2013, Ahmed 2015). Superabsorbent polymers are hydrogels that have been widely accepted for their liquid capture capacity and their wide application in various agricultural, pharmaceutical, and construction sectors, and can reach an absorption of up to 500 times their weight (Hublik and Schinner 2000, Kabiri and Zohuriaan-Mehr 2004, Kumar et al. 2009, Li 2010).
Molecular immobilization is a technique that allows the industrial application of enzymes because it improves stability, activity, and handling, which translates into prolonged activity times (Nannipieri and Bollag 1991, Ortiz et al. 2006, Liu et al. 2009, Muñoz and Zuluaga 2009). However, this process is carried out with polymerizations prepared at the time of the immobilization, and to date, there are no reports of using prefabricated copolymers for the immobilization of nutrient medium conditioned with enzymatic activities. Thus, this study aimed to develop an alternative to use Hydrogel 3005K4® as an immobilizing vehicle for a conditioned medium containing ligninolytic enzymes with application potential in bioremediation. The latter can be achieved thanks to the high absorption of the copolymer in liquid media and the interactions between the positive charges, specifically of the enzyme laccase. This enzyme has the most tolerance to changes in pH and temperature, generating an interaction with the negative charges of the networks formed by the acrylamide acrylate potassium copolymer. Once the copolymer absorbs the conditioned medium, it is kept in the three-dimensional network. As the copolymer dehydrates or is replaced with water, the conditioned medium containing the enzymes of interest is released.
MATERIALS AND METHODS
Copolymer
Hydrogel 3005K4®, a copolymer of acrylamide and potassium was used with two particle sizes, 1-2 mm and 2-4 mm. Hydrogel 3005K4® has an absorption capacity of 270 to 400 times its weight in deionized water. In water with 1000 ppm of NaCl only, the absorption capacity is 150 to 270 times its weight, and in the presence of different electrolytes, the absorption capacity is 100 to 150 times its weight.
Preparation of the conditioned medium
The ligninolytic fungus Trametes versicolor CDBB-H-1051 was obtained from the microbial collection of the Departamento de Biotecnología y Bioingeniería at Centro de Investigaciones y Estudios Avanzados of the Instituto Politécnico Nacional, Mexico. Petri dishes with potato dextrose agar (PDA) were inoculated with the fungi for propagation of the mycelium and were incubated at 30 ± 1 ºC for 10 days under natural light. The fungus was grown in liquid medium. The inoculum was prepared with 20 squares of 4.2 × 4.21 mm ligninolytic fungi grown in PDA medium added to 500 mL Erlenmeyer flasks with yeast-peptone-glucose (YPG) medium. The flasks were incubated with continuous stirring at 100 rpm at 30 ± 1 ºC for 10 days under natural light. Subsequently, the biomass was filtered and homogenized for 5.0 s with an electric hand blender in a sterile beaker. A 5.0% volume/volume (v/v) mixture of the homogenized fungus was added to the modified Galhaup medium (Pouci 2008) and allowed to incubate for 30 days at 30 ± 1 ºC and 100 rpm under natural light. Next, the medium was filtered through Whatman® 1 filter paper using a vacuum to separate the fungus from the culture medium, and the recovered liquid was termed conditioned medium.
Absorption of the conditioned Galhaup medium
To test the absorption of the conditioned medium in the polymer, different ratios of acrylamide copolymer and potassium acrylate were tested with conditioned medium as shown in table I.
TABLE I VARIABLES AND LEVELS USED TO EVALUATE THE ABSORPTION OF THE CONDITIONED MEDIUM (CM) IN THE ACRYLAMIDE AND POTASSIUM ACRYLATE COPOLYMER.
Variables | Levels | ||
Copolymer + Conditioned medium (w/v) | 0.05:10 (CM0) | 0.1:10 (CM1) | 0.2:10 (CM2) |
Size of copolymer | 1-2 mm 2-4 mm | 1-2 mm 2-4 mm | 1-2 mm 2-4 mm |
w/v = weigh / volume
CM0 = copolymer-conditioned medium ratio 0.05: 10 w/v.
CM1 = copolymer-conditioned medium ratio 0.1: 10 w/v.
CM2 = copolymer-conditioned medium ratio 0.2: 10 w/v.
In addition, the time taken by the copolymer to absorb the conditioned medium and the amount of copolymer needed to absorb 100% of the conditioned medium was measured (Chen and Zhao 2000, Bai et al. 2010, Ekebafe 2011, Asgher et al. 2012, Ahmed 2015). Finally, the desorption percentage of conditioned medium at 35 ºC was determined. Calculations of the percentage and degree of swelling and the percentage of absorption were performed using formulas 1, 2 and 3.
The percentage of hydration was determined from the following equation:
and the degree of swelling using the following formulae:
Percentage absorption:
Desorption of the conditioned Galhaup medium
The desorption of the conditioned medium was determined when the copolymer was exposed to room temperature on a Whatman® 1 filter paper under vacuum conditions. The completely hydrated copolymer with the conditioned medium was placed on a filter paper 3.6 cm in diameter and was left at room temperature (25-30 ºC) for 72 h. This procedure was performed in triplicate at 0, 3, 6, 24, 48, and 72 h to measure polymer moisture and weight. In addition, we determined whether there was desorption of the enzymes by performing a visual analysis (green color) of enzymatic activity on the filter paper using 1.8 mM 2,2’-azino-bis [3-ethylbenzothiazoline-6-sulfonic acid] (ABTS) directly. This analysis was based on the method described by Liu et al. in 2009, as there is no currently developed method to determine the enzymatic activity of this type of hydrogel.
To find the desorption of the conditioned medium from the copolymer in the soil, we used an 80 g soil sample from Centro Educativo de Riego of the División de Ciencias de la Vida (DICIVA) at the Universidad de Guanajuato. The sampling area was 330 m2 and was located at a latitude of 20º44’39.2” N, longitude 10º11’939.4” W, and 1750 m asl with rainy and dry seasons throughout the year. The temperature varies from 7 ºC to 32 ºC. The soil was managed by conservation tillage for 12 years. The soil is classified as pelic vertisol with loamy-clayey texture according to the analyses performed at the Laboratorio de Tecnologías para la Sustentabilidad of the DICIVA. The sample was ground and sieved in # 20 mesh. One hundred grams of soil and 15 g of hydrated copolymer with conditioned medium were placed into a glass jar. The treatments (polymer sizes 1-2 mm and 2-4 mm) were maintained at 28 ± 2.0 ºC for 24 days. Samples were evaluated in triplicate for 0, 2.5, 10 and 24 days, and for each time, the soil moisture was evaluated to determine how it changed with the release of the conditioned medium.
Desorption of the conditioned medium in water was performed by placing the previously hydrated copolymer with the conditioned medium, with two different weights (1.5 and 3 g) in 10 mL of water. The weight of the copolymer was assessed on days 0, 2, and 5, while the absorbance of the aqueous medium surrounding the copolymer was quantified at 230 nm using a UV-Vis spectrophotometer on days 0 and 5. These measurements were conducted to elucidate the desorption kinetics of the enzymes.
ChemSketch program version 14.000 (Copyright © 1994-2013 Advanced Chemistry Development, Inc.) was used to identify possible interactions of the conditioned medium and the copolymer. Also, an analysis of variance (ANOVA) type III sums of squares was performed.
RESULTS AND DISCUSSION
The absorption percentage obtained at various times of the conditioned medium showed a statistically significant correlation between the ratio of the copolymer-conditioned medium with the exposure time and copolymer particle size (p = 0.000, p = 0.000 and p = 0.026 with α = 0.05). The controls showed more efficient absorption in less time and had a lower amount of 100% copolymer absorption at 6 h at a ratio of 0.1:10 w/v copolymer-water (Table II). For both copolymer particle sizes, improved absorption was detected when 0.2 g of copolymer per 10 mL of conditioned medium was added, resulting in an absorption ratio 50 times the weight of the copolymer. This ratio is less efficient than that of the control at 0.1 g of polymer with 10 mL of water. According to the supplier, this copolymer can absorb 270 to 400 times its weight in deionized water and 150 to 270 times its weight in water with 1000 ppm of NaCl. Therefore, its absorption capacity was much lower than that reported by the supplier, although the fluid conditions were not the same. This result was attributed to the presence of ions with positive charges. Even with this absorption capacity, the costs of using this copolymer (approximately US $ 7) are below the use of other materials such as alginates (approximately US $ 170).
TABLE II PERCENTAGE OF ABSORPTION DETECTED IN THE ACRYLAMIDE AND POTASSIUM ACRYLATE COPOLYMER IN THE PRESENCE OF THE CONDITIONED MEDIUM (CM) RECOVERED FROM Trametes versicolor USING TWO PARTICLE SIZES.
Polymer size | Time (h) | % Absorption | ||
CM0 | CM1 | CM2 | ||
1-2 mm | 0 | 4.33 ± 0.06 | 6.00 ± 0.00 | 8.33 ± 0.06 |
3 | 31.33 ± 0.12 | 61.33 ± 0.12 | 100 ± 0.00 | |
6 | 32.67 ± 0.12 | 61.67 ± 0.07 | 100 ± 0.00 | |
24 | 32.67 ± 0.12 | 61.67 ± 0.07 | 100 ± 0.00 | |
Cont* 1-2 mm | 0 | 5.67 ± 0.00 | 12.67 ± 0.14 | 30.00 ± 1.73 |
6 | 94.33 ± 0.07 | 100.00 ± 0.00 | 100.00 ± 0.00 | |
24 | 89.33 ± 0.00 | 100.00 ± 0.00 | 100.00 ± 0.00 | |
2-4 mm | 0 | 4.00 ± 0.00 | 3.67 ± 0.06 | 5.00 ± 0.14 |
3 | 21.00 ± 0.00 | 46.33 ± 0.32 | 77.00 ± 0.14 | |
6 | 30.00 ± 0.20 | 54.67 ± 0.00 | 86.00 ± 0.00 | |
24 | 36.67 ± 0.14 | 60.67 ± 0.00 | 97.00 ± 0.00 | |
Cont* 2-4 mm | 0 | 6.67 ± 0.00 | 6.00 ± 0.14 | 5.50 ± 0.1 |
6 | 86.67 ± 0.57 | 100.00 ± 0.00 | 100.00 ± 0.00 | |
24 | 92.00 ± 0.00 | 100.00 ± 0.00 | 100.00 ± 0.00 |
*Cont = Water.
CM0 = copolymer-conditioned medium ratio 0.05: 10 w/v.
CM1 = copolymer-conditioned medium ratio 0.1: 10 w/v.
CM2 = copolymer-conditioned medium ratio 0.2: 10 w/v.
There are reports of absorption of various compounds, such as methylene blue, crystal violet and heavy metals or arsenic, by using specific designs of copolymers for each type of compound and manipulating the pH and temperature for their conditioning. Our experiment was conducted under pH 6.3 - 6.7 and room temperature (25 - 31 ºC) (Zhen and Zhang 2008, Singh et al. 2010, Villarroel et al. 2010, Snoeck et al. 2014). To optimize the absorption processes, the conditions and compound to be absorbed must be evaluated, and a specific hydrogel or copolymer design must be assessed, which can result in considerable increases in costs. We used a single type of copolymer in different treatments to reduce costs.
In the analysis of hydration and degree of swelling, we found 150 to 300 times increased hydration and swelling in the CM0 and CM1 control treatments with respect to the treatments with the conditioned medium (Table III).
TABLE III HYDRATION CAPACITY AND DEGREE OF SWELLING OF THE ACRYLAMIDE AND POTASSIUM ACRYLATE COPOLYMER IN THE PRESENCE OF THE CONDITIONED MEDIUM RECOVERED FROM Trametes versicolor USING TWO PARTICLE SIZES.
Polymer size | Time (h) | % Hydration | Degree of swelling | ||||
CM0 | CM1 | CM2 | CM0 | CM1 | CM2 | ||
1-2 mm | 0 | 634.41 ± 49.82 | 456.17 ± 68.55 | 373.71 ± 23.34 | 7.34 ± 0.5 | 5.56 ± 0.69 | 4.74 ± 0.23 |
3 | 5684.43 ± 99.69 | 5902.81 ± 147.91 | 4704.09 ± 71.60 | 57.84 ± 1.00 | 60.03 ± 1.48 | 48.04 ± 0.72 | |
6 | 5761.36 ± 59.00 | 5827.43 ± 75.72 | 4762.08 ± 103.66 | 58.61 ± 0.59 | 59.27 ± 0.76 | 48.62 ± 1.04 | |
24 | 5780.33 ± 87.90 | 5849.60 ± 193.62 | 4736.40 ± 95.77 | 58.80 ± 0.88 | 59.50 ± 1.94 | 48.36 ± 0.96 | |
Cont* 1-2 mm | 0 | 1008.42 ± 109.30 | 1059.84 ± 194.51 | 1234.44 ± 502.67 | 11.08 ± 1.09 | 11.60 ± 1.95 | 13.34 ± 5.03 |
6 | 15069.67 ± 728.03 | 7658.67 ± 139.89 | 3770.02 ± 47.29 | 151.70 ± 7.28 | 77.59 ± 1.40 | 38.70 ± 0.47 | |
24 | 17046.06+ ± 467.15 | 9392.13 ± 266.96 | 4678.44 ± 106.61 | 171.46 ± 4.67 | 94.92 ± 2.67 | 47.78 ± 1.07 | |
2-4 mm | 0 | 539.26 ± 71.62 | 239.93 ± 17.65 | 202.82 ± 56.77 | 6.39 ± 0.72 | 3.40 ± 0.18 | 3.03 ± 0.57 |
3 | 3501.71 ± 13.30 | 4218.09 ± 315.49 | 3431.06 ± 74.52 | 36.02 ± 0.13 | 43.18 ± 3.15 | 35.31 ± 0.75 | |
6 | 4719.37 ± 364.23 | 4867.23 ± 305.84 | 4124.11 ± 79.52 | 44.86 ± 6.32 | 49.67 ± 3.06 | 42.24 ± 0.80 | |
24 | 5622.87 ± 432.35 | 5448.30 ± 181.25 | 4331.40 ± 57.15 | 57.23 ± 4.32 | 55.48 ± 1.81 | 44.31 ± 0.57 | |
Cont* 2-4 mm | 0 | 707.37 ± 123.82 | 398.19 ± 154.94 | 161.90 ± 26.62 | 8.07 ± 1.24 | 4.98 ± 1.55 | 2.62 ± 0.27 |
6 | 12962.33 ± 963.47 | 7785.47 ± 418.44 | 3845.54 ± 121.25 | 130.62 ± 9.63 | 78.85 ± 1.98 | 39.46 ± 1.21 | |
24 | 14898.65 ± 207.61 | 9354.97 ± 197.60 | 4484.15 ± 130.86 | 157.64 ± 13.33 | 94.55 ± 1.98 | 45.84 ± 1.31 |
*Cont = Water
CM0 = copolymer-conditioned medium ratio 0.05: 10 w/v
CM1 = copolymer-conditioned medium ratio 0.1: 10 w/v
CM2 = copolymer-conditioned medium ratio 0.2: 10 w/v
Table III shows that the degree of swelling and hydration of the copolymer were similar between the control and the samples with conditioned medium in the CM2 treatment (1 - 2 mm copolymer with conditioned medium 4736.40 ± 95.77; control with water 4678.44 ± 106.61; 2-4 mm copolymer with conditioned medium 4331.40 ± 57.15; contro with water 4484.15 ± 130.86). This result shows that this relationship is ideal to recover the conditioned medium.
In the desorption process of the medium conditioned from the hydrogel, we found that both time and the copolymer’s size had a statistically significant effect on the release of the conditioned medium (p = 0.0000, p = 0.0013, respectively, with α = 0.05). In the case of desorption in soil, only time had a statistically significant effect, with day 10 being the maximum release point (p = 0.0000 with α = 0.05.) The desorption time of the conditioned medium decreased when exposed to the soil. In the case of soil, the desorption time was slower and began on day 10. The process was constant without desorption at three days of exposure for both particle sizes. Desorption reached a maximum desorption point of < 83%, as shown in table IV.
TABLE IV PERCENTAGE RELEASE OF CONDITIONED MEDIUM WHEN THE ACRYLAMIDE AND POTASSIUM ACRYLATE COPOLYMER WAS EXPOSED TO AIR AND SOIL.
Copolymer | Time (h) | % of conditioned medium released | |
CS | CL | ||
Exposed to | 0 | 0.00 ± 0.00 | 0.00 ± 0.00 |
Air | 3 | 16.33 ± 0.60 | 10.85 ± 0.05 |
24 | 51.72 ± 5.16 | 42.51 ± 4.41 | |
48 | 95.78 ± 0.90 | 72.03 ± 1.82 | |
72 | 97.1 ± 0.13 | 96.87 ± 0.22 | |
Soil | 0 | 0.00 ± 0.00 | 0.00 ± 0.00 |
48 | 41.90 ± 2.53 | 41.16 ± 3.76 | |
120 | 52.61 ± 1.36 | 51.16 ± 1.71 | |
240 | 74.74 ± 4.13 | 79.70 ± 0.72 | |
576 | 79.05 ± 2.87 | 82.56 ± 6.32 |
CS = 1-2 mm copolymer (small copolymer).
CL = 2-4 mm copolymer (large copolymer).
The release of the conditioned medium contained in the hydrogel in water was determined by changing the absorbance of the water. Due to the release of the characteristic color of the conditioned medium, it was possible to determine the desorption of this medium in water. The absorbances correlated with the amount of copolymer, showing that the absorbance increased with the amount of copolymer (Table V).
TABLE V RELEASE OF LACCASE WHEN THE ACRYLAMIDE AND POTASSIUM ACRYLATE COPOLYMER WAS EXPOSED TO WATER BY MEASURING THE ABSORBANCE AT 230 NM.
Copolymer | Time | Absorbance | |
CS | CL | ||
Water 10:3 | 0 | 0.19 ± 0.02 | 0.15 ± 0.03 |
120 | 0.75 ± 0.01 | 0.93 ± 0.02 | |
Water 10:1.5 | 0 | 0.10± 0.01 | 0.10 ± 0.01 |
120 | 0.37± 0.00 | 0.45 ± 0.06 |
10:3 = ratio of index-archivos10 mL of water to 3 g of copolymer with conditioned medium.
10:1.5 = ratio of 10 mL of water to 1.5 g of copolymer with conditioned medium.
CS= Small copolymer
CL= Large copolymer
In contrast, we detected that the copolymer, when in water, tended to release the conditioned medium and absorb the available water simultaneously. By this, the copolymer increased its weight by more than 70% when the ratio was 3 g of copolymer (with conditioned medium) to 10 mL of water and increased more than 85% when the ratio was 1.5 g of copolymer (with conditioned medium) to 10 mL of water (Fig. 1). From day 2, the weight of the copolymer varied between 0.02-0.4 g, showing that the release of the conditioned medium and the absorption of the water had ceased (Fig. 1).
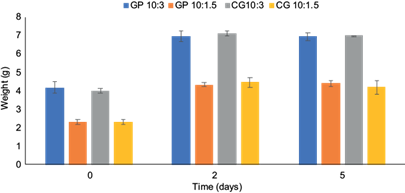
Fig. 1 Behavior of the copolymer with conditioned medium when immersed in water (CP = copolymer 1-2 mm; CG = copolymer 2-4 mm; 10:3 = ratio of 10 mL of water to 3 g of copolymer with conditioned medium; 10:1.5 = ratio of 10 mL of water to 1.5 g of copolymer with conditioned medium).
One possible factor that affected the percentage of absorption of the conditionate medium is the presence of cations such as Co+ and Mg+. The positive charges may compete for binding with the negative partial charges from the oxygen in the copolymer, causing interference between dipole-dipole bonds (Fig. 2). Additionally, the presence of enzymes that have a positive charge may compete with water and prevent the formation of hydrogen bonds with the molecules of the polymeric network that make up the copolymer, and can decrease their swelling and absorption capacities in a range of pH 5-9. Villarroel et al. (2010) used potentially biodegradable hydrogels obtained from polymerized and cross-linked acrylamide in the presence of the poly [3-hydroxybutyrate] biopolymer for the absorption of copper and nickel. They showed a high affinity and efficiency of absorption for copper molecules. This affinity results in a decrease in the capacity of the absorption process. During the desorption process on filter paper, enzymatic activity of laccase was detected, resulting in the characteristic green coloration of the ABTS oxidation reaction (Fig. 3).
CONCLUSION
It has been proven that the use of copolymers for the immobilization of nutrient medium conditioned with viable enzymes is possible. The copolymer had reduced absorption capacity in the presence of the conditioned medium recovered from T. versicolor due to possible interactions between the ion-dipole or dipole-dipole forces of the copolymer and the Mg+, Cu+, and enzymes with positive charges present in the conditioned medium. We found an absorption capacity of 50 times the weight of the copolymer. In the desorption process, the conditioned medium was released into water and soil and exposed to the environment, and we found that the copolymer had an affinity for water under the condition tested. Laccase activity was seen visually during desorption on filter paper. However, the initial and final enzyme units still need to be determined due to the conditions of the assay. These results help to consider whether this mixture could help to release the enzymes during a bioremediation process and at the same time, to keep the humidity on the plant.