INTRODUCTION
In the last two decades, the world population increased by 25.7 %, from 6.2 to 7.7 billion (GBD 2020), which caused an increase in a good quality and safe food production (Fung et al. 2018). In this sense, the intensive use of pesticides in agriculture has made it possible to satisfy the population’s food needs (Carvalho 2017). Pyrethroid insecticides stand-out because of their broad-spectrum activity towards the Lepidoptera order, their moderate persistence under field conditions, high potency, and cost-effectiveness (Weston et al. 2009, 2011, Pankaj et al. 2016).
Pyrethroids are synthetic insecticides derived from pyrethrin (derived from Chrysanthemum plants). This kind of insecticides has been used for more than 40 years and represent more than 30 % of the global insecticide market (Pietrantonio et al. 2007, Yao et al. 2015, Kalita et al. 2017). Cypermethrin (CYP) is a pyrethroid insecticide used worldwide in many crops due to its high efficacy (Tang et al. 2018). However, several studies have shown its acute toxicity to fish, invertebrates, and humans; therefore, their intensive use negatively impacts the environment and human health (Jin et al. 2011, Hocine et al. 2016, Ullah et al. 2018). The toxicity produced by CYP has been attributed mainly to two degradation metabolites, cis-3-(2’,2′-dichlorovinyl)-2,2-dimethylcyclopropane carboxylic acid (cis-DCCA), and 3-phenoxybenzoic acid (3-PBA) (Yao et al. 2015). Different strategies have been implemented in agriculture to reduce the impact of insecticides, such as integrated pest management (IPM), reduction in the use of insecticides, the use of genetically modified crops (GE), the creation and use of biopesticides, and the implementation of precision agriculture (Soberón et al. 2016). So far, bioremediation has been proposed as the most complete method of biodegradation of insecticides to restore the environment (Asim et al. 2021).
Bioremediation is considered an eco-friendly and cost-effective procedure for removing xenobiotics (polycyclic aromatic hydrocarbons, pesticides, chlorinated compounds, phenols, and others) of contaminated environments (Mao and Guan 2016, Mishra et al. 2021). Particularly, native microorganisms of contaminated environments represent an important source of bioremediation agents. Several studies aim to find genera of soil-derived bacteria with insecticide-degrading activity (Cycoń and Piotrowska-Seget 2016). Constant exposure to insecticides has allowed certain bacteria to develop the ability to use them as a substrate, generating less toxic or more assimilable metabolites that mitigate the environmental impact (Cycoń et al. 2009, Akbar and Sultan 2016). Bacteria such as strain Klebsiella sp. ZD112 (Wu et al. 2006), Serratia sp. JCN13, and Pseudomonas aeruginosa CH7 (Zhang et al. 2010, 2011), and Bacillus subtilis (Gangola et al. 2018) can degrade CYP through different mechanisms of action, mainly by hydrolytic enzymes (Fig. 1).

Fig. 1 Biodegradation of cypermethrin by B. subtilis and Klebsiella sp. Production of (A) 3-(2,2-dichloroethenyl)-2,2-dimethylcyclopropanecarboxylate, (B) 3-(2,2-dichlorovinyl)-2,2-dimethylcyclopropanecarboxylic acid, and (C) and 2-hydroxy-2-(3-phenoxyphenyl) acetonitrile.
In this study, the main objective was to isolate and identify new microorganisms capable of using CYP as a carbon or nitrogen source for growth, which can be used in CYP remotion. Bacteria were isolated from agricultural soil samples and their ability to biodegrade CYP in liquid media was determined. Main metabolites generated by the CYP degradation using strain Klebsiella pneumoniae YH43 were identified as biotransformation products.
MATERIALS AND METHODS
Sample collection
The sample collection site was in Fresnillo City, Zacatecas, Mexico (23º 12’ N-103º 30’ W). The soils are used for bean and corn crops, and CYP ([cyano-(3-phenoxyphenyl)methyl]-3-(2,2-dichloroethenyl)-2,2-dimethylcyclopropane-1-carboxylate) is periodically applied. Approximately one month after the last application of CYP, a total of 10 different soil samples were collected from a depth of 0-20 cm. Subsequently, the samples were mixed and homogenized (bean and corn crop soil, respectively) to obtain two composite samples (Ammari et al. 2015).
The samples were then transferred in airtight plastic bags to the Laboratorio de Biología of the Unidad Profesional Interdisciplinaria de Ingeniería, Instituto Politécnico Nacional Campus Zacatecas (Laboratory of Biology, Interdisciplinary Professional Engineering Unit, Zacatecas Campus of the National Polytechnic Institute) (UPIIZ-IPN).
Isolation of bacteria
One gram of the composite sample soil from each culture was used and dissolved in 20 mL of minimal saline medium (MSM). The composition of the MSM was g/L: 0.5 g (NH4)2SO4, 0.3 g K2HPO4, 0.01 g KCl, 0.01 g NaCl, 0.8 g dextrose, and 100 mg/L CYP ≥ 98 % from Sigma-Aldrich. The sample was incubated for 24 h at 35 ºC and 120 rpm. Samples were assayed by triplicate under sterile conditions.
After the incubation period, plates with nutrient agar (MCD) supplemented with CYP (100 mg/L) were inoculated by rod extension with the previously obtained culture. The plates were incubated in static conditions at 35 ºC for 24 h. The colonies were characterized morphologically with an optical microscope taking into consideration elevation, edge, shape, surface, and color. The colonies with different characteristics were selected and isolated.
Molecular identification
Genomic DNA extraction was performed on individually identified colonies. The Wizard Genomic DNA purification kit (Promega A1120, USA) was used according to the protocol described by the manufacturer. The 16s rDNA region was amplified by end-point PCR, using the Bac1-FW 5’-AGAGAGTTTGATCVTGGCTCAG-3’ and 16S-1400 RV 5’-GCGGTGTGTGTACAAGGCCCG-3’ oligonucleotides reported by Criollo et al. (2012). The reaction was adjusted to a final volume of 25 µL: 50 ng/µL gDNA, 1 X DNA polymerase Taq Buffer, 2.5 mM MgCl2, 0.2 mM dNTPs, 0.2 µM BAC-1FW, and 16S- 1400 RV, 0.125 U/µL GoTaq Polymerase. Amplification conditions were 94 ºC for 3 min, followed by 30 cycles of denaturation at 94 ºC for 30 s, alignment at 57 ºC for 30 s, extension at 72 ºC for 30 s, and a final extension at 72 ºC for 3 min in a Labnet Multigene TM mini thermal cycler.
The PCR products were purified following the ExoSAP-IT protocol (Affymetrix, Santa Clara, CA) and sequenced according to the conditions indicated in the BigDye Terminator v.3.1 cycle sequencing kit of the ABI 3130 system (Applied Biosystems, Foster City, CA). The electropherogram was visualized, edited, and assembled with Chromas Lite 2.1 (Technelysium) and SeqMan software from the Lasergene suite DNASTAR 8 (Madison, WI). The assembled nucleotide sequences were compared with the NCBI nr/nt database using the BLASTn tool to identify selected ClustalW bacteria (homology > 99 %; Koolivand et al. 2019). A phylogenetic tree was constructed with the sequences obtained using the ClustalW method (Tamura et al. 2007).
Growth in the presence of CYP as a carbon source
All assays were performed by triplicate under sterile conditions. Erlenmeyer flasks containing 20 mL of MSM supplemented with CYP (100 mg/L) were inoculated with 3 × 106 cells/mL of each selected bacterium. Flasks were incubated at 35 ºC and 120 rpm in a ZHWY 200B incubator (Zhicheng). Aliquots of 5 mL were drawn at different times (0, 6, 12, 24, 24, 36, 48, 56, 72, 96, 120, 140, 180, and 360 h) and placed in plastic microwells to determine the optical density at 600 nm (OD600 nm) in a Thermo Scientific Genesys 10S UV-Vis spectrophotometer. Three flasks were introduced as controls: 1 (MSM), 2 (MSM with inoculum and without CYP), and 3 (MSM only with CYP).
CYP biodegradation assay
For the CYP biodegradation assays, the bacterium that previously showed the best growth in the presence of CYP was selected. The bacterium was reactivated under the above-mentioned conditions. From this seed flask, an inoculum of 3 × 106 cells/mL was collected by triplicate and added to a flask containing 30 mL of MSM supplemented with CYP (100 mg/L). The culture was monitored, and aliquots of 1.0 mL were drawn at different times (0, 6, 12, 24, 36, 48, 56, 72, 96, 120, 140, 180, and 360 h) to determine the percentage of CYP biodegradation. Biodegradation was calculated from a standard curve with different CYP concentrations (5, 20, 30, 40, 50, 60, 80, and 100 mg/L). The results were read by triplicate in a spectrophotometer (UV-Vis Thermo Scientific Genesys 10S) at a λ = 210 nm and plotted by simple linear regression.
Degradation products extraction
Thirteen samples were obtained by triplicate from the different times, placed in Falcon tubes (Corning), and vortexed for 3 min. Subsequently, they were centrifuged at 14 000 rpm for 30 min and the supernatant was recovered. Metabolites were extracted by the liquid-liquid technique with ethyl acetate 2:1 (v/v). The organic phase was recovered, and the solvent was removed under reduced pressure at 40 ºC. The obtained product was resuspended in 5 mL of methanol. This solution was filtered with a 0.22 µm pore membrane (Millex GP, Millipore Express PES membrane). The filtrate was used for substrate analysis by ultra-performance liquid chromatography-mass spectrometry (UPLC-MS).
Analysis by UPLC-MS
Samples were analyzed at the Centro de Biotecnología Genómica of the Instituto Politécnico Nacional (Genomic Biotechnology Center of the National Polytechnic Institute). The UPLC-MS analysis was performed using an Acquity UPLC system coupled to a Waters QDA mass detector column (Milford, MA): Acquity UPLC Cortecs C18 1.6 µm, 3.0 × 100 mm. The injection volume was 1 µL at a flow rate of 0.5 mL/min. The column temperature was maintained at 40 ºC during the analysis, using a gradient elution with a mobile phase of acetonitrile and 0.1 % formic acid in water at a ratio of 30:70. The total run time for samples with CYP was 8 min. Data acquisition, data management, and instrument control were performed using Empower software.
RESULTS
Isolation and identification
In this work, bacteria from soil samples of bean and corn crops from Fresnillo City, Zacatecas, Mexico were obtained. Six bacterial strains were morphologically identified from a primary culture (soil inoculated in MSM with CYP) and subsequently transferred to an enriched medium supplemented with 100 mg/L of CYP. Two strains were isolated from corn soil and four from bean soil. Molecular identification by 16S rDNA sequencing showed that the bacteria belonged to the genera Acinetobacter, Serratia, Pseudomonas, and Klebsiella (Table I), in particular two strains of the genus Klebsiella and two strains of the genus Pseudomonas.
TABLE I BACTERIA ISOLATED FROM CORN AND BEAN CROP SOILS WITH ADDED CYPERMETHRIN MEDIA AND IDENTIFIED BY 16S RDNA SEQUENCING.
Code | Crop | Bacteria | Access number |
FP2 | Bean | Acinetobacter calcoaceticus | AB680365.1 |
FP1 | Bean | Klebsiella pneumoniae (YH43) | AP014950.1 |
MP2 | Corn | Klebsiella pneumoniae (KP-1) | CP012883.1 |
FP4 | Bean | Pseudomonas plecoglossicida (NyZ12) | KY646089.1 |
MP4 | Corn | Pseudomonas putida (n7) | JQ782496.1 |
FP5 | Bean | Serratia marcescens | KX928068.1 |
Growth in the presence of CYP as a carbon source
To select the most tolerant strain with the best growth, the six identified strains were inoculated in MSM with CYP at 100 mg/L as the maximum concentration. Figure 2 shows the growth behavior of each bacterium after 180 h of culture. The best adapted genus was Klebsiella. K. pneumoniae YH43 showed the best adaptation using CYP as the only carbon source, with a greater exponential growth in the experiment. K pneumoniae KP-1 and S. marcescens spp. also showed an exponential growth, but lower than the latter. On the contrary, P. plecoglossicida NyZ12, A. calcoaceticus, and P. putida n7 showed a lower growth in all the evaluated times.
CYP degradation analysis
The percentage of CYP degradation by K. pneumoniae YH43 in a liquid medium (pH 7.0) at 35 ºC was calculated from the equation of the straight line generated from the standard curve (Fig. 3). The OD of each flask with MSM and CYP (100 mg/L) determined at different times, was substituted into the equation to determine changes in CYP concentration. CYP degradation by K. pneumoniae YH43 was 87.55 % at 360 h of culture.
UPLC-MS analysis
Finally, an UPLC-MS analysis was done to know the potential metabolites of the CYP degradation by K. pneumoniae YH43. Initially, the CYP standard at time 0 h was identified at a time of 2.94 min with a molecular weight of 401.21 (Fig. 4A) and different metabolites previously reported were detected (Table II). After 360 h, the metabolites detected were methyl-3-(2,2-dichlorovinyl)-2-2-dimethylcyclopropane-1-carboxylate; methyl-3-(2,2-dichlorovinyl)-2-(hydroxymethyl)-2-methylcyclopropane-1-carboxylate; 3-(2,2-dichlorovinyl)-2-hydroxymethyl)-2-methylcyclopropane-1-carboxylic acid; methyl-3-(2,2-dichlorovinyl)-2-(hydroxymethyl)-2-methylcyclopropane-1-carboxylate; 3-phenoxybenzyl alcohol, and 3-phenoxybenzoic acid (3-PBA; Fig. 4B and 5).

Fig. 4 Ultra-performance liquid chromatography-mass spectrometry (UPLC-MS) analysis. (A) Chromatogram of cypermethrin at time 0 h; (B) metabolites produced by K. pneumoniae YH43 after 360 h. a: methyl-3-(2,2-dichlorovinyl)-2-2-dimethylcyclopropane-1-carboxylate; b: methyl-3-(2,2-dichlorovinyl)-2-(hydroxymethyl)-2-methylciclopropane-1-carboxilate; c: 3-(2,2-dichlorovinyl)-2-hydroxymethyl)-2-methylcyclopropane-1-carboxylic acid; d: methyl-3-(2,2-dichlorovinyl)-2-(hydroxymethyl)-2-methylciclopropane-1-carboxilate; e: 3-p henoxybenzyl alcohol; and f: 3-phenoxibenzoic acid (3-PBA).
TABLE II METABOLITES IDENTIFIED BY ULTRA-PERFORMANCE LIQUID CHROMATOGRAPHY-MASS SPECTROMETRY (UPLC-MS) OF α-CYPERMETHRIN (100 mg/L) DEGRADATION BY K. pneumoniae YH43.
Metabolites | Mw reported m/z | Time 96 h | Time 360 h | % Identity | ||
Mw founded m/z | Rt (min) | Mw founded m/z | Rt (min) | |||
Cypermetrine standard | 416.30 | 401.21 | 2.938 | 401.22 | 2.930 | 100 |
Alfa-cypermetrine | 416.3 | 415.13 | 1.36 | 415.16 | 1.367 | 98 |
methylcyclopropane-1-carboxylic acid (cis-DCCA) | 209.07 | ND | ND | ND | ND | ND |
3-phenoxibenzoic acid (3-PBA) | 214.22 | 214.26 | 1.578 | 214.31 | 1.550 | 99 |
I cypermetrine | 430.41 | 432 | 1.334 | 432 | 0.710 | 96 |
3-phenoxybenzyl-3-(2,2-dichlorovinyl)-2,2-dimethylcyclopropane-1-carboxylate | 404.39 | ND | ND | 402 | 0.760 | 96 |
2-(aminooxy)-2-oxo-1-(3-phenoxyphenyl) ethyl-3-(2,2-dichlorovinyl)-2,2-dimethylciclopropane-1-carboxilate | 449.43 | 449.73 | 5.553 | ND | ND | 99 |
2-(methoxy)-2-oxo-1-(3-phenoxyphenyl) ethyl-3-(2,2-dichlorovinyl)-2,2-dimethylcyclopropane-1-carboxylate | 464.44 | 464.35 | 0.710 | 464 | 0.660 | 99 |
Cyano (3-(4-methoxyphenoxy) phenyl) methyl-3-(2,2-dichlorovinyl)-2,2-dimethylcyclopropane-1 carboxylate | 462.45 | 462 | 4.398 | 462 | 3.390 | 99 |
(3- phenoxyphenyl) methanol | 210.31 | ND | ND | 209 | 2.93 | 98 |
Methyl-3-pheenoxybenzoate | 238.32 | 238.04 | 1.31 | 238.03 | 1.295 | 99 |
3-(4-hydroxyphenoxy) benzoic acid | 240.29 | 241.30 | 0.651 | ND | ND | 98 |
Methyl-3-(4-hydroxyphenoxy) benzoate | 255.33 | 256.34 | 3.4 | 256 | 3.355 | 98 |
Methyl-3-(4-methoxiphenoxy) benzoate | 270.36 | 269.26 | 2.30 | 269.15 | 2.44 | 98 |
3-(2,2-dichlorovinyl)2,2-dimethylcyclopropane-1-carboxylic acid | 215.11 | 214.26 | 1.626 | 214.31 | 1.557 | 98 |
Methyl-3-(2,2-dichlorovinyl)-2-2-dimethylcyclopropane-1-carboxylate | 230.15 | 230.26 | 1.595 | 230.25 | 1.35 | 99 |
3-(2,2-dichlorovinyl)-1-methylcyclopropane-1,2-dicarboxylic acid | 245.10 | 247.62 | 1.373 | 247.60 | 1.367 | 96 |
Dimethyl-3-(2,2-dichlorovinyl)-1-methylcyclopropane-1,2-dicarboxylate | 275.17 | ND | ND | 275.07 | 0.833 | 99 |
3-(2,2-dichlorovinyl)-2-hydroxymethyl)-2-methylcyclopropane-1-carboxylic acid | 232.12 | ND | ND | 233.12 | 1.343 | 98 |
Methyl-3-(2,2-dichlorovinyl)-2-(hydroxymethyl)-2-methylciclopropane-1-carboxilate | 247.16 | 247.15 | 2.456 | 247.20 | 2.461 | 99 |
Cyano (3-(4-hydroxyphenoxy) phenyl) methyl-3-(2,2-dichlorovinyl)-2,2-dimethylcyclopropane-1 carboxilate | 447.42 | ND | ND | 447 | 0.789 | 99 |
3-phenoxybenzaldehyde | 198.26 | ND | ND | ND | ND | ND |
Dichloroethenyl, dimethylcyclopropane carboxylic acid | 104.53 | ND | ND | ND | ND | ND |
3-phenoxybenzyl alcohol | 199.0 | ND | ND | 200 | 1.462 | 98 |
(RS)- alpha-cyano-3-phenoxybenzyl alcohol | 225.5 | 225.21 | 1.140 | 225 | 1.137 | 99 |
(1RS)- cis, trans-3- (2,2-dimethylcyclopropanecarboxylate | 208.06 | ND | ND | 208 | 6.991 | 99 |
3-phenyl benzoate | 213.21 | ND | ND | 214.22 | 1.557 | 98 |
3,4-Dihydroxybenzoate | 153.11 | 150.02 | 0.675 | 150.01 | 0.678 | 96 |
Mw: molecular weight, Rt: retention time, ND: not detected.
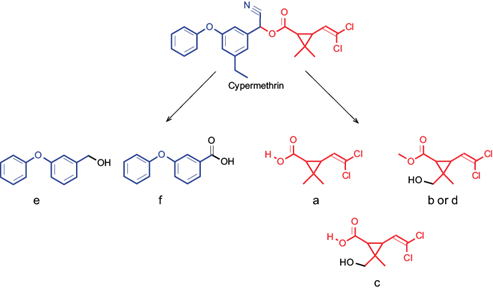
Fig. 5 Metabolites detected in the pathway of cypermethrin degradation by the K. pneumoniae YH43 bacteria. (a) Methyl-3-(2,2-dichlorovinyl)-2-2-dimethylcyclopropane-1-carboxylate; (b) methyl-3-(2,2-dichlorovinyl)-2-(hydroxymethyl)-2-methylciclopropane-1-carboxilate; (c) 3-(2,2-dichlorovinyl)-2-hydroxymethyl)-2-methylcyclopropane-1-carboxylic acid; (d) methyl-3-(2,2-dichlorovinyl)-2-(hydroxymethyl)-2-methylciclopropane-1-carboxilate; (e) 3-phenoxybenzyl alcohol; and (f) 3-phenoxibenzoic acid (3-PBA).
DISCUSSION
In this work, six bacteria from pesticide contaminated soil were identified as agents capable to tolerate CYP. The presence of CYP in the medium caused a selective effect on the growth of the bacteria. Our findings are consistent with previous studies of strains resistant to pesticides isolated from soil and water samples contaminated from areas of high agricultural activity (Huang et al. 2018).
Commonly, K. pneumoniae is one of the most dangerous species to health, with high virulence and antibiotic resistance. In this study, the 16S rDNA gene sequence analysis identified FP1 and MP2 isolated as K. pneumoniae strains. Strain K. pneumoniae YH43 has been isolated and identified from potato crop soil samples and used as a model to study nitrogen fixation (Iwase et al. 2016). Its presence in this type of source could be due to its adaptation to soil conditions where CYP is commonly applied (Nyawade et al. 2020).
On the other hand, two strains of the genus Pseudomonas (P. plecoglossicida NyZ12 and P. putida n7) were identified. Several studies show that both strains are capable of catabolizing aromatic compounds from industrial chemicals and agrochemicals (Wongsa et al. 2004, Chetverikov et al. 2017, Nogales et al. 2017, Yan et al. 2017). In this study, both strains only tolerated CYP.
A. calcoaceticus was also identified in this study. A previous study showed that A. calcoaceticus can use the insecticide chlorpyrifos as sole carbon source for their growth (Zhao et al. 2014). Additionally, A. calcoaceticus can grow in the presence of polycyclic aromatic hydrocarbons such as fluorene and phenanthrene, as well as heavy metals such as copper and cadmium (Liu et al. 2016, Méndez et al. 2017). However, in this study A. calcoaceticus only tolerated CYP. The isolate FP5, identified as S. marcescens, can degrade compounds such as the herbicide nicosulfuron (Zhang et al. 2012) and hexachlorobutadiene. Therefore, S. marcescens is considered as useful for the bioremediation of contaminated environments (Li et al. 2008). In our study it only tolerated CYP.
K. pneumoniae YH43, K. pneumoniae KP-1, and S. marcescens showed exponential growth using CYP as the only carbon source. The rest of the strains showed atypical growth during the experiment, which suggests that these bacteria are tolerant to concentrations of CYP lower than 100 mg/L and possibly require more culture time to adapt to the CYP concentration tested. Strain K. pneumoniae YH43 showed the best growth; therefore, it was selected for the biodegradation assays. In this work, K. pneumoniae YH43 caused an 87.55 % degradation of CYP, in contrast to the findings of Tang et al. (2019), who reported that under similar conditions strain K. pneumoniae BPBA052 degraded 45.42 % of β-cypermethrin at a concentration of 100 mg/L over a period of 48 h.
According to the metabolites of CYP degradation by strain K. pneumoniae YH43 identified by UPLC-MS, the results suggest that the degradation occurred through ester bond hydrolysis, which agrees with the findings of Tallur et al. (2008) for A. calcoaceticus, Sphingomonas sp., and Micrococcus sp. This pathway is considered one of the main routes for CYP degradation (Yao et al. 2015, Huang et al. 2018). Methyl-3-(2,2-dichlorovinyl)-2-2-dimethylcyclopropane-1-carboxylate is a key metabolite in the degradation pathway that causes breaking of the ester bond and loss of insecticidal activity. Additionally, the compounds 3-phenoxybenzyl alcohol and 3-phenoxybenzoic acid (3-PBA) are described as end products of the CYP degradation pathway that can be further mineralized under natural environmental conditions to products such as gallic acid, catechol, and protocatechuic acid (Huang et al. 2018).
CONCLUSION
In this study, K. pneumoniae YH43 was isolated and identified as a microorganism capable of growing in MSM using CYP as a carbon source. This strain has the ability to cause an 87.55 % degradation of CYP. Additionally, two compounds were identified as main products of the CYP degradation: α-cyano-3-phenoxybenzyl alcohol and cis,trans-3-(2,2-dichlorovinyl)-2,2-dimethylcyclopropane carboxylate, suggesting an ester bond hydrolysis. These findings suggest that K. pneumoniae YH43 can be used in the bioremediation of CYP contaminated soils.