INTRODUCTION
Chromium is used in diverse metal products and processes. Some of the main uses of chromium compounds are (a) plastic coatings, (b) electroplating of metals as anti-corrosion agents, (c) leather tanning and finishing, and (d) in pigments and wood preservatives (Mohan et al. 2006). Chromium reaches the environment via leakage, poor storage, or unsafe disposal practices. Naturally occurring chromium is mainly the result of dissolved minerals from weathering of chromites and other chromium bearing minerals present in bedrock and soil (Nriagu et al. 1988). Chromium occurs in wastewater resulting from the previously mentioned processes in both trivalent and hexavalent forms. Chromium (III) is nontoxic when present in trace level but it is mutagenic in 6+ state. Though Cr (III) is not toxic, it can be oxidized to be in toxic form, chromium (VI), in natural waters (Kannan 1995, Ahmed et al. 2013). Cr (VI) is carcinogenic and toxic. The major toxic effects of Cr (VI) are chronic ulcers, dermatitis, corrosive reaction in nasal septum and local effects in lungs (Ghosh 2009). Hexavalent chromium is considered as one of the top 16 toxic pollutants because of its carcinogenic and teratogenic characteristics (Bansal et al. 2009). Effluents from tanning, electroplating, paint and textile industries contain higher hexavalent chromium concentration than the maximum allowable contaminant level (Siboni et al. 2011).
The tolerable levels of hexavalent chromium into the inland surface water and potable water are 0.1 mg/L and 0.05 mg/L respectively (Hsu et al. 2009). To protect the public health, Cr (VI) must be removed from the industrial effluents before discharging into the environment. Using traditional treatment methods, Cr (III) can be treated by precipitating the hydroxide salt at a pH of 9. Traditional treatment techniques are much more complex when treating waste with Cr (VI) or “hex chrome.” It must be reduced to Cr (III) before it can precipitate. The reduction process is usually done at a pH of 3 with sodium bisulfite as the reducing agent, although other reducing agents such as ferrous ion can also be used. Once the chrome is reduced, it can be precipitated as the hydroxide at a pH of 8 to 9 (Nethaji et al. 2013). Adsorption of chromium on different sorbents such as iron, iron oxide, iron coated sand, and iron coated activated carbon (Petrusevski et al. 2002), and granular ferric hydroxides (Driehaus and Jekel 1998) have also been investigated. Naturally occurring ores and minerals, namely kaolinite (Guhab and Chaudhuri 1998), magnetite (Shipley et al. 2009), hematite and maghemite (Tuutijarvi et al. 2009) have also been used for the adsorption of some metal ions though not as extensively as other materials. According to Grosvenor et al. (2004), iron oxide films produced after short oxygen exposure times contain a mixture of magnetite (Fe3O4) and maghemite (g-Fe2O3). According to Hu et al. (2004), the metal ion adsorption by magnetite is a combination of electrostatic attraction and ligand exchange at various pH conditions.
The use of mentioned iron-based adsorbents is limited due to high operation cost, sludge formation or technical difficulties in preparation of materials. Recently magnetic separation emerged as a promising environmental purification technique since it produces no contaminants and has the ability to treat large amount of wastewater within a short span of time (Rocher et al. 2004). Since the magnetic particles are superparamagnetic (they are magnetized only at the external magnetic field), they can be recovered very quickly by external magnetic field and reused without losing the active sites (Inbaraj and Chen 2011).
The objective of the present study is to investigate the possible use of blast furnace dust (BFD), an steelmaking wastes, as an alternative adsorbent material for the removal of Cr (VI) from wastewater. Batch experiments are carried out for kinetic studies on the removal of Cr(VI) from aqueous solution. The influence of various important parameters such as time, adsorbent amount, and initial Cr (VI) concentration are investigated. Many kinetic models were studied and the Langmuir, Freundlich, Tempkin and Dubinin-Radushkevich models are used to fit the experimental equilibrium isotherm data obtained in this study.
EXPERIMENTAL PROCEDURE
Chromium removal tests
Two type of chromium solutions samples was used in the adsorption tests: First, synthetic solution (or synthetically prepared electroplating effluent) was prepared using a chromium (IV) atomic absorption standard solution (Aldrich, 1000 mg/L Cr in nitric acid) and softened tap water (free carbonates), Fe total = 1 mg/L and SO4 -2 = 200 mg/L. Chromium concentration from 1 to 12 mg/L was using to adsorption tests. Initial pH of 3 was fixed with HNO3 analytical grade reactive. At this pH, the most stable specie of chromium is Cr (VI) as HCrO4 - ions, according to the species distribution diagram showed in figure 1. Second solution samples were obtained from steel plating industry wastewater, containing 700 mg/L of chromium (as chromic acid), Fe total = 50 mg/L and SO4-2 = 400 mg/L, at pH = 3. Similarly, figure 1 indicates that stable chromium species in the wastewater solution is Cr (VI) as HCrO4 - ions at the solution pH.

Fig. 1 Chromium species distribution in aqueous solution. Modeling with medusa software for [Cr]solution = 12 mg/L, at 1 atm and 25 ºC
Batch tests were performed to remove chromium from the synthetic and electroplating effluent solutions. The batch experiments were carried out in 200 mL borosil conical bottles. A specific amount of BFD was added in 100 mL of aqueous Cr(VI) solution, and then stirred for a predetermined period (found out from the kinetic studies) at 25 ºC. Afterwards, the resultant solution was filtered using a filter paper. Test time, amount of BFD and chromium concentration parameters were studied in these experiments. Chromium determination in solutions after and before adsorption tests was carried out by atomic absorption spectroscopy (AAS, Perkin Elmer 3100).
The amount of Cr (VI) adsorbed by the sawdust and the percentage removal of Cr (VI) were calculated using the following equations (1) and (2), respectively:
where, qe is the adsorption capacity in mg/g, Ci, C0 and Ce are the initial, outlet and equilibrium concentration of Cr (VI) in mg/L, V is the volume of Cr (VI) solution in mL and W is the total amount of adsorbent in g.
BFD characterization
Industrial BFD samples were collected from a dust collector system installed in a steelmaking plant. The chemical and physical characteristics are detailed and discussed in previous work (Carrillo-Pedroza et al. 2014). A resume of the main characteristics is showed in table I. As can be seen in the table, BFD have a specific surface area value of 21.7 m2/g. This value is in the same order that the values reported in literature for different iron oxides, as showed in table II. On the other hand, the isoionic point (IIP) value of 5 for BFD indicated that the protonation of BFD surface is enhanced at pH lower than IIP (positive surface charge), while deprotonation is promoted at pH greater than IIP (negative surface charge). Comparing, literature reports IIP of 3.1 for blast furnace sludge (Lopez-Delgado et al. 1998), and pHZPC for magnetite of 6.4 to 6.7 (Mamindy-Pajany et al. 2011, Chowdhury et al. 2012), respectively. Finally, the values of the magnetic properties of BFD indicated in table I are similar at the values observed in magnetite mineral, soft ferrites and synthetic magnetite (Shipley et al. 2009, Ahmed et al. 2013, Nethaji et al. 2013). This feature is very important for some applications where post-adsorption magnetic recovery of these materials would be required.
RESULTS AND DISCUSSION
Adsorption kinetics
To understand the sorption mechanism of Cr (III) on BFD, the kinetic data were fitted into different models reported in literature (Badruddoza et al. 2013, Li et al. 2013, Nethaji et al. 2013, Oladoja et al. 2013). A brief description of the kinetics model is mentioned as follow.
The pseudo-first order kinetic model is based on the Lagergren equation, represented as:
where qt and q e (mg/g) are the amount of solute adsorbed at time t (min) and at equilibrium, respectively. The first order rate constant for kinetic model is k1 (min-1). The model describes the kinetic process of the liquid-solid phase adsorption.
The pseudo-second order kinetic model is described by the following equation,
In this equation, the driving force (q e -q t ) is proportional to the available fraction of actives sites and k 2 is the second order rate constant.
Intraparticle diffusion model assume that adsorption by internal diffusion or intraparticle diffusion is the rate-controlling step. This model is given by the equation (5):
where kid is the intraparticle diffusion rate constant. The plot q t against t1/2 represents the different steps of adsorption: external or film diffusion, intraparticle diffusion and adsorption in active sites or mass action. Then, the linear portion of the curve qt versus t1/2 represents the intraparticle diffusion. The slope yields the intraparticle diffusion rate constant kid and the intercepts reflects the boundary layer (BL) effect. The bigger the intercept, the greater is the contribution of the surface sorption to the rate-controlling step.
Finally, the Elovich equation. This model is used to described the kinetics of chemisorption of a gas. The equation is expressed as:
where b is the desorption constant and a the initial adsorption rate.
The results obtained from the different models are summarized in table III. It can be seen that the correlation coefficient of pseudo-second-order and intraparticle models (r2 = 0.997 and 0.984, respectively) are much higher than the other models. Therefore, that the adsorption kinetics follow intraparticle and pseudo-second-order models could suggest intra-particle diffusion with adsorption in active sites. In the initial stages of adsorption of Cr (VI), the concentration gradient between the film and the available pore sites is large, and hence the rate of adsorption of Cr (VI) is faster. The rapid adsorption of chromium by BFD can be attributed to external surface adsorption. It is easy for chromium access to active adsorption sites, thus resulting in a rapid uptake of chromium. The rate of adsorption decreases in the later stages of Cr (VI) adsorption probably due to the slow pore diffusion of the solute ion into the bulk of the adsorbent.
Adsorption isotherm study
In order to have a best understanding of the phenomenon of adsorption that occurs between chromium and BFD, different adsorption isotherm models were analyzed. The Langmuir, Freundlich, Tempkin and Dubinin-Radushkevich adsorption isotherm equations were used to interpret the nature of chromium adsorption on BFD samples. Table IV shows the isotherm parameter from chromium adsorption in synthetic and industrial plating wastewater solution samples, respectively. The table also shows the error functions used to define the best-fitting relationship (Foo and Hameed 2010). Error functions, as correlation coefficient (r2), sum square error (SEE), sum of absolute errors (SAE) and average relative error (ARE) are widely described in literature (Ng et al. 2003, Kumar and Sivanesan 2006, Karadag et al. 2007, Mane et al. 2007).
TABLE IV ISOTHERM PARAMETERS AND ERROR FUNCTIONS FROM THE SORPTION OF Cr (VI) ON BLAST FURNACE DUST FOR SYNTHETIC SOLUTION
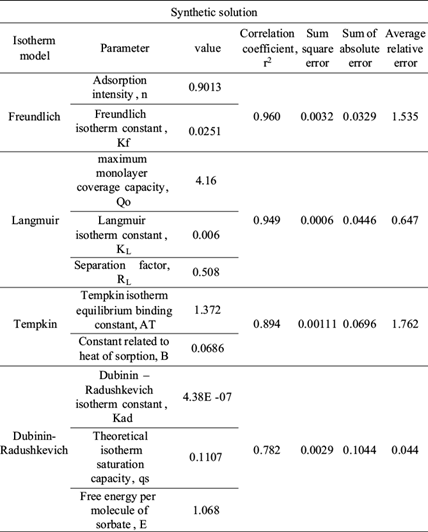
Figures 2 and 3 show the comparative curves of the isotherm models that have a major adjust to experimental data, according to error functions. The results and the isotherm models are described and analyzed below. Langmuir adsorption isotherm describes quantitatively the formation of a monolayer adsorbate on the outer surface of the adsorbent, and after that no further adsorption takes place (Langmuir 1916, De Boer 1968). Thereby, the Langmuir isotherm represents the equilibrium distribution of metal ions between the solid and liquid phases (Kundu and Gupta 2006, Vijayaraghavan et al. 2006). The Langmuir isotherm is valid for monolayer adsorption onto a surface containing a finite number of identical sites. The model assumes uniform energies of adsorption onto the surface and no transmigration of adsorbate in the plane of the surface (Demirbas et al. 2008). Based upon these assumptions, Langmuir isotherm is represented by the following equation:

Fig. 2 Plot of equilibrium experimental data and calculated equilibrium data using different isotherm models for the adsorption of Cr (VI) onto blast furnace dust (BFD), for synthetic solution with a initial Cr (VI) concentration of 5 mg/L, temperature 25 ºC, initial pH 3

Fig. 3 Plot of equilibrium experimental data and calculated equilibrium data using different isotherm model for the adsorption of Cr (VI) onto blast furnace dust, for industrial steel plating wastewater solution with a initial Cr(VI) concentration of 700 mg/L, temperature 25 ºC, initial pH 3
where:
Ce |
the equilibrium concentration of adsorbate (mg/L) |
qe |
the amount of metal adsorbed per gram of the adsorbent at equilibrium (mg/g) |
Q0 |
maximum monolayer coverage capacity (mg/g) |
KL |
Langmuir isotherm constant (L/mg) |
A dimensionless constant, commonly known as separation factor (RL) defined by Webber and Chakkravorti (1974) can be represented as:
where:
C0 |
initial concentration |
KL |
the constant related to the energy of adsorption (Langmuir Constant) |
RL value indicates the adsorption nature to be either unfavorable if RL > 1), linear if RL = 1, favorable if 0 < RL < 1 and irreversible if RL = 0. From the data calculated in table IV and table V, the RL value, 0.508, is greater than 0 but less than 1 indicating that Langmuir isotherm is propitious. In this work, for synthetic solutions, the maximum monolayer coverage capacity (Q0) from Langmuir isotherm model was determined to be 4.16 mg/g, KL is 0.006 L/mg and the r2 value is 0.956 proving that the sorption data fitted well to Langmuir isotherm model. In the case of industrial plating wastewater solutions, the Langmuir parameters values obtained were Q0 = 0.212 mg/g and KL = 0.0019 L/mg (r2 = 0.887) and RL = 1.51. This last value indicates an unfavorable or very limited adsorption. Possibly due to high concentration of sulphate and Fe ions in the wastewater solution.
TABLE V ISOTHERM PARAMETERS AND ERROR FUNCTIONS FROM THE SORPTION OF Cr (VI) ON BLAST FURNACE DUST FOR INDUSTRIAL STEEL PLATING WASTEWATER SOLUTION

Freundlich adsorption isotherm is commonly used to describe the adsorption characteristics of heterogeneous surfaces (Freundlich 1906, Hutson and Yang 2000). These data often fit the empirical equation proposed by Freundlich:
where
Kf |
Freundlich isotherm constant (mg/g) |
n |
adsorption intensity |
Ce |
the equilibrium concentration of adsorbate (mg/L) |
The constant Kf is an approximate indicator of adsorption capacity, while 1/n is a function of the strength of adsorption in the adsorption process. If n = 1, then the partition between the two phases is independent of the concentration. If value of 1/n is below one it indicates a normal adsorption. On the other hand, 1/n being above one indicates cooperative adsorption (Mohan and Karthikeyan 1997, Haghseresht and Lu 1998). From the data in tables IV and V, n = 0.9013, therefore 1/n = 1.11 for synthetic solutions (r2 = 0.966), while the value of n = 0.044 (1/n = 22.5) for industrial plating wastewater solution (r2 = 0.801). The r2 and 1/n values obtained in the adsorption of chromium in synthetic and industrial solution are indicative of a cooperative adsorption process.
The Tempkin isotherm contains a factor that explicitly takes into account adsorbent-adsorbate interactions. The model assumes that heat of adsorption (function of temperature) of all molecules in the layer would decrease linearly rather than logarithmic with coverage (Tempkin and Pyzhey 1940, Aharoni and Ungarish 1977). The model is given by the equation 10. From plotting the quantity sorbed qe vs ln Ce, the constants are determined from the slope and intercept.
where:
AT |
Tempkin isotherm equilibrium binding constant (L/g) |
b |
Tempkin isotherm constant |
R |
universal gas constant (8.314J/mol/K) |
T |
temperature at 298K |
B |
RT/b, constant related to heat of sorption (J/mol) |
From the Tempkin plot, the following values were estimated (Tables IV and V): AT = 1.372 L/g, B = 0.0686 KJ/mol (r2 = 0.894) and AT = 0.00213 L/g, B = 149.22 KJ/mol (r2 = 0.667), for synthetic and industrial plating wastewater, respectively. The low B value obtained in synthetic solution is an indication of the heat of sorption for a physical adsorption process. In the case of B value for industrial plating wastewater solution, this value cannot be considered representative due to the low r2 and the high SEE and SAE error function values.
The fourth isotherm model used in this work was the Dubinin-Radushkevich isotherm (Dubinin and Radushkevich 1947, Dubinin 1960). The model has been successfully fitted high solute activities and the intermediate range of concentrations data as well.
where:
qs |
theoretical isotherm saturation capacity (mg/g) |
Kad |
Dubinin-Radushkevich isotherm constant (mol2/kJ2) |
e |
-Radushkevich isotherm constant. |
The model is usually applied to distinguish the physical and chemical adsorption of metal ions with its mean free energy (E), per molecule of sorbate, which can be computed by the relationship (Hobson 1969):
where BDR is denoted as the isotherm constant. Meanwhile, the parameter e can be calculated as:
where R, T and Ce represent the gas constant (8.314 J/mol K), absolute temperature (K) and adsorbate equilibrium concentration (mg/L), respectively.
From the linearized form of equations (11) and (12), qs was determined as 0.1107 mg/g and the mean free energy as E = 1.068 KJ/mol (r2 = 0.871) for synthetic solution, whereas for industrial plating solution qs was determined as 13092 mg/g and the mean free energy to E = 0.001 KJ/mol (r2 = 0.895). In the case of industrial plating wastewater solutions, the experimental data fit better at Dubinin-Radushkevich isotherm model than the other models, as is indicated in three of four error functions showed in tables IV and V. Therefore, the low free energy value is well indicative of a physical adsorption process for the industrial plating wastewater solution.
As was mentioned, Cr (VI) is found as H2CrO4. In solution, Cr (VI) is found as CrO4 2-, HCrO4 - or Cr2O72-, depending on both pH of the medium and total Cr (VI) concentration. For industrial plating wastewater, at pH > 1, deprotonated forms of Cr (VI) are seen. Above pH 7 only CrO4 2- ions exist in solution throughout the concentration range. In the pH between 1 and 6, HCrO4 - is predominant (Chowdhury et al. 2012). The anion adsorption sites on the BFD are aquo-groups (-Fe- OH2 +) and hydroxide groups (Fe-OH). The surface chemistry of such sorbents, in contact with an aqueous solution is determined, to a large extent, by deprotonation or a hydroxyl association reaction, according to the following reactions:
The reaction represented in equation 14 occurs preferably at a low pH, while the reaction indicated by equation 15 occurs at a higher pH range. In our case (pH = 3), anion HCrO4- can be absorbed by the positive charged surface due to the product reaction of equation (14). Then, iron zero valent-magnetite-wustite particles from BFD may adsorb either negatively or positively charged species by electrostatic attraction depending on pH of the solution. From the measured zeta potential of BFD at different pH values, the isoelectric point (IEP) value of the used material was 5. Then, at low pH (synthetic and industrial solutions pH = 3), the iron phases observed in table II react with the chromium acid solution to form FeOH+ in the BFD particle surface, according to the following reactions, where Gibbs free energies were calculated from HSC Chemistry software V5.1 (Roine 2002):
The positively charged BFD surface attracts and reacts with the HCrO4 - ions to form Cr2FeO4, according to:
Then, the sorption of chromium (VI) using BFD is first governed by diffusion followed by surface compound formation (in accordance to equation 19). According to Ho and McKay (1999) review, the pseudo-first order rate equation of Lagergren can be used as a rate equation in adsorption kinetics as a chemical phenomenon and a diffusion equation for diffusion through a boundary liquid film.
The results shows that BFD adsorbent removes up to 50 % and 35 % of chromium in synthetic electroplating solution and industrial plating wastewater solution, respectively. It may be due to pH and interference of other ions present in solutions (not determined in the present study). According to Dakiky et al. (2002) and Bhattacharya et al. (2008), increasing the pH will shift the concentration of HCrO4 - to Cr2O7 2− and other forms as CrO4 -. Maximum adsorption at pH below 3 indicates that it was the HCrO4 - form of Cr (VI), the predominant species at this pH range and adsorbed preferentially on the adsorbent. Better adsorption capacity observed at pH 3 may be attributed to the large number of H+ ions present at this pH value, which in turn neutralize the negatively charged hydroxyl groups (OH-) on adsorbed surface thereby reducing the hindrance to the diffusion of dichromate ions. On the other hand, some studies indicate that various kinds of anions in industrial and groundwater, such as chloride, sulfate and nitrate, may lead to competitive adsorption (Badruddoza et al. 2013). As per the results reported by Babu and Gupta (2008) and Gupta and Babu (2009), the percentage removal of Cr (VI) is significantly affected by the presence of SO4 -2 in the industrial effluent. In their studies, synthetic solution with chemical composition such to the electroplating industry and the tannery industry effluents were treated with the sawdust, which resulted in a decrease in the percentage removal from 96 % to 55.4 % and 96% to 50.25 % respectively. Their results show that the adsorbent capacity for Cr (VI) removal is decreased approximately by 50 % for the synthetic electroplating and tannery effluents. These values are very similar at present study. Then, the high SO4 -2 content in plating solutions decrease the Cr (IV) remove due at competitive ion adsorption by electrostatic attraction between the negatively charged species by in the positive charged BFD surface, according to the following reaction:
Then, the reducing of H+ ions to form FeOH+ (equations 16 and 17) and the presence of sulphate ions could be decreasing the diffusion of dichromate ions to BFD particle surface, explaining the low adsorption observed in the results.
However, in spite of the results in terms of adsorption efficiency, the adsorption capacity obtained (0.14 mg/g for a 5 mg/L Cr (VI)) synthetic solution, and 18 mg/g for 515 mg/L Cr (VI) industrial solution) is comparable with some of the low cost adsorbents reported in the literature, which are indicated in table VI. Considering that BFD is a material that is obtained in the same integrated steelmaking industry where plating wastewater is also generated, the high availability of BFD makes this material the most attractive low cost adsorbent for these type of industry.
CONCLUSIONS
Crm(VI) from industrial wastewater streams is investigated. BFD is found to be a optional adsorbent for the removal of Cr (VI) as compared to many other low-cost and commercially available adsorbents. According to kinetic study, the rapid adsorption of chromium by BFD can be attributed to the external surface adsorption; after, adsorption decrease probably due to the slow pore diffusion of the solute ion into the bulk of the adsorbent. The equilibrium adsorption data were best fitted (according different error functions) with Freundlich isotherm model and Dubinin-Radushkevich for synthetic solution and industrial plating wastewater, respectively. This results confirms the physical adsorption of Cr (VI) onto the BFD. The presence of other ions such as SO4 2 in aqueous solutions are found to have significant effect on Cr (VI) adsorption. However, the low percentage value of 35 % for adsorption of Cr (VI) in industrial plating wastewater can be sufficient to make significant savings in the actually most common process of removal of chromium such as is the reduction-precipitation. Additionally, the low cost of BFD and their magnetic properties (to facilitate its recovery), expands the scope of their possible applications as complementary Cr (VI) remove method in the industrial plating wastewater treatment process.