Introduction
The anthracnose caused by Colletotrichum spp. is one of the most destructive crop diseases worldwide (Meng et al., 2020) and in the guarana plant (Paullinia cupana var. sorbilis) caused by Colletotrichum guaranicola Albuquerque, is one of the main limiting factors for the expansion of the production of guarana in the State of Amazonas, Brazil (Silva et al., 2018). The lifestyle patterns of Colletotrichum species are highly regulated by specific gene families and biochemical interactions that occur through specific enzymes and secondary metabolites produced at the host-pathogen interface (De Silva et al., 2017).
Pathogenicity is due to the ability of the microorganism to produce extracellular enzymes that can modify the cellular components present in the plant tissues (Armesto et al., 2019; Parveen et al., 2017). Phytopathogenic fungi secrete a variety of enzymes such as cutinases, pectinases, hemicellulases and cellulases, which will act in the degradation of the plant cuticle and the cell wall, colonization, and consequently in the onset of symptoms (Armesto et al., 2019). Along with hydrolytic enzymes, fungal oxidases such as polyphenol oxidase (PPO), which is recognized to be involved in necrotic lesions, might play an important role in this process (Boeckx et al., 2015).
Comparative studies of the enzymatic activity of pathogenic and non-pathogenic C. guaranicola and its relationship with the infection process facilitate the understanding of the enzymatic mechanisms related to pathogenicity and could provide information for the establishment of pathogen management strategies and knowledge about the pathogen-host interaction. Based on this, the present study aimed to evaluate the enzymatic activity and the initial infection process of endophytic and pathogenic strains of Colletotrichum guaranicola in the guarana plant.
Material and methods
Fungal strains
Fourteen pathogenic and nine endophytic strains were evaluated. The fungal strains were obtained from guarana leaves collected in fields at the municipalities of Manaus, Maués, Presidente Figueiredo and Rio Preto da Eva (State of Amazonas, Brazil) (Table 1). For the isolation of endophytes, healthy and undamaged guarana leaves were used according to Petrini (1996). The pathogenic strains were obtained from leaves with typical anthracnose symptoms. The indirect isolation was performed on potato dextrose agar medium (PDA, Difco) at 27 °C (Alfenas and Mafia, 2007). The identification of the strains was carried out using morphological characters of 100 conidia and 100 appressoria of each strain and was compared with descriptions in literature (Albuquerque, 1960; Bentes and Barreto, 2004).
Table 1 Enzymatic activity of Colletotrichum guaranicola strains by diffusion in specific solid substrates
Strain (sample origin) |
Enzymatic activity [Degradation halo (mm)] | |||||
Lipase | Amylase | Polyphenol oxidase |
Cellulase | Pectinase | Protease | |
Pathogenic | ||||||
I 01 (1)† | 0.00 c | 3.63 d | 0.96 a | 0.26 a | 0.33 a | 0.30 a |
I 02 (1) | 0.00 c | 4.96 a | 0.83 a | 0.05 c | 0.23 b | 0.24 a |
I 04 (2) | 0.32 b | 4.56 b | 0.00 d | 0.03 c | 0.43 a | 0.32 a |
I 06 (2) | 0.57 a | 4.70 b | 0.43 b | 0.05 c | 0.23 b | 0.36 a |
I 07 (1) | 0.00 c | 3.96 c | 0.20 c | 0.10 b | 0.23 b | 0.33 a |
I 08 (3) | 0.28 b | 4.23 c | 0.00 d | 0.00 d | 0.26 b | 0.27 a |
I 09 (3) | 0.00 c | 3.63 d | 0.00 d | 0.00 d | 0.30 b | 0.21 a |
I 10 (3) | 0.00 c | 4.76 b | 0.00 d | 0.00 d | 0.43 a | 0.17 a |
I 11 (3) | 0.32 b | 5.20 a | 0.21 c | 0.00 d | 0.10 b | 0.30 a |
I 12 (3) | 0.26 b | 5.00 a | 0.43 b | 0.00 d | 0.10 b | 0.28 a |
I 461 (1) | 0.00 c | 4.60 b | 0.55 b | 0.00 d | 0.20 b | 0.25 a |
P11 (4) | 0.30 b | 5.00 a | 0.81 a | 0.05 c | 0.40 a | 0.26 a |
P17 (4) | 0.00 c | 4.96 a | 0.00 d | 0.00 d | 0.30 b | 0.20 a |
P18 (4) | 0.50 a | 5.26 a | 0.00 d | 0.00 d | 0.30 b | 0.23 a |
Endophytic | ||||||
I 13 (1) | 0.00 c | 5.13 a | 0.00 d | 0.06 b | 0.16 b | 0.34 a |
I 16 (4) | 0.34 b | 4.36 c | 0.26 c | 0.00 d | 0.36 a | 0.20 a |
I 17 (4) | 0.30 b | 4.00 c | 0.43 b | 0.00 d | 0.16 b | 0.17 a |
P 01 (3) | 0.41 b | 4.70 b | 0.33 c | 0.06 b | 0.16 b | 0.28 a |
P 07 (4) | 0.53 a | 4.36 c | 0.28 c | 0.05 c | 0.23 b | 0.32 a |
P 09 (4) | 0.00 c | 4.16 c | 0.00 d | 0.08 b | 0.20 b | 0.26 a |
P 10 (3) | 0.27 b | 4.10 c | 0.45 b | 0.05 c | 0.56 a | 0.24 a |
P 12 (3) | 0.41 b | 4.73 b | 0.76 a | 0.03 c | 0.36 a | 0.27 a |
P 13 (3) | 0.00 c | 4.03 c | 0.00 d | 0.00 d | 0.26 b | 0.21 a |
CV (%) | 5.09 | 2.35 | 8.22 | 2.07 | 7.71 | 5.48 |
Means followed by the same letter in the same column do not differ from each other (Scott-Knott test, P ≤ 0.05). CV: coefficient of variation. †Sample origin: (1) Manaus, (2) Rio Preto da Eva, (3) Presidente Figueiredo, (4) Maués.
Enzyme assays
Protease, amylase, and pectinase of endophytic and pathogenic strains were evaluated according to the protocol described by Valente et al. (2002) with some adaptations for pectinolytic assays. In this case, the analysis was performed with the substitution of CTAB by Congo red 0.05 % (Sigma-Aldrich). PPO and lipolytic activities were assessed according to the protocol of Trigiano et al. (2016). Cellulolytic activity was determined according to the protocol of Nogueira and Cavalcanti (1996).
Protease activity was evaluated using a culture medium containing skimmed milk in its constitution [0.25 g KH2PO4, 0.125 g KCl 0.05 g MgSO4 ·7H2O, 0.025 g CaCl2, 6.25 mL 22.5 % skimmed milk (Parmalat), 2.5 g glucose, 250 mL distilled water; 3 g agar; pH 5.4]. The observation of the presence of lighter concentric halos around the colony was an indication of the extracellular protease activity.
Amylase production was detected on medium containing soluble starch (0.25 g soluble starch, 0.5 g yeast extract, 0.25 g K2HPO4, 0.125 g MgSO4 ·7H2O, 25 μg thiamine-HCl, 4.25 g agar, and 0.25 mL of the micronutrient solution composed of 0.127 g FeNH4 (SO4)2 ·12H2O, 0.178 g ZnSO4·7H2O, 0.074 g MnSO4 ·4H2O, 0.008 g CuSO4, 0.01 g CoSO4, 0.01 g H3BO, 100 mL of distilled water, pH 7.3 ± 0.2); after incubation, the plates were flooded with iodine solution.
Pectinase activity was determined by cultivating the fungi in pectin agar medium [0.25 g (NH4)2SO4, 0.5 g KH2PO4, 0.75 g Na2HPO4, 0.025 g FeSO4 ·7H2O, 0.13 mg CaCl2 ·2H2O, 125 mL distilled water, 2.94 g sodium citrate, 3 g agar, 1.25 g pectin, pH 4.5]. To detect pectinase production the fungal culture plates were flooded with a 0.05 % Congo red solution (2.5 g L-1) supplemented with 0.1 M Tris-HCl buffer pH 8.0 for 30 min at room temperature (25 ± 2 °C). The solution was then discarded and the activity detected by the formation of a light halo surrounded by a red zone.
PPO activity was determined using gallic acid as substrate (1000 mL distilled water, 15 g malt extract, 20 g agar and 5 g gallic acid). PPO production was observed after 24 and 48 hours; its evaluation was made by measuring the halo around the colonies.
The lipase production was evaluated in culture medium using Tween 20 as substrate (10 mL tween-20-sorbitol monolaurate-, 8 g peptone, 0.1 g CaCl2 ·H2O, 20 g agar, 990 mL distilled water). After the incubation period, the lipase activity was observed by the presence of a white flocculent precipitate visible underneath and in front of the mycelial layer, where calcium crystals formed.
Cellulolytic activity was evaluated using a medium supplemented with the soluble carboxymethylcellulose (CMC) (Synth) as the sole source of carbon [3.0 g NaNO3, 1.0 g (NH4)2SO4, 0.5 g MgSO4, 0.5 g KCl, 0.01 g FeSO4 ·7H2O, 10 g CMC, 20.0 g agar, 1000 mL distilled water]. Plates containing 5-mm diameter mycelium discs were incubated for 4 d at 28 °C. The samples were then subjected to heat shock for 16 h at 50 °C. Plates containing fungal cultures were flooded with 10 mL of Congo red solution (0.05 %) supplemented with 0.1 M Tris-HCl buffer pH 8.0 for 30 min at 25 ± 2 °C. The solution was then discarded and each enzymatic activity was detected by the formation of a light halo surrounded by a red zone.
The experimental design was completely randomized with five replicates of the 23 fungal strains. PDA discs without fungi were deposited on Petri dishes with culture medium for each enzyme and used as control. A semi-quantitative evaluation was performed by measuring the degradation halo formed in each assay. The degradation halo values were statistically analyzed, and the means compared by the Scott-Knott test (P ≤ 0.05) using the ASSISTAT version 7.7 software (Silva and de Azevedo, 2016).
Infection process of endophytic and pathogenic Colletotrichum guaranicola
To detect differences in tissues pre-penetration and colonization that could be related to the pathogenicity mechanism, the initial infection process of pathogenic and endophytic C. guaranicola in leaves of a susceptible guarana clone was studied. To select strains of pathogenic and endophytic fungi, a pathogenicity test was carried out with the whole set of strains. Each fungus was cultured on PDA (Difco) medium for 10 d at 25 ± 2 °C. Healthy guarana seedlings of clone 300 were used. The inoculation was performed on young leaves in phenological stages 1 to 3, as previously described by Bentes and Matsuoka (2002).
Four 5-mm diameter fungal colony plugs were deposited on the adaxial side of leaves without lesions. Three leaves of each seedling were inoculated and kept in a humid chamber for 48 h. The infection was assessed for 7 d, based on the presence or absence of symptoms. Conidia germination and appressorium formation were quantified in 100 conidia for each strain, the leaves inoculated with pathogenic and endophytic strains of C. guaranicola were selected according to the results of the pathogenicity test.
The inoculated samples were collected at 12, 24, and 48 hours after inoculation (hai). After this period, symptoms were visible in plants inoculated with the pathogenic strain, evidencing the colonization of tissues. The collected samples were cut into 0.5 cm2 fragments and processed according to the methodology described by Longo et al. (1994). Conidia were observed in light microscope (Carl Zeiss, Jena, Germany) and photographed with the AxioCAM ERc 5s camera with a 40x objective.
The experimental design was completely randomized with four replicates; the experimental unit was one plant. The control consisted of plants that received PDA culture medium discs without fungal inoculum. Data were submitted to analysis of variance (ANOVA) and the Scott-Knott (P ≤ 0.05) using the ASSISTAT version 7.7 program (Silva and de Azevedo, 2016). Inoculated samples were collected at 48 hai and cut into pieces of 0.5 cm2. Samples were then fixed on a solution composed of 10 mL 35 % formaldehyde, 50 mL 95 % ethyl alcohol, 5 mL glacial acetic acid and 35 mL distilled water, for 48 h. Samples were preserved in 70 % ethyl alcohol aqueous solution for 48 h.
Samples were dehydrated in a series of aqueous ethanol solutions at 30, 50, 80 and 100 % (v/v) under vacuum. Dehydrated samples were then embedded into historesin (Leica) and successively submitted to alcohol and historesin at the following concentrations: 1:1 (v/v, 2 h), 1:3 (v/v, 2 h), neat historesin for 48 h. Embedded samples were cut on a rotary microtome (Leica RM 2145, Nussloch, Germany) into 6-μm thick sections. Histological sections were mounted under microscope slides and stained with 0.1 % toluidine blue. The slides were observed with a Carl Zeiss light microscope and photographed with the AxioCAM ERc 5s camera with a 40x objective.
Results and discussion
Enzymatic assay
The enzyme activities of C. guaranicola strains are presented in Table 1. All strains showed amylase activity with degradation halo ranging from 3.63 to 5.26 mm (Table 1). The largest halo degradations were observed within the pathogenic set and one endophytic strain (I 13) showing a halo of 5.13 mm. Similarly, Khodadadi et al. (2020) evaluated the production of extracellular enzymes of Colletotrichum isolates on starch agar plates and showed that all the species produced halo zones reflecting amylase activity after exposure to iodine.
According to Velho et al. (2018), most pathogens utilize starch to perform their metabolic activities, and the degradation occurs directly by the action of amylases. These enzymes promote starch degradation, which can likely be used as an energy source during growth and sporulation (Armesto et al., 2019).
The medium used for polyphenol oxidase had as main constituent gallic acid and the presence of a dark halo around colonies indicating PPO activity. Fourteen out of 23 strains (eight pathogenic and six endophytic) presented positive results for the assessed enzymes. The halo diameter ranged from 0.21 to 0.96 mm (Table 1), with no distinction between pathogenic and endophytic. Tozze Júnior et al. (2016), characterising the aggressiveness and enzymatic activity of Colletotrichum spp. associated with avocado (Persea americana) anthracnose, detected laccase activity in only 14 of the 30 isolates studied, with the distinction of six groups. According to the authors, there is evidence of the involvement of laccase in the activation of quiescent infections promoted by C. gloeosporioides in avocado during fruit ripening, in addition to the action of these enzymes in the detoxification of antifungal compounds and tannins present in the host.
According to Armesto et al. (2019), the greater expression of amylase and laccase activities in fungi may be related to their high needs for nutrients in the tissue colonization process. Amylase is directly related to energy requirement and laccase (polyphenol oxidase) with the penetration process.
Lipase activity was detected by the presence of a white precipitate below the mycelial layer, as a result of the formation of calcium salt crystals of the lauric acid released by the enzyme (Hankin and Anagnostakis, 1975). Strains I 06 and P18 (pathogenic), and P07 (endophytic) showed the greatest degradation halo for the lipase enzyme. For seven pathogenic and three endophytic strains (Table 1) degradation halos of lipase were not observed. Lipase represents an important enzyme complex in pathogenesis for decomposing host cellular components as phospholipids in the plasma membrane. The use of Tween 20 to screening fungi for lipase activity is a simple and appropriate method. However, oil substrate and their constitutive effect on extracellular lipase that varies from one to another organism. Lipase production can also be influenced by diverse factors such as carbon and nitrogen sources, pH in culture media, temperature, oxygen levels, and agitation (Geoffry and Achur, 2018).
In this study the differences observed on lipase production by pathogenic and endophytic isolates of C. guaranicola can be affected by intraspecific variations between isolates, as demonstrated by Armesto (2019) on C. gloeosporioides and coffee plants pathosystem, where the samples showed different enzymes profiles that may be associated to virulence.
Only for 12 strains (six endophytic and six pathogenic) showed cellulolytic enzyme production, with the formation of a small degradation halo (Table 1). Khodadadi et al. (2020) observed that all isolates belonging to C. noveboracense, C. fioriniae and C. chrysophilum showed cellulolytic activity after five days of incubation on PDA as a yellow halo around the colony in plates, including CMC stained with Congo red solution and NaCl. According to Acosta-Rodríguez et al. (2005), C. lindemuthianum was more efficient on medium supplemented with cellulose than on the CMC medium. In the first one, cellulolytic activity was detectable at 3 days of incubation, while after 13 days of growth in the CMC medium only about 35 % of the result was reached with cellulose.
Either the CMC medium could not have favoured the production of the enzyme or the evaluation time may not have been sufficient to verify the formation of the halo. Overall, these authors observed different cellulolytic enzyme production profiles between pathogenic and non-pathogenic C. lindemuthianum strains, suggesting that this enzyme has a role in the degradation of the plant cell wall during the infection onset. All the fungal strains assessed in the present study had positive results for pectinolytic enzymes, with halos ranging from 0.10 to 0.56 mm (Table 1). There were no differences between pathogenic and endophytic strains. High pectinolytic activity is likely related to pectin, which is a major plant component that could induce the fungus to produce a larger amount of these enzymes (Armesto et al., 2019). In the present study, the proteolytic enzyme was evident for all fungal strains. Degradation halos varied from 0.17 to 0.36 mm and there were no statistical differences between pathogenic and endophytic strains (Table 1).
Proteases are abundant in fungi, they generate peptides or amino acids to support fungal growth and contribute to degradation of the plant cell wall (González et al., 2016). Redman and Rodriguez (2002) reported a direct relationship between protease activity and virulence in Colletotrichum coccodes; according to the authors, extracellular protease activity was required for pathogenicity and virulence, and the elimination of protease activity transformed the virulent pathogen into a non-pathogen.
In the present study, pathogenic and endophytic strains were not distinguished based on the production of extracellular enzymes; both amount and rate of enzyme produced can interfere with the plant infection process resulting in success or failure in the establishment of the pathogen in the host.
Parveen et al. (2017) stated that differences in enzyme production by fungal pathogens can represent their virulence and specificity in causing diseases. Based on these data, it seems reasonable to suggest that these enzymatic activities should not be used as markers of virulence for these fungal isolates, considering that specific conditions in the host tissue, such as temperature and pH, are also important factors for regulation of gene expression and subsequent enzyme production (Velho et al., 2018).
Pre-penetration events
All pathogenic and endophytic strains were assessed for pathogenicity and strains were selected for the infection and colonization studies. None of the endophytic strains developed infection symptoms in the plant after 7 days of inoculation; however, in tomato (Solanum lycopersicum) infected with Colletotrichum coccodes, the infection symptoms appeared before 7 days after inoculation, mainly in fruits inoculated with artificial wound (Nieto-Angel et al., 2019).According to the statistical analysis, significant differences were observed in the injured area size caused by the pathogenic strains (Table 2).
Table 2 Injured area in guarana leaves inoculated with mycelial discs containing Colletotrichum guaranicola pathogenic strains.
Strain | Injury area (cm2) | Strain | Injury area (cm2) |
I 01 | 1.56 e | I 10 | 2.96 b |
I 02 | 2.40 c | I 11 | 2.26 c |
I 04 | 2.73 b | I 12 | 2.80 b |
I 06 | 3.53 a | I 461 | 1.90 d |
I 07 | 2.93b | P11 | 2.26 c |
I 08 | 2.53 c | P17 | 2.36 c |
I 09 | 2.26 c | P18 | 2.46 c |
CV % | 4.86 |
Means with the same letter do not differ from each other (Scott-Knott test, P ≤ 0.05). CV: coefficient of variation.
Brader et al. (2017) claim that commonalities and differences exist in colonization routes for both pathogenic and non-pathogenic endophytes. The pathogenic strain I 06, the most aggressive one observed in the present study, was selected along with the endophytic strain P10 for the study of initial infection and colonization process in guarana leaves. Symptoms were observed only on leaves inoculated with the pathogenic C. guaranicola strains after 48 hai. It appeared as small, reddish stains that indicate cell death. Fungal re-isolation corroborated the hypothesis that different endophytic C. guaranicola strains can stay in the endophytic association in guarana without manifesting symptoms. In addition, pathogenic strains induced symptoms appearance within 48 hai.
There was significant difference (Scott-Knott test, P ≤ 0.05) regarding germination and appressorium formation between endophytic and pathogenic strains (Figure 1A). Conidia germination was observed 12 hai for 84 and 66 % of the pathogenic and endophytic strains, respectively. Overall, pathogenic and endophytic strains reached 100 and 82 % germination respectively at 48 hai. Regarding appressoria formation, 76 % conidia of pathogenic strains formed appressorium at 12 hai and reached 100 % at 48 haI, while for endophytic strains 48 % were observed at 12 hai and 70 % at 48 hai (Figure 1B).

Figure 1 A) pathogenic and endophytic conidia germination of C. guaranicola, B) appressoria formation by endophytic and pathogenic C. guaranicola strains.
No difference was observed in the morphology of appressorium produced by endophytic or pathogenic strains. Appressoria presented globose to subglobose form with the presence of melanin. According to Ryder and Talbot (2015), the presence of melanin in the appressorium keeps the pressure of turgor due to a lowering in the porosity of the appressorium cell wall. Fungal melanin does not only serve as protection against environmental stresses but also determine virulence in some pathogenic fungi (Ludwig et al., 2014). Melanin impregnation in the appressoria wall enables Colletotrichum species to build up high osmotic pressure and finally penetrate itself into the cell wall (Reboledo et al., 2015).
In the genus Colletotrichum, conidia germination and appressoria formation occur between 3 and 48 h after conidia deposition (Araujo and Stadnik, 2013). Its speed is strongly influenced by environmental factors and chemical or physical signs present on the surface of the host. Bentes and Matsuoka (2002) observed that the beginning of conidia germination for pathogenic C. guaranicola occurred between 6 and 12 hai, reaching the maximum of germination in the period of 24 hai (94.7 %). The same authors also observed that appressoria formation reached its maximum within 24 hai (94 %).
Colonization of plant tissues by the pathogenic strain I 06 was evidenced by the appearance of symptoms at 48 hai. No symptoms were observed seven days after inoculation in the plants inoculated with the endophytic strain P10. Evaluation of sections of leaf tissue showed colonization by the pathogenic strain I 06 in the cells of the epidermis and palisade parenchyma. Intracellular hyphae presence and parenchyma cells degradation were observed (Figure 2).
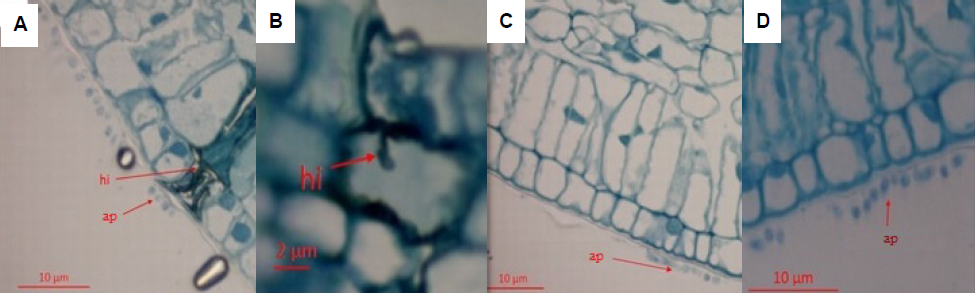
Figure 2 Process of C. guaranicola pathogenic and endophytic colonization in leaves of susceptible guarana (clone 300) within 48 hai. A) hyphae (hi) of the C. guaranicola pathogenic strain colonizing the tissues of the host plant, B) infection process of pathogenic strain with presence of appressoria (ap) on the surface and penetration hypha, C) and D) endophytic appressoria on the leaf surface of the host plant.
Pre-penetration and colonization processes by pathogenic C. guaranicola strains in guarana resembled those observed for other Colletotrichum species (Barimani et al., 2013; Bentes and Matsuoka, 2002; Jayawardena et al., 2016). Appressoria presence was observed on the surface of leaf epidermis in the samples inoculated with the endophytic strain P10 but no cell colonization was observed in the sections analyzed at 48 hai (Figure 2). Delay in tissue colonization by the endophytic strain (P10), compared to the pathogenic one (I 06), can be related to pathogenicity mechanisms, which make the pathogenic strain more efficient in degrading the host natural barriers and colonizing cells.
Despite that results show no in vitro variation in enzymatic activity among strains, the pathogenic strains were more efficient in infecting and colonizing guarana tissues, resulting in symptoms at 48 hai. The pre-invasion perception of plant-derived signals reprograms the fungal gene expression, a genome-wide expression, and secondary metabolism enzymes are induced before penetration and tissue colonization (O’Connell et al., 2012). In presence of host tissue, some enzyme can be produced in different amount and it might be related to the variable ability of different Colletotrichum strains to effectively penetrate and spread into host plant tissues performing a higher level of virulence.
The lifestyle patterns of Colletotrichum species are highly regulated by both specific gene families and biochemical interactions that occur through specific enzymes and secondary metabolites produced at the host-pathogen interface (De Silva et al., 2017). Results observed in this study were similar to those reported by Horowitz et al. (2002), who studied colonization of pathogenic and endophytic strains of C. acutatum on strawberry; they observed that pathogens quickly developed into the host tissues, causing tissue necrosis four days after inoculation.
Endophytes conidia presented germination by forming a germinative tube and giving rise to the appressoria, that failed to penetrate the leaf tissue, resulting in an epiphytic growth without invasion into the plant. Penetration of endophytic was observed approximately seven days after inoculation and was restricted to the intercellular spaces of subcuticular layer of the hosts, without causing any visible damage. According to Freeman and Rodriguez (1993), a single gene mutation transformed a Colletotrichum magna pathogenic strain into an endophytic one, leading to slight differences between both strains. Overall, the effect of pre-penetration and penetration of Colletotrichum species appear to be similar; however, there are differences between species in the mechanisms of adhesion, melanization and cutinase production for penetration into the cuticle of the plant (Perfect et al., 1999).
Conclusions
There was no enzymatic difference between the pathogenic and endophytic strains evaluated in the present study. Pre-germination data showed differences between endophytic and pathogenic strains. Tissue colonization by pathogenic strain was evidenced by the appearance of symptoms 48 hai while for the samples inoculated with endophytic strains, the presence of appressoria was observed on the surface of the epidermis 48 hai, and no cell colonization was observed. The effect of pre-penetration and penetration of Colletotrichum species appears to be similar; even though, there are differences between species in the mechanisms of adhesion, melanization and cutinase production for penetration into the plant cuticle.