Services on Demand
Journal
Article
Indicators
-
Cited by SciELO
-
Access statistics
Related links
-
Similars in SciELO
Share
Revista mexicana de fitopatología
On-line version ISSN 2007-8080Print version ISSN 0185-3309
Rev. mex. fitopatol vol.36 n.1 Texcoco Jan./Apr. 2018
https://doi.org/10.18781/r.mex.fit.1710-7
Review articles
Phosphites as alternative for the management of phytopathological problems
1 Facultad de Agronomía, Universidad Autónoma de Sinaloa. Carretera Culiacán-Eldorado Km 17.5, Aparatado Postal 25, CP. 80000, Culiacán, Sinaloa, México.
Phosphites are compounds derived from phosphorous acid used as an alternative for the control of phytoparasitic organisms and their effectiveness has been tested against protozoa, oomycetes, fungi, bacteria and nematodes; however, compared to conventional synthesized fungicides, phosphites are generally less effective at reducing damage by phytopathogens. The phosphite ion is easily transported in the plants via xylem and phloem, so it has been used in foliar application, drench of plant root and neck, injection trunk, through drip irrigation mixed in the nutrient solution in hydroponics, seed treatment, aerial application in low volume, or as treatment in immersion of seeds and fruits. The mechanisms of action involved in the prophylactic effects of phosphites are diverse and include the stimulation of biochemical and structural defense mechanisms in plants and direct action that restricts the growth, development and reproduction of phytopathogenic organisms.
Key words: potassium phosphite; biostimulant; control of phytopathogens
Los fosfitos son compuestos derivados del ácido fosforoso empleados como alternativa para el control de organismos fitoparásitos y su eficacia se ha probado contra protozoarios, oomycetes, hongos, bacterias y nematodos; sin embargo, en comparación con los fungicidas convencionales sintetizados, generalmente son menos eficaces para disminuir el daño por fitopatógenos. El ion fosfito es fácilmente transportado en las plantas vía xilema y floema, por lo que se ha utilizado en aplicación foliar, baño a la raíz y cuello de la planta, inyección al tronco, a través de riego por goteo mezclado en la solución nutritiva en hidroponía, tratamiento a semilla, aplicación aérea en bajo volumen o como tratamiento en inmersión de semillas y frutos. Los mecanismos de acción involucrados en los efectos profilácticos de los fosfitos son diversos e incluyen la estimulación de los mecanismos de defensa bioquímica y estructural en las plantas, además de la acción directa que restringe el crecimiento, desarrollo y reproducción de los organismos fitopatógenos.
Palabras clave: fosfito de potasio; bioestimulante; control de fitopatógenos
The irrational use of synthetic pesticides in agriculture increases problems of environmental pollution and public health, and decreases biodiversity in the agroecosystems as well as the development of resistant-phytopathogenic organisms. Furthermore, the current agricultural marketing requires products that are safe for consumers and created using low environmental impact processes. Thus, disease control based on the use of inorganic salts, which aside from being effective in managing crop diseases have minimum adverse consequences, becomes relevant. To this regard, Deliopoulos et al. (2010) reported that 34 different salts have been used for such purpose, and suggested that phosphite salts stand out because of their use frequency and control effectiveness.
Phosphites are oxyanions derived from phosphorous acid (H3PO3 -) regularly combined with non-metal cations, such as potassium, sodium, calcium or ammonia. The terms “phosphite” and “phosphonate” are commonly used in the literature to refer to salts derived from phosphorous acid, the same as “hydrogen phosphates”, “orthophosphites”, “phosphonic acid compounds” and “phosphorous acid compounds” (Deliopoulos et al., 2010).
The chemical difference between phosphate (H2PO4 -) and phosphite (H2PO3 -) is an oxygen atom that is replaced by another of hydrogen (Figure 1). When oxygen is replaced, there is a profound difference in the way phosphates and phosphites behave in living organisms (McDonald et al., 2001; Achary et al., 2017). Due to their structural similarity, phosphites are thought to be akin to phosphates. However, the use of phosphites as fungicides and biostimulants to control plant pathogens is widely accepted, but their use as a phosphorus source in plant nutrition is currently debated (Borza et al., 2014; Gómez-Merino and Trejo-Téllez, 2015; Alexandersson et al., 2016; Manna et al., 2016). Such difference gives phosphites a greater mobility in soil and plant tissues, as well as a greater capacity to penetrate through leaves, stems and roots (Tkaczyk et al., 2016).
The use of phosphites in agriculture has been studied mainly because of their action to control phytoparasite organisms or as a nutrition source for cultivated plants (Gómez-Merino and Trejo-Téllez, 2015; Alexandersson et al., 2016). The latter may occur only if phosphites are applied to soil and come into contact with bacteria that have the capacity to oxidize them to phosphates (McDonald et al., 2001; Manna et al., 2016). However, since this is a very slow process that can take up to four months, it becomes impractical in agriculture (McDonald et al., 2001; Lovatt and Mikkelsen, 2006).
In early 1930, researchers concluded that phosphites were not an efficient nutrition source for plants. However, 40 years later, phosphites returned to the agrochemicals market as an alternative for controlling plant diseases, when the French company Rhône-Poulenc offered the active ingredient fosetyl-aluminum to control mildews and diseases caused the Phytophthora genus (Tkaczyk et al., 2016; Achary et al., 2017). Phosphites, as an alternative for controlling phytoparasite organisms, have been extensively studied and proven to be effective against protozoa, oomycetes, fungi, bacteria and phytoparasite nematodes (Table 1), as well as biostimulators (Gómez-Merino and Trejo-Téllez, 2015) that reduce damages caused by weeds (Manna et al., 2016; Achary et al., 2017) and UV-B radiation (Oyarburo et al., 2015).
Table 1 Control of phytoparasite organisms using phosphites.
Organismo fitoparásito | Planta hospedante | Fosfito | Referencia |
Protozoarios | |||
Plasmodiophora brassicae | Brassica rapa | Potasio | Kammerich et al., 2014 |
Oomycetes | |||
Peronospora destructor | Allium cepa | Potasio | Monsalve et al., 2012 |
P. manshurica | Glicine max | Potasio | Silva et al., 2011 |
P. parasitica | Brassica oleracea | Potasio | Becót et al., 2000 |
Phytophthora cinnamomi | Macadamia spp. | Potasio | Akinsanmi y Dreth, 2013 |
P. cinnamomi | Ananas comosus | Potasio | Anderson et al., 2012 |
Phytophthora infestans | Solanum tuberosum | Aluminio | Kromann et al., 2012 |
P. infestans | S. tuberosum | Calcio | Lobato et al., 2008 |
P. infestans | S. tuberosum | Potasio | Borza et al., 2017 |
P. infestans | S. tuberosum | Potasio | Kromann et al., 2012 |
P. infestans | S. tuberosum | Potasio | Liljeroth et al., 2016 |
P. nicotianae | Nicotiana tabacum | Potasio | Smillie et al., 1989 |
P. palmivora | Carica papaya | Potasio | Smillie et al., 1989 |
Plasmopara viticola | Vitis vinifera | Potasio | Pinto et al., 2012 |
Pseudoperonospora cubensis | Cucumis melo | Potasio | Méndez et al., 2010 |
Pythium aphanidermatum | C. sativus | Cobre | Abbasi y Lazarovits, 2006 |
P. irregulare | C. sativus | Cobre | Abbasi y Lazarovits, 2006 |
P. ultimum | C. sativus | Cobre | Abbasi y Lazarovits, 2006 |
P. ultimum | C. sativus | Potasio | Mofidnakhaei et al., 2016 |
Hongos | |||
Alternaria alternata | Malus domestica | Potasio | Reuveni et al., 2003 |
Cercospora coffeicola | Coffea arabica | Potasio | Costa et al., 2014 |
Cochliobolus miyabeanus | Oryza sativa | Potasio | Nascimento et al., 2016 |
Colletotrichum gloeosporioides | C. arabica | Potasio | Ogoshi et al., 2013 |
C. gloeosporioides | Fragaria vesca | Potasio | MacKenzie et al., 2009 |
C. gloeosporioides | Malus domestica | Potasio | Araujo et al., 2010 |
Fusarium solani | Solanum tuberosum | Calcio | Lobato et al., 2008 |
F. solani | S. tuberosum | Potasio | Lobato et al., 2008 |
Fusicladium effusum | Carya illinoinensis | Potasio | Bock et al., 2012 |
Hemileia vastatrix | Coffea arabica | Potasio | Costa et al., 2014 |
Oidium sp. | Cucumis sativus | Potasio | Yáñez et al., 2012 |
Oidium sp. | C. sativus | Potasio | Yáñez et al., 2014 |
Penicillium digitatum | Citrus limon | Potasio | Cerioni et al., 2013 |
P. italicum | C. limon | Potasio | Cerioni et al., 2013 |
P. expansum | Malus domestica | Potasio | Amiri y Bompeix, 2011 |
P. expansum | Malus domestica | Potasio | Lai et al., 2017 |
Rhizoctonia solani | Solanum tuberosum | Calcio | Lobato et al., 2008 |
R. solani | S. tuberosum | Potasio | Lobato et al., 2008 |
Venturia inaequalis | S. tuberosum | Potasio | Percival et al., 2009 |
V. pirina | Pyrus communis | Potasio | Percival et al., 2009 |
Bacterias | |||
Erwinia carotovora | Solanum tuberosum | Potasio | Lobato et al., 2011 |
E. amylovora | Pyrus malus | Potasio | Aćimović et al., 2015 |
Pseudomonas syringae pv. actinidiae | Actinidia deliciosa | Aluminio | Monchiero et al., 2015 |
Nematodos | |||
Helicotylenchus spp. | Musa paradisiaca | Potasio | Quintero-Vargas y Castaño-Zapata, 2012 |
Heterodera avenae | Avena sativa | Potasio | Oka et al., 2007 |
Meloidogyne marylandi | Triticum aestivum | Potasio | Oka et al., 2007 |
Meloidogyne spp. | M. paradisiaca | Potasio | Quintero-Vargas y Castaño-Zapata, 2012 |
Pratylenchus bracyurus | Glycine max | Potasio | Dias-Areira et al., 2012 |
Pratylenchus bracyurus | Zea mays | Potasio | Dias-Areira et al., 2012 |
P. bracyurus | Zea mays | Manganeso | Puerari et al., 20015 |
Radopholus similis | M. paradisiaca | Potasio | Quintero-Vargas y Castaño-Zapata, 2012 |
Application methods
The phosphite ion is easily transported in plants via xylem and phloem (McDonald et al., 2001; Tkaczyk et al., 2016), so it has been used in foliar applications (Rebollar-Alviter et al., 2010; Silva et al., 2011; Pagani et al., 2014; Yáñez et al., 2014; Liljeroth et al., 2016; Borza et al., 2017), drench of plants root and neck (Oka et al., 2007; Akinsanmi and Dreth, 2013), trunk injection (Bock et al., 2012; Akinsanmi and Drenth, 2013: Aćimović et al., 2015; Aćimović et al., 2016) through drip irrigation mixed in a nutrient solution in hydroponics (Förster et al., 1998), seed treatments (Abbasi and Lazarovits 2006; Lobato et al., 2008), low-volume aerial applications (Hardy et al., 2001) or as a treatment in seed and fruits immersion (Anderson et al., 2012; Cerioni et al., 2013, Borin et al., 2017).
Effectiveness
The levels of effectiveness of phosphites to control phytoparasite organisms vary depending on the ion bonded to phosphite, application method, pathogen organism and host plant (Table 2; Figure 2). For example, mandarin orange fruits immersed in solutions containing calcium and potassium phosphites contributed to reduce 50% of fruits infected with citrus green mold caused by Penicillium digitatum (Cerioni et al., 2013); also, soja plants treated with potassium phosphite showed up to 50% less damage by Peronospora manshurica than non-treated plants (Silva et al., 2011). Abbasi and Lazarovits (2006) reported 80% less cucumber plants infected by Pythium spp. when seeds were treated by immersion in solutions containing copper phosphite. Ogoshi et al. (2013) obtained a 62.5% decrease in the severity of Colletotrichum gloeosporioides in coffee plants treated with potassium phosphite. In another experiment, 93% of papaya plants treated with potassium phosphite survived the attack of Phytophthora palmivora, but only 24% of non-treated plants survived (Vawdrey and Westerhuis, 2007).
Table 2 Pathogen sensitivity to phosphites in potato (Taken from Lobato et al., 2010).
Fosfito de calcio | Fosfito de potasio | Fosfito de cobre | |
Patógeno | mg mL-1 | ||
Phytophthora infestans | 0.09x | 0.15 | <0.04 |
Rhizoctonia solani | 1.87 | >3.56 | 1.04 |
Fusarium solani | 1.29 | >3.56 | 0.68 |
Streptomyces scabies | 0.83 | 1.99 | 0.22 |
xCompound concentration to inhibit 50% growth.
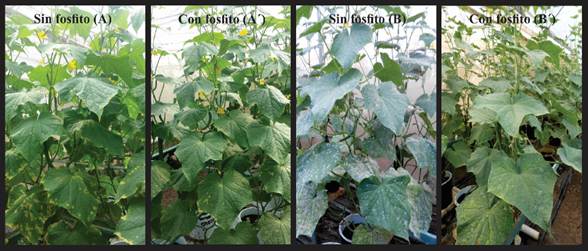
Figure 2 Incidence and severity of Pseudoperonospora cubensis (A and A´) and Sphaerotheca fuliginea (B and B´) in cucumber plants cultivated in the greenhouse.
Compared with conventional synthetic fungicides, phosphites are usually less effective to reduce damages caused by phytopathogens. Méndez et al. (2010) were able to reduce the damage caused by Pseudoperonospora cubensis in melon plants treated with chlorothalonil and mancozeb, but not in those that were treated with potassium phosphite (Figure 3). Aćimović et al. (2016) were also able to significantly reduce the incidence of Erwinia amylovora using streptomycin sulfate, compared with the incidence of the pathogen when they used potassium phosphite (Figure 4); the compounds were injected into apple tree trunks. Meyer and Hausbeck (2013) reported a significant decrease in the incidence of death squash plants infected by Phytophthora capsici when they were treated with fluopicolide, mandipropamid or dimethomorph fungicides, compared with the incidence of plants treated with potassium phosphite. In general, the reports on the effectiveness of phosphites and conventional fungicides in controlling phytoparasite organisms suggest that phosphites are less effective and could not replace fungicides at all, but that if they are included as part of an integrated pest management program they could help reduce the use of fungicides as well as the probability that the organisms develop resistance (Liljeroth et al., 2016). Méndez et al. (2010) reported similar effectiveness against Pseudoperonospora cubensis in squash plants by alternately using chlorothalonil/mancozeb and potassium phosphite compared with the effectiveness when using only fungicides (Figure 3). Liljeroth et al. (2016) also reported that the protection against Phytophthora infestans in potato was similar using flluazinam, cyazofamid, mandipropamid, metalaxyl + flluazinam or flluopicolide + propamocarb at 100% of the recommended dose, or at 50% of the dose mixed with potassium phosphite.

Figure 3 Average area under the progress curve (ABCPE) from a treatment against Pseudoperonospora cubensis in cucumber plants (Developed by the authors with data from Méndez et al., 2010).

Figure 4 Control of Erwinia amylovora after two applications of a resistance inducer or antibiotics to trunks of apple trees (Developed by the authors with data from Aćimović et al., 2016).
Action mode
The action mechanisms involved in the prophylactic effects of phosphites include direct and indirect action. It has been stated that when the phosphite ion come into contact with phytopathogen organisms, it affects their growth and reproduction, because it influences the expression of genes that encode the synthesis of essential compounds in their cell structure and physiology (direct action). When the phosphite ion enters the plant tissue cells (indirect action) activates biochemical (production of: polysaccharides, proteins related to pathogenesis, phytoalexins, etc.) and structural defense (such as callose deposition) mechanisms that restrict pathogens penetration and survival in the plant (Figure 5).

Figure 5 Action mode of phosphites, schematic representation (developed by the authors using data from Daniel and Guest, 2006; Jackson et al., 2000; King et al., 2010; Eshraghi et al., 2011; Olivieri et al., 2012; Cerioni et al., 2013; Dalio et al., 2014).
Direct action
The addition of phosphites to the medium culture reduced mycelium growth, as well as the number of spore-generating structures and of produced and germinated spores (Figure 6). Thus, with this practice, we observed a growth decrease of Alternaria alternata (Reuveni et al., 2003), Colletotrichum gloeosporioides (Araujo et al., 2010), Fusarium culmorum and F. graminearum (Hofgaard et al., 2010), Mycosphaerella fijiensis (Mogollón and Castaño, 2012), Penicillium digitatum (Cerioni et al., 2013), Phytophthora cinnamomi (Wilkinson et al., 2001; Won et al., 2009; King et al., 2010), P. cinnamomi, P. palmivora and P. nicotianae (Smillie et al., 1989), P. infestans (Bórza et al., 2014), P. plurivora (Dalio et al., 2014) and Pythium aphanidermatum, P. ultimum, P. irregulare, P. myriotylum, P. torulosum, P. volutum and P. graminicola (Cook et al., 2009). The decrease level in the microorganisms’ growth as a consequence of the phosphite ion is determined by the organism tested, amount of phosphite added to the culture medium, type of ion bonded to phosphite and pH produced by the culture medium (Lobato et al., 2010).

Figure 6 Pythium aphanidermatum in Potato Dextrose Agar (PDA) culture medium (left) and distilled water (right). A and A´ without potassium phosphite; B and B´ with potassium phosphite.
The susceptibility of the microorganisms to the phosphite ion was demonstrated by Wong et al. (2009), who determined the negative effect of phosphite and the positive effect of phosphate on Phythopthora cinnamomic growth, when grown in a culture medium enriched with salts containing each ion individually.
As for interspecific susceptibility, Hofgaard et al. (2010) were able to reduce Fusarium culmorum, F. graminearum and Microdochium majus mycelium growth by 60, 80 and 90%, respectively, using 10 μl ml-1 of potassium phosphite. To inhibit Phytophthora infestans in vitro growth, Lobato et al. (2010) used a lower dose, followed by the dose used to inhibit Streptomyces scabies, Rhizoctonia solani and Fusarium solani growth.
The intraspecific susceptibility was studied by Wilkinson et al. (2001), who determined that from 21 Phytophthora cinnamomi isolates collected in western Australia, 9% were susceptible, 82% intermediate susceptible and 9% tolerant. Smillie et al. (1989) showed Phytophthora cinnamomi, P. palmivora and P. nicotiana susceptibility to potassium phosphite, and explained that as the concentration of phosphite increased in the culture medium, the weight of the biomass produced by Phytophthora species decreased. Cook et al. (2009) also evaluated the concentration of phosphite and mefenoxan fungicide needed to inhibit 50% mycelium growth of eight Pythium aphanidermatum isolates, and reported that all the isolates were susceptible to both compounds, but that the concentrations of phosphite were higher than those of the fungicide.
On the other hand, the phosphate ion reduced pH in the culture medium at acidity levels that were associated with Phytophthora infestans, Rhizoctonia solani, Fusarium solani and Streptomyces scabies low growth (Lobato et al., 2010). Araujo et al. (2010) reported a larger decrease in the diameter of Colletotrichum gloeosporioides colonies as well as in the growth speed index using formulations containing potassium phosphite, which reduced pH in the medium culture. Also, potassium phosphite affected Alternaria alternata (Reuveni et al., 2003), Colletotrichum gloeosporioides (Ogoshi et al., 2013), Penicillium digitatum (Cerioni et al., 2013), P. expansum (Amiri and Bompeix, 2011) and Peronospora parasitica (Becót et al., 2000) spore germination, as well as Fusarium oxysporum f sp. cubense (Davis and Grant, 1996), Mycosphaerella fijiensis (Mogollón y Castaño, 2012), Peronospora sparsa (Hukkanen et al., 2008), Phytophthora plurivora (Dalio et al., 2014) and P. cinnamomi (Wong et al., 2009) spore production.
The effect of phosphites added to a medium culture was explained by King et al. (2010), who reported changes induced by potassium phosphite in the expression of the genes that encode protein synthesis and builds Phytophthora cinnamomi cells and cytoskeleton, which caused hyphae distortion and cell wall lysis.
Indirect action
The indirect action of the phosphite ion includes stimulation of structural and biochemical defense mechanisms in plants. Pilbeam et al. (2011) described lignin and suberine deposition around tissue damaged by Phytophthora cinnamomi in eucalyptus plants treated with potassium phosphite, which limited the pathogen’s development. Olivieri et al. (2012) reported an increased pectin content in periderm and cortex tissue and tubers from potato plants treated with potassium phosphite, a feature that improves resistance to different pathogens. Jackson et al. (2000) reported that the development of lesions caused by P. cinnamomi was highly restricted when they applied a high concentration of phosphite in Eucalyptus marginata tissue, and that the reduced number of lesions was associated with a significant increase of defense enzymes (4-coumarate coenzyme A ligase (4CL) and cinnamyl-alcohol dehydrogenase) and soluble phenols. Daniel and Guest (2006) demonstrated that when Arabidopsis thaliana plantlets were treated with potassium phosphite and inoculated with Phytophthora palmivora zoospores, the infected cells showed increased cytoplasmic activity, development of cytoplasmic aggregates, release of hydrogen peroxide, located cell death, and enhanced phenolic compounds accumulation. Eshraghi et al. (2011) also reported that when Arabidopsis thaliana plantlets were treated with potassium phosphite and inoculated with Phytophthora cinnamomi, the infected cells increased their production of calose and hydrogen peroxide.
Conclusions
Phosphites are effective compounds to control protozoa, oomycetes, fungi, bacteria and phytoparasite nematodes, but compared with synthetic conventional fungicides, they tend to be less effective. The effectiveness level of phosphites to solve phytopathological problems is associated with the problem organism, the host plant and the ion bonded to phosphite. Because of its efficient translocation in plant tissue, they can be applied to canopy, stems, roots or fruits. The action mechanisms involved in the prophylactic effects of phosphites are diverse and include the stimulation of biochemical and structural defense mechanisms in plants, besides the direct action that limits phytoparasite organisms’ growth, development and reproduction. Integrating phosphites as part of a phytopathological problems management program allows to reduce the number of fungicide applications as well as the probability that the organisms develop resistance.
Literatura citada
Abbasi PA and Lazarovits G. 2006. Seed treatment with phosphonate (AG3) suppresses Pythium damping-off of cucumber seedlings. Plant Disease 90:459-464. https://doi.org/10.1094/PD-90-0459 [ Links ]
Achary VMM, Ram B, Manna M, Datta D, Bhatt A, Reddy M and Agrawal PR. 2017. Phosphite: a novel P fertilizer for weed management and pathogen control. Plant Biotechnology Journal 1-16. https://doi.org/10.1111/pbi.12803 [ Links ]
Aćimović SG, Zeng Q, McGhee GC, Sundin GW and Wise JC. 2015. Control of fire blight (Erwinia amylovora) on apple trees with trunk-injected plant resistance inducers and antibiotics and assessment of induction of pathogenesis-related protein genes. Front. Plant Science 6:1-6. https://doi.org/10.3389/fpls.2015.00016 [ Links ]
Aćimović SG, VanWoerkom AH, Garavaglia T, Vandervoort C, Sundin GW and Wise JC. 2016. Seasonal and cross-seasonal timing of fungicide trunk injections in apple trees to optimize management of apple scab. Plant Disease 100:1606-1616. https://doi.org/10.1094/PDIS-09-15-1061-RE [ Links ]
Akinsanmi OA and Drenth A. 2013. Phosphite and metalaxyl rejuvenate macadamia trees in decline caused by Phytophthora cinnamomi. Crop Protection 53:29-36. https://doi.org/10.1016/j.cropro.2013.06.007 [ Links ]
Alexandersson E, Mulugeta T, Lankinen Å, Liljeroth E and Andreasson E. 2016. Plant resistance inducers against pathogens in Solanaceae species-from molecular mechanisms to field application. International Journal Molecular Sciences 17:1-25. https://doi.org/10.3390/ijms17101673 [ Links ]
Amiri A and Bompeix G. 2011. Control of Penicillium expansum with potassium phosphite and heat treatment. Crop Protection 30:222-227. https://doi.org/10.1016/j.cro-pro.2010.10.010 [ Links ]
Anderson JM, Pegg KG, Scott C and Drenth A. 2012. Phosphonate applied as a pre-plant dip controls Phytophthora cinnamomi root and heart rot in susceptible pineapple hybrids. Australasian Plant Pathology 41:59-68. https://doi.org/10.1007/s13313-011-0090-6 [ Links ]
Araujo L, Valdebenito-Sanhueza RM and Stadnik MJ. 2010. Avaliação de formulações de fosfito de potássio sobre Colletotrichum gloeosporioides in vitro e no controle pósinfeccional da mancha foliar de Glomerella em macieira. Tropical Plant Pathology 35:54-59. http://www.scielo.br/pdf/tpp/v35n1/a10v35n1 [ Links ]
Becót S, Pajot E, Le Corred D, Monot C and Silué D. 2000. Phytogard (K2HPO3) induces localized resistance in cauliflower to downy mildew of crucifers. Crop Protection 19:417-425. https://doi.org/10.1016/S0261-2194(00)00034-X [ Links ]
Bock CH, Brenneman TB, Hotchkiss MW and Wood BW. 2012. Evaluation of a phosphite fungicide to control pecan scab in the southeastern USA. Crop Protection 36:58-64. https://doi.org/10.1016/j.cropro.2012.01.009 [ Links ]
Borin RC, Possenti JC, Rey MS, Bernardi C and Mazaro SM. 2017. Phosphites associated to fungicides for diseases control and sanity in corn seeds. Applied Research and Agrotechnology 10:83-92. https://doi.org/10.5935/PAeT.V10.N1.09 [ Links ]
Borza T, Peters RD, Wu Y, Schofield A, Rand J, Ganga Z, Al-Mughrabi KI, Coffin RH and Wang-Pruski G. 2017. Phosphite uptake and distribution in potato tubers following foliar and postharvest applications of phosphite-based fungicides for late blight control. Annals of Applied Biology 1:127-139. https://doi.org/10.1111/aab.12322 [ Links ]
Borza T, Schofield A, Sakthivel G, Bergese J, Gao X, Rand J and Wang-Pruski G. 2014. Ion chromatography analysis of phosphite uptake and translocation by potato plants: dose-dependent uptake and inhibition of Phytophthora infestans development. Crop Protection 56:74-81. https://doi.org/10.1016/j.cropro.2013.10.024 [ Links ]
Cerioni L, Rapisarda VA, Doctor J, Fikkert S, Ruiz T, Fassel R and Smilanick JL. 2013. Use of phosphite salts in laboratory and semicommercial tests to control citrus postharvest decay. Plant Disease 97:201-212. http://dx.doi.org/10.1094/PDIS-03-12-0299-RE [ Links ]
Cook PJ, Landschoot PJ and Schlossberg MJ. 2009. Inhibition of Pythium spp. and suppression of Pythium blight of turfgrasses with phosphonate fungicides. Plant Disease 93:809-814. https://doi.org/10.1094/PDIS-93-8-0809 [ Links ]
Costa BHG, De Resende MLV, Ribeiro PM, Mathioni SM, Padua MS and Da Silva MBJ. 2014. Suppression of rust and brown eye spot diseases on coffee by phosphites and by-products of coffee and citrus industries. Journal of Phytopathology 162:635-642. https://doi.org/10.1111/jph.12237 [ Links ]
Dalio RJD, Fleischmann F, Humez M and Osswald W. 2014. Phosphite protects Fagus sylvatica seedlings towards Phytophthora plurivora via local toxicity, priming and facilitation of pathogen recognition. PLoS One 9, e87860. https://doi.org/10.1371/journal.pone.0087860 [ Links ]
Daniel R and Guest D. 2006. Defence responses induced by potassium phosphonate in Phytophthora palmivora-challenged Arabidopsis thaliana. Physiologycal and Molecular Plant Pathology 67:194-201. https://doi.org/10.1016/j.pmpp.2006.01.003 [ Links ]
Davis AJ and Grant GR. 1996. The effect of phosphonate on the sporulation of Fusarium oxysporum f. sp. cubense. Australasian Plant Pathology 25:31-35. https://doi.org/10.1071/AP96007 [ Links ]
Dias-Arieira CRP, Marini PM, Fontana LF, Roldi M and Silva TRB. 2012. Effect of Azospirillum brasilense, Stimulate® and potassium phosphite to control Pratylenchus brachyurus in soybean and maize. Nematropica 42:170-175. http://journals.fcla.edu/nematropica/article/view/79597/76915 [ Links ]
Deliopoulos T, Kettlewell PS and Hare MC. 2010. Fungal disease suppression by inorganic salts: a review. Crop Protection 29:1059-1075. https://doi.org/10.1016/j.cro-pro.2010.05.011 [ Links ]
Eshraghi L, Anderson J, Aryamanesh N, Shearer B, McComb J, Hardy GES and O’Brien PA. 2011. Phosphite primed defence responses and enhanced expression of defence genes in Arabidopsis thaliana infected with Phytophthora cinnamomi. Plant Pathology 60:1086-1095. https://doi.org/10.1111/j.1365-3059.2011.02471.x [ Links ]
Förster H, Adaskaveg JE, Kim DH and Stanghellini ME. 1998. Effect of phosphite on tomato and pepper plants and on susceptibility of pepper to Phytophthora root and crown rot in hydroponic culture. Plant Disease 82:1165-1170. https://doi.org/10.1094/PDIS.1998.82.10.1165 [ Links ]
Gómez-Merino FC and Trejo-Téllez LI. 2015. Biostimulant activity of phosphite in horticulture. Scientia Horticulturae 196:82-90. https://doi.org/10.1016/j.scienta.2015.09.035 [ Links ]
Hardy GES, Barrett S and Shearer BL. 2001. The future of phosphite as a fungicide to control the soilborne plant pathogen Phytophthora cinnamomi in natural ecosystems. Australians Plant Pathology 30:133-139. https://doi.org/10.1071/AP01012 [ Links ]
Hofgaard IS, Ergon A, Henriksen B and Tronsmo AM. 2010. The effect of potential resistance inducers on development of Microdochium majus and Fusarium culmorum in winter wheat. European Journal of Plant Pathology 128:269-281. https://doi.org/10.1007/s10658-010-9662-5 [ Links ]
Hukkanen A, Kostamo K, Kärenlampi S and Kokko H. 2008. Impact of agrochemicals on Peronospora sparsa and phenolic profiles in three Rubus arcticus cultivars. Journal of Agricultural and Food Chemistry 56:1008-1016. https://doi.org/10.1021/jf072973p [ Links ]
Jackson TJ, Burgess T, Colquhoun I and Hardy GESTJ. 2000. Action of the fungicide phosphite on Eucalyptus marginata inoculated with Phytophthora cinnamomi. Plant Pathology 49:147-154. https://doi.org/10.1046/j.1365-3059.2000.00422.x [ Links ]
Kammerich J, Beckmann S, Scharafat I and Ludwig-Müller J. 2014. Suppression of the clubroot pathogen Plasmodiophora brassicae by plant growth promoting formulations in roots of two Brassica species. Plant Pathology 63:846-857. https://doi.org/10.1111/ppa.12148 [ Links ]
King M, Reeve W, Van der Hoek M.B, Williams N, McComb J, O’Brien PA and Hardy GE. 2010. Defining the phosphite-regulated transcriptome of the plant pathogen Phytophthora cinnamomi. Mol Genet Genomics 284:425-35. https://doi.org/10.1007/s00438-010-0579-7 [ Links ]
Kromann P, Pérez WG, Taipe A, Schulte-Geldermann E, Sharma BP, Andrade-Piedra JL and Forbes GA. 2012. Use of phosphonate to manage foliar potato late blight in developing countries. Plant Disease. 96:1008-1015. https://apsjournals.apsnet.org/doi/pdf/10.1094/PDIS-12-11-1029-RE [ Links ]
Lai T, Wang Y, Fan Y, Zhou Y, Bao Y and Zhou T. 2017. The response of growth and patulin production of postharvest pathogen Penicillium expansum to exogenous potassium phosphite treatment. International Journal of Food Microbiology 244:1-10. https://doi.org/10.1016/j.ijfoodmicro.2016.12.017 [ Links ]
Liljeroth E, Lankinen Å, Wiik L, Burra DD, Alexandersson E and Andreasson E. 2016. Potassium phosphite combined with reduced doses of fungicides provides efficient protection against potato late blight in large-scale field trials. Crop Protection 86:42-55. https://doi.org/10.1016/j.cro-pro.2016.04.003 [ Links ]
Lobato MC, Machinandierena MF, Tambascio C, Dosio GAA, Caldiz DO, Dalio GR, Andreu AB and Olivieri FP. 2011. Effect of foliar applications of phosphite on post-harvest potato tubers. European Journal Plant Pathology 130:155-163. https://doi.org/10.1007/s10658-011-9741-2 [ Links ]
Lobato MC, Olivieri FP, González AEA, Wolski EA, Daleo GR, Caldiz DO and Andreu AB. 2008. Phosphite compounds reduce disease severity in potato seed tubers and foliage. European Journal Plant Pathology 122:349-358. https://doi.org/10.1007/s10658-008-9299-9 [ Links ]
Lobato MC, Olivieri FP, Daleo GR and Andreu AB. 2010. Antimicrobial activity of phosphites against different potato pathogens. Journal Plant Disease Protection 3:102-109. https://doi.org/10.1007/BF03356343 [ Links ]
Lovatt CJ and Mikkelsen RL. 2006. Phosphite fertilizers: what are they? Can you use them? What can they do? Better Crops 90:11-13. https://www.ipni.net/ppiweb/bcrops.nsf/$webindex/3EF696A6E5851563852572140026EACD/$file/06-4p11.pdf [ Links ]
MacKenzie SJ, Mertely JC and Peres NA. 2009. Curative and protectant activity of fungicides for control of crown rot of strawberry caused by Colletotrichum gloeosporioides. Plant Disease 93:815-820. https://doi.org/10.1094/PDIS-93-8-0815 [ Links ]
Manna M, Achary VMM, Islam T, Agrawal PK and Reddy MK. 2016. The development of a phosphite-mediated fertilization and weed control system for rice. Scientific Reports 6:1-13. https://doi.org/10.1038/srep24941 [ Links ]
McDonald AE, Grant BR and Plaxton WC. 2001. Phosphite (phosphorous acid): its relevance in the environment and agriculture and influence on plant phosphate starvation response. Journal Plant Nutrition 24:1505-1519. http://dx.doi.org/10.1081/PLN-100106017 [ Links ]
Méndez LW, Arauz LF y Ríos R. 2010. Evaluación de fungicidas convencionales e inductores de resistencia para el combate de mildiú velloso (Pseudoperonospora cubensis) en melón (Cucumis melo). Agronomía Costarricense 34:153-164. https://revistas.ucr.ac.cr/index.php/agrocost/article/view/3629/3534 [ Links ]
Meyer MD and Hausbeck MK. 2013. Using soil-applied fungicides to manage Phytophthora crown and root rot on summer squash. Plant Disease 97:107-112. https://doi.org/10.1094/PDIS-12-11-1071-RE [ Links ]
Mofidnakhaei M, Abdossi V, Dehestani A, Pirdashti H and Babaeizad V. 2016. Potassium phosphite affects growth, antioxidant enzymes activity and alleviates disease damage in cucumber plants inoculated with Pythium ultimum. Archives of Phytopathology and Plant Protection 49:207-221. http://dx.doi.org/10.1080/03235408.2016.1180924 [ Links ]
Mogollón AM y Castaño J. 2012. Evaluación in vitro de inductores de resistencia sobre Mycosphaerella fijiensis Morelet. Revista Facultad Nacional de Agronomía 65:6327-6336. http://www.redalyc.org/articulo.oa?id=179924340004 [ Links ]
Monchiero M, Lodovica MG, Pugliese M, Spadaro D and Garibaldi A. 2015. Efficacy of different chemical and biological products in the control of Pseudomonas syringae pv. actinidiae on kiwifruit. Australasian Plant Pathology 44:13-23. https://doi.org/10.1007/s13313-014-0328-1 [ Links ]
Monsalve V, Viteri RSE, Rubio CNJ and Tovar DF. 2012. Efectos del fosfito de potasio en combinación con el fungicida metalaxyl + mancozeb en el control de mildeo velloso (Peronospora destructor Berk) en cebolla de bulbo (Allium cepa L.). Revista Facultad Nacional de Agronomía-Medellin 65:6317-6325. http://www.redalyc.org/articulo.oa?id=179924340003 [ Links ]
Nascimento KJT, Araujo L, Resende RS, Schurt DA, Silva WL and Rodrigues FA. 2016. Silicon, acibenzolar-S-methyl and potassium phosphite in the control of brown spot in rice. Bragantia 75:212-221. http://dx.doi.org/10.1590/1678-4499.281 [ Links ]
Ogoshi C, de Abreu MS, da Silva BM, Neto HS, Júnior PMR and de Resende MLV. 2013. Potassium phosphite: a promising product in the management of diseases caused by Colletotrichum gloeosporioides in coffee plants. Bioscience Journal 29:1558-1565. http://www.seer.ufu.br/index.php/biosciencejournal/article/view/17148/13302 [ Links ]
Oka Y, Tkachi N and Mor M. 2007. Phosphite inhibits development of the nematodes Heterodera avenae and Meloidogyne marylandi in cereals. Phytopathology 97:396-404. https://doi.org/10.1094/PHYTO-97-4-0396 [ Links ]
Olivieri FP, Feldman ML, Machinandiarena MF, Lobato MC, Caldiz DO, Dalo GR and Andreu AB. 2012. Phosphite applications induce molecular modifications in potato tuber periderm and cortex that enhance resistance to pathogens. Crop Protection 32:1-6. https://doi.org/10.1016/j.cropro.2011.08.025 [ Links ]
Oyarburo NS, Machinandiarena MF, Feldman ML, Daleo GR, Andreu AB and Olivieri FP. 2015. Potassium phosphite increases tolerance to UV-B in potato. Plant Physiology and Biochemistry 88:1-8. https://doi.org/10.1016/j.plaphy.2015.01.003 [ Links ]
Pagani APS, Dianese AC and Café-Filho AC. 2014. Management of wheat blast with synthetic fungicides, partial resistance and silicate and phosphite minerals. Phytoparasitica 42:609-617. https://doi.org/10.1007/s12600-014-0401-x [ Links ]
Percival GC, Noviss K and Haynes I. 2009. Field evaluation of systemic inducing resistance chemicals at different growth stages for the control of apple (Venturia inaequalis) and pear (Venturia pirina) scab. Crop Protection 28:629-633. https://doi.org/10.1016/j.cropro.2009.03.010 [ Links ]
Pilbeam RA, Howard K, Shearer BL and Hardy GEJ. 2011. Phosphite stimulated histological responses of Eucalyptus marginata to infection by Phytophthora cinnamomi. Tree 25:1121-1131. https://doi.org/10.1007/s00468-011-0587-1 [ Links ]
Pinto KM, Do Nascimento C, Gomes EC, Da Silva HF and Miranda J. 2012. Efficiency of resistance elicitors in the management of grapevine downy mildew Plasmopara viticola: epidemiological, biochemical and economic aspects. European Journal Plant Pathology 134:745-754. https://doi.org/10.1007/s10658-012-0050-1 [ Links ]
Puerari HH, Dias-Arieira CR, Cardoso MR, Hernandes I and Brito ODC. 2015. Resistance inducers in the control of root lesion nematodes in resistant and susceptible cultivars of maize. Phytoparasitica 43:383-389. https://doi.org/10.1007/s12600-014-0447-9 [ Links ]
Quintero-Vargas C y Castaño-Zapata J. 2012. Evaluación de inductores de resistencia para el manejo de nematodos fitoparásitos en plántulas de plátano. Revista de la Academia Colombiana de Ciencias Exactas, Físicas y Naturales 36:575-586. http://www.scielo.org.co/pdf/racefn/v36n141/v36n141a08.pdf [ Links ]
Rebollar-Alviter A, Wilson LL, Madden LV and Ellis MA. 2010. A comparative evaluation of post-infection efficacy of mefenoxam and potassium phosphite with protectant efficacy of azoxystrobin and potassium phosphite for controlling leather rot of strawberry caused by Phytophthora cactorum. Crop Protection 29:349-353. https://doi.org/10.1016/j.cropro.2009.12.009 [ Links ]
Reuveni M, Sheglov D and Cohen Y. 2003. Control of moldy-core decay in apple fruits by β-aminobutyric acids and potassium phosphites. Plant Disease 87:933-936. https://doi.org/10.1094/PDIS.2003.87.8.933 [ Links ]
Silva OC, Santos HAA, Dalla-Pria M and May-De Mio LL. 2011. Potassium phosphite for control of downy mildew of soybean. Crop Protection 30:598-604. https://doi.org/10.1016/j.cropro.2011.02.015 [ Links ]
Smillie R, Grant BR and Guest D. 1989. The mode of action of phosphite: evidence for both direct and indirect modes of action on three Phytophthora spp. in plants. Phytopathology 79:921-926. https://doi.org/10.1094/Phyto-79-921 [ Links ]
Tkaczyk M, Kubiak KA, Sawicki J, Nowakowska JA and Oszako T. 2016. The use of phosphates in forestry. Forest Research Papers 77:76-81. https://doi.org/10.1515/frp-2016-0009 [ Links ]
Vawdrey LL and Westerhuis D. 2007. Field and glasshouse evaluations of metalaxyl, potassium phosphonate, acibenzolar and tea tree oil in managing Phytophthora root rot of papaya in far northern Queensland, Australia. Australasian Plant Pathology 36:270-276. https://doi.org/10.1071/AP07016 [ Links ]
Wilkinson CJ, Holmes JM, Tynan KM, Colquhoun IJ, Mc-comb JA, Hardy GESTJ and Dell B. 2001. Ability of phosphite applied in a glasshouse trial to control Phytophthora cinnamomi in five plant species native to Western Australia. Australasian Plant Pathology 30:343-351. https://doi.org/10.1071/AP01055 [ Links ]
Wong MA, McComb BJ, Hardy BGEJ and O’Brien PA. 2009. Phosphite induces expression of a putative proteophos-phoglycan gene in Phytophthora cinnamomi. Australasian Plant Pathology 38:235-241. https://doi.org/10.1071/AP08101 [ Links ]
Yáñez JMG, Ayala TF, Partida RL, Velázquez AT, Godoy ATP y Díaz VT. 2014. Efecto de bicarbonatos en el control de cenicilla (Oidium sp.) en pepino (Cucumis sativus L.). Revista Mexicana de Ciencias Agrícolas 5:991-1000. http://www.scielo.org.mx/scielo.php?script=sci_arttext&pid=S2007-09342014000600007 [ Links ]
Yáñez JMG, León DJF, Godoy ATP, Gastélum LR, López MM, Cruz OJE y Cervantes DL. 2012. Alternativas para el control de la cenicilla (Oidium sp.) en pepino (Cucumis sativus L.). Revista Mexicana de Ciencias Agrícolas 3:259-270. http://www.redalyc.org/articulo.oa?id=263123201004 [ Links ]
Received: October 31, 2017; Accepted: December 22, 2017