1. Introduction
COATLI will produce images with FWHM of approximately 0.3 arcsec in a field of view (FoV) larger than 4 arcmin over a large fraction of the sky from 550 nm to 920 nm, from the Observatorio Astronómico Nacional (OAN) in Sierra San Pedro Mártir (SPM). The site has seeing values similar to the sites in Hawaii, Chile and the Canary Islands, with an average value of 0.79 arcsec FWHM at 500 nm and a corresponding value of the Fried parameter of 13 cm (Skidmore 2009). By using a combination of fast guiding and active optics, COATLI will be able to obtain images better than the excellent seeing, even in relatively poor atmospheric conditions. The one drawback of the instrument is that the telescope will be relatively small (50 cm), which will limit us to science on relatively bright sources. We estimate 10σ limiting magnitudes in dark/bright time of 23.8/23.4 in r, 23.3/22.9 in i, and 22.6/22.3 in z in 1000 seconds.
As have been exposed in Watson et al. (2016), examples of scientific cases for COATLI can be eclipsing binaries in the Trapezium, HII regions in nearby galaxies, galactic star clusters, location of gammaray bursts in their host galaxies, sub-stellar companions in the solar neighborhood, multiplicity in young clusters, and planetary nebulae in the galactic bulge.
The telescope and instrument COATLI were conceived from the fact that if a telescope diameter is less than 3.3 times the Fried parameter, fast guiding or tip-tilt correction can reduce the phase variance to less than 1 rad2 and hence produce diffractionlimited images (Watson et al. 2016). Theoretically, at the OAN/SPM one could then obtain diffractionlimited images at 500 nm with a 50 cm telescope with fast guiding only. However, in order to deliver this image quality, the static image quality of the telescope and instrument must also be diffractionlimited.
The telescope selected for COATLI is a comercial 50 cm f/8 Ritchey-Chrétien from the company ASTELCO on a German mount. By design, a Ritchey-Chrétien is free of spherical aberration and coma. Nevertheless, telescope misalignments can introduce static aberrations of these two types: spherical aberration caused by errors in the distance between the two mirrors and constant coma caused by tilts and misalignments in any of the mirrors (Wilson 2004). Additionally, irregularities in mirror polishing and mechanical support of the mirrors will introduce astigmatism in the beam. In practice, though, spherical aberration is not a problem, provided the instrument focal plane is close to the telescope focal plane. Therefore, the active optics system will have to correct both static astigmatism and constant coma.
COATLI will do fast guiding using a tip-tilt mirror and active optics correction using a deformable mirror. The deformable mirror is an Adaptica Saturn 48-element push-pull electrostatic mirror. The instrument does not have a wavefront sensor; hence, the aberrations will be detected and measured using the donut method (Tokovinin and Heathcote 2006), which consists of fitting a model of a defocused aberrated beam to an out-of-focus image on the detector. The required defocus will be introduced by the deformable mirror.
In this paper, we present the novelty of the optical design of the instrument and the details of it. In § 2 we present and justify the design architecture; in § 3 we put the optical design of COATLI in a wider context and present a comparison to designs of similar astronomical instruments; in § 4 we present the requirements of the instrument with its nominal image quality and efficiency; in § 5 we discuss the telescope’s aberrations and the active optics correction; in § 6 we discuss the tolerancing of the instrument; in § 7 we present a brief discussion on thermal behavior and ghosts; in § 8 we present the possibility of using the B band for additional science; and we finish with some conclusions in § 9.
2. Design architecture
COATLI will have two channels, one with an EMCCD for tip-tilt correction, namely the blue channel, and one with a standard CCD to avoid excess Poisson noise, namely the red channel. The blue channel will observe at the seeing limit in the g band (from 400 to 550 nm) and the red channel will observe at the diffraction limit in the riz bands (from 550 to 920 nm).
There are several considerations which guide the design architecture. Firstly, the deformable mirror shall be located in a pupil plane, and the blue and red channels shall be separated with a dichroic before the deformable mirror, because its reflectivity in the blue part of the spectrum is not good. Secondly, the tip-tilt mirror shall be located before the dichroic in order for the fast guiding system to work in close loop at the blue channel. And thirdly, the tip-tilt mirror shall be located at another pupil plane, since otherwise it would shift the image of the telescope’s secondary mirror over the deformable mirror, potentially laying out of its small active surface (10.8 mm in diameter). In addition, placing the dichroic in a collimated beam avoids the introduction of asymmetric aberrations to the red channel.
Therefore, the system needs a collimator system common to both the blue and red channels, a pupil transfer relay that conjugates the pupil image from the tip-tilt to the deformable mirror, and two independent camera systems for the two channels. The blue detector is an Andor Ixon EMCCD with 1024×1024 13 μm pixels and the red detector is an Andor Ikon CCD with 2048×2048 13.5 μm pixels.
The two detectors are located approximately on the same side of the telescope, opposite the German mount. The optical design is based on several achromatic lens doublets and one lens triplet for the red camera. The use of lenses as opposed to mirrors is convenient because the instrument needs to be very compact and because it is a visible instrument. The optical layout of COATLI is shown in Figures 1 and 2. Light from the telescope enters the instrument through an entrance window (1) towards a fold mirror (2) which bends the beam in a direction perpendicular to the optical axis of the telescope; the collimator (3), common for the two channels, is an achromat doublet that sends the beam towards the tilt mirror (4), located at a pupil plane; the dichroic (5) then splits the beam at 550 nm, transmitting the red channel and reflecting the blue channel downwards. Blue light is then bent with another fold mirror (6) towards the blue camera (7), an achromatic doublet with a small telephoto effect; blue filters (8) are located in one wheel between the camera and the detector. Red light passes through the dichroic towards the pupil transfer relay, which is formed by two non-identical achromatic doublets (9 and 13); the red filters (10 and 11) are located in two separate wheels before and after the focal plane that lies in the relay; the deformable mirror (13) is located at a second pupil plane, and this sends the light towards the red camera (14), a telephoto triplet. The maximum sizes of the two pupil images are 13.0 mm and 10.7 mm for the pupil images on the tip-tilt mirror and on the deformable mirror, respectively.

Fig. 1 3D layout of COATLI. The optical components of the instrument are numbered as follows. 1: entrance window (EW); 2: common path fold mirror (CFM); 3: collimator (COL); 4: tip-tilt mirror (TM); 5: dichroic (DIC); 6: blue channel fold mirror (BFM); 7: blue channel camera (BCAM); 8: blue channel filter (BF); 9/12: pupil relay (REL); 10/11: red channel filters (RF); 13: deformable mirror (DM); 14: red channel triplet camera (RCAM); 15: beam splitter (BS); and 16: pupil camera (PCAM). The color figure can be viewed online.

Fig. 2 2D layout of COATLI, where (a) shows a bottom view along the azimuthal axis and (b) shows a lateral view along the axis of the collimator.
Right before the red detector, there is a beam splitter (15) the first surface of which is uncoated and reflects 4% of the light towards a comercial Tamron 12VM1040ASIR lens (16) which produces an image of the pupil on a CMOS UI-1220-M-GL detector; this pupil camera is used for diagnostic and alignment purposes. The second surface of the beam splitter is coated in order to reduce the intensity of the pupil ghost and increase the efficiency in the red channel. In addition, the inclination and thickness of the beam splitter have been chosen so that the pupil image and its ghost do not overlap on the CMOS detector. The pupil image has a diameter of 0.86 mm or 140 pixels on the detector.
All lens groups except for the red camera (excluding the pupil camera lens, which is a comercial component) are non-cemented doublets of calcium fluoride (CaF2) and Ohara S-FTM16 (Brown et al. 2004). The optics workshop of the IA-UNAM (Instituto de Astronomía Universidad Nacional Autónoma de México) has experience in polishing the two materials for the near infrared instrument FRIDA (Cuevas et al. 2006). The red camera telephoto is N-BK7/CaF2/N-BK7 triplet. This group on its own produces a relatively high axial chromatic shift, however the red camera is not strongly powered and the chromatic shift induced by it is opposite to the residual chromatism left by all the previous optics. This leaves the red channel nearly apochromatic. No aspheric surfaces are present in the design, which will allow all the lenses to be manufactured in the optical workshops of the Instituto de Astronomía of the Universidad Nacional Autónoma de México (IA-UNAM). Table 1 shows the optical prescriptions of all the lenses of COATLI, where D is diameter, R is curvature radius, FL is focal length and CT is center thickness. Negative and positive focal lengths indicate divergent/convergent lenses and all units are milimeters.
Table 1 Optical prescription for the lenses of COATLI
Lens | Material | D | R1 | R2 | FL | CT |
---|---|---|---|---|---|---|
COL1 | S-FTM16 | 40 | 100.608 cx | 40.000 cc | -112.675 | 6.0 |
COL2 | CaF2 | 40 | 56.671 cx | 40.000 cx | 54.167 | 15.1 |
BCAM1 | CaF2 | 40 | 92.493 cx | 95.011 cx | 108.264 | 10.4 |
BCAM2 | S-FTM16 | 40 | 41.473 cc | 100.32 cx | -119.975 | 6.0 |
REL1 | CaF2 | 40 | 93.429 cx | 49.591 cx | 74.834 | 12.4 |
REL2 | S-FTM16 | 40 | 40.000 cc | 69.914 cx | -158.640 | 6.0 |
REL3 | S-FTM16 | 40 | 137.918 cx | 40.000 cc | -95.605 | 6.0 |
REL4 | CaF2 | 40 | 56.4254 cx | 40.000 cx | 54.069 | 15.1 |
RCAM1 | N-BK7 | 40 | 200.203 cc | 40.000 cc | -64.723 | 6.0 |
RCAM2 | CaF2 | 40 | 71.604 cx | 40.000 cx | 59.283 | 14.3 |
RCAM3 | N-BK7 | 40 | 111.771 cx | 41.564 cc | -128.462 | 6.0 |
There are three FLI CFW1 filter wheels each with eight holes for filters of 25 mm diameter: one in the blue channel, before the detector, with filters 5 mm thick; and two in the red channel, between the pupil transfer relay, with filters 3 mm and 5 mm thick respectively. The two red filter wheels will have one clear filter each to avoid the need to refocus when using one or the other.
3. Comparison to other astronomical instruments
The novelty of the design lies in the fact that the instrument needs to deliver diffraction-limited images in the visible, in a wide FoV and a relatively broad spectral range. To our knowledge, no similar design has ever been reported. Other astronomical instruments with adaptive optics (AO) usually work in the near infrared and have a smaller corrected field. (Although, for large telescopes, this may be equivalent in terms of the number of Airy discs within the field). Typically, these instruments use mirrors for their powered optics to avoid chromatic aberration, and have only one or none pupil planes, which simplifies enormously the optical design. Examples of these are PUEO at the CFHT (Arsenault et al. 1994), NAOS at the VLT (Rousset et al. 1998), the Lick AO system (Gavel et al. 2000), or PHARO at Palomar (Hayward et al. 2001), to mention a few. FRIDA, the future AO instrument for the GTC (Cuevas et al. 2006), has demonstrated the excellent performance of achromatic lens systems for a diffraction-limited instrument, for an equivalent FoV (40 arcsec in a 10.4 m telescope vs 4 arcmin in a 0.5 m telescope, with the same amount of pixels on the detector, and both instrument being Nyquist sampled), but as the previous, FRIDA will work in the near infrared. In contrast, VisAO for the Magellan Clay telescope (Kopon et al. 2010) is an example of an AO instrument in the visible, in a 6.5 m telescope. However, this is a direct imaging instrument with almost no optics, since the telescope’s secondary mirror acts as the adaptive element, which provides a very small corrected FoV of 8.4 arcsec.
4. Requirements and nominal performance
4.1. Instrument Requirements
The high level requirements of COATLI are summarized in Table 2. The image quality in the blue channel is given in terms of the ratio of the d 80 (this is the standard definition of the diameter that contains 80% of the energy from the image of a point source) of the instrument with the atmospheric contribution and the seeing limited case, and shall be lower than 1.25 (with a goal of better than 1.10). In the red channel, the Strehl Ratio shall be at least 0.7 (with a goal of better than 0.8). The field of view (FoV) of the two channels shall be at least 4 arcmin in diameter, and the plate scale shall be between 0.25 and 0.35 arcsec in the blue channel, and between 0.100 and 0.125 arcsec per pixel in the red channel.
Table 2 High level requirements of COATLI
Channel | Wavelength (nm) | Fov (arcmin) | Scale (arcsec/px) | Image quality |
---|---|---|---|---|
Blue | 400 - 550 | Diam ≥ 4 | 0.250 - 0.350 |
|
Red | 550 - 922 | Diam ≥ 4 | 0.100 - 0.125 | SR≥0.7 (goal:0.8) |
The requirements in FoV are specified for an appropriate coverage of the isokinetic angle, which is expected to be about 2 arcmin (Watson et al. 2016). The plate scales are specified with an upper limit given by the need to sample at the seeing limit in the blue channel, and to sample the best expected images of 0.25 arcsec FWHM in the red channel. The lower limits are to reduce the contribution of the detector noise when oversampling.
The requirements for image quality are given in terms of the Strehl Ratio
(SR) and the d80 ratio. The superscripts
D, S and I correspond to
the contributions of diffraction, seeing and the instrument’s optics including
the telescope, respectively, so that
4.2. Nominal Performance
Nominal performance refers to the instrument as designed, without taking into account tolerancing or thermal effects. The nominal scales are 0.29 arcsec/px at the blue detector and 0.12 arcsec/px at the red detector, with fields of view of 5 × 5 arcmin and 4.2 × 4.2 arcmin respectively. The respective working f ratios are f/18.2 and f/45. The spot diagrams for the broad bands g in the blue channel and riz in the red channel are shown in Figure 3. The nominal image quality for the red and blue channels is shown in Figure 4, following the criteria established in Table 2. In principle, there is no need for focus adjustment at the red detector for the different filters. However, in case of this being necessary, it can be done using the deformable mirror. From Figures 3 and 4, we see that the optical quality of the instrument is well within the requirements from § 4.1. Bands griz achieve the requirements and goals from Table 2 in the whole area of the detector. The red channel is clearly diffraction limited. For the blue channel, assuming that the seeing scales with the value of d 80, with a seeing of 0.6 arcsec we get a FWHM better than 0.63 arcsec in the g band.

Fig. 3 Spot diagram for the blue and red channels (above and below, respectively). We show the spot diagram for the g band filter in the blue channel, and the riz bands filters for the red channel. Diagrams for the center, edges and corners of the detector are shown. The circle is the Airy disk for a central wavelength in each band. The square grid has a size of 1 arcsec in all cases. The color figure can be viewed online.

Fig. 4 Image quality of the nominal design in the blue (left) and right (right) channels, following the criteria established in Table 2. We show the values at the center of the detector (solid), at the edge (dashed) and at the corners (dot-dashed).
The focusing of COATLI will be done as follows. The blue channel will be focused using the secondary mirror and the red channel will use the deformable mirror.
4.3. Efficiency
Filters and the dichroic are provided by Custom Scientific, as well as anti-reflecting coatings for all the lenses and the second surface of the beam splitter for the pupil camera. Given the relatively short wavelength range, transmissivity will be at least 99% per surface per lens. The requirements of COATLI define that the mean efficiency shall be greater than 35%, 35%, 25% and 15% respectively in the griz bands, excluding only the atmosphere and the central obturation of the telescope. The transmission curves for the five broad band filters and dichroic, and the estimated total efficiency for the two channels of COATLI are shown in Figure 5. This efficiency estimate takes account of the telescope, all the optics with coatings (lenses, mirrors, windows and filters) and the detectors.

Fig. 5 Transmission coefficient of the griz filters and the dichroic of COATLI (left) and efficiency estimation for the blue and red channels (right). In the right plot, we show the efficiency of the telescope and instrument without filters nor detectors (black dotted), without filters but with detectors (black solid) and with filters griz and detectors (color solid). The color figure can be viewed online.
5. Active optics correction
The deformable mirror, located at the second pupil plane in the red channel path, will be able to correct for focus, astigmatism and coma. The aberrations will be measured using the donut method (Tokovinin and Heathcote 2006), which consists of fitting a model of a defocused aberrated beam to an out-of-focus image on the detector. The deformable mirror itself will be able to produce the desired defocus. The stroke of the deformable mirror corresponding to defocus, astigmatism and coma was measured at the facilities of the IA-UNAM, using a Zygo GPI-6” Fizeau laser interferometer, applying a modal deformation of the mirror’s actuators. Table 3 shows the values of the Zernike coefficients corresponding to the maximum stroke of the deformable mirror for defocus, astigmatism and coma, at a voltage of 240 V. Astigmatism can be applied independently from coma and defocus, since it uses the outer electrodes of the deformable mirror. Coma and defocus have to be applied sharing the inner electrodes of the deformable mirror.
Table 3 Zernike coefficients at the deformable mirror
Coefficient | Mode | DM stroke (nm RMS) |
---|---|---|
Z4 | defocus | 1352 |
Z5 | astigmatism | 508 |
Z6 | astigmatism | 503 |
Z7 | coma | 204 |
Z8 | coma | 187 |
Figure 6 shows the Zernike coefficients Z5 and Z8 (representative for astigmatism and coma) in wavefront error (WFE) RMS on the deformable mirror induced by astigmatism (Z5) on the surfaces of the telescope’s primary mirror (M1) and secondary mirror (M2) and misalignments of M2. As an example, we could correct a maximum of 254 nm of Z5 RMS on M1, ora maximum of 325nm of Z5RMS on M2, or a combination of the two; and a maximum of 1.1 mm of misalignment of M2 (assuming no focus correction).
6. Tolerancing
The tolerancing study was made through 200 Monte Carlo simulations where all the relevant optical and optomechanical parameters were varied following a parabolic statistic within a given range, specified individually for each parameter. The range for some of the parameters is given by the manufacturer, some others depend on the capabilities of the workshops and laboratories at the IA-UNAM, some parameters are more sensitive than others, etc. Also, some of the tolerances come from, or induce, restrictions in the optomechanics, which has also been designed at the IA-UNAM, and will be manufactured in our mechanics workshops. The rest of the parameters are either restrictions of the optics workshops at the IA-UNAM (such as tolerances in radius and thicknesses of the lenses), of the laboratory measurement procedures (such as tolerances in parallelism and surface irregularity), or have been evaluated in order not to degrade image quality below specifications.
The tolerances are shown in Table 4. In the table, from left to right, we show the tolerances in: refractive index (n), curvature radius of each surface of the lens (r), thickness (t), position along the optical axis (z), decentering (∆y), inclination (α), surface inclination or parallelism (p) and surface irregularity (irr). The table is divided in three sections, the first corresponding to the lens parameters, the second to mirrors and the third to windows, filters and/or plates. The labels COL1+2, BCAM1+2, REL1+2, REL3+4 and RCAM1+2+3 refer to groups of lenses in their optomechanical barrel. The parameters with a dagger superscript are tolerances specified by an external manufacturer. While tolerances in some parameters are more restrictive in some elements than in others, when the difference is small we have maintained the more restrictive value for all the equivalent elements.
Table 4 Summary of the tolerances of the instrument
Element | n | r (mm) | t (mm) | z (mm) | ∆y (mm) | α (◦) | p (◦) | irr (fringes) |
---|---|---|---|---|---|---|---|---|
COL1 | ±5 × 10 −5† | ±0.10 | ±0.10 | ±0.10 | ±0.03 | ±0.04 | ±0.03 | ±1 |
COL2 | ±5 × 10 −5† | ±0.10 | ±0.10 | ±0.05 | ±0.03 | ±0.04 | ±0.03 | ±1 |
COL1+2 BCAM1 | ±5 × 10 −5† | ±0.10 | ±0.10 | ±0.10 | ±0.10 ±0.03 | ±0.10 ±0.04 | ±0.03 | ±1 |
BCAM2 | ±5 × 10 −5† | ±0.10 | ±0.10 | ±0.05 | ±0.03 | ±0.04 | ±0.03 | ±1 |
BCAM1+2 REL1 | ±5 × 10 −5† | ±0.10 | ±0.10 | ±0.10 | ±0.10 ±0.03 | ±0.10 ±0.04 | ±0.03 | ±1 |
REL2 | ±5 × 10 −5† | ±0.10 | ±0.10 | ±0.05 | ±0.03 | ±0.04 | ±0.03 | ±1 |
REL1+2 REL3 | ±5 × 10 −5† | ±0.10 | ±0.10 | ±0.10 | ±0.10 ±0.03 | ±0.10 ±0.04 | ±0.03 | ±1 |
REL4 | ±5 × 10 −5† | ±0.10 | ±0.10 | ±0.05 | ±0.03 | ±0.04 | ±0.03 | ±1 |
REL3+4 RCAM1 | ±5 × 10 −5† | ±0.10 | ±0.10 | ±0.10 | ±0.10 ±0.03 | ±0.10 ±0.04 | ±0.03 | ±1 |
RCAM2 | ±5 × 10 −5† | ±0.10 | ±0.10 | ±0.05 | ±0.03 | ±0.04 | ±0.03 | ±1 |
RCAM3 | ±5 × 10 −5† | ±0.10 | ±0.10 | ±0.10 | ±0.10 | ±0.10 | ±0.03 | ±1 |
RCAM1+2+3 | ±0.10 | ±0.10 | ||||||
CFM | ±0.05 | ±0.10 | ±0.10† | |||||
TM | ±0.50 | ±0.10 | ±0.20† | |||||
BFM | ±0.50 | ±0.10 | ±0.10† | |||||
DM | ±0.50 | ±0.10 | ||||||
EW | ±0.10† | ±1.00 | ±1.00 | ±0.002† | ±0.20† | |||
DIC | ±0.10† | ±0.50 | ±1.00 | ±0.017† | ±0.17 / ±0.33 | |||
BF | ±0.10† | ±1.00 | ±1.00 | ±0.017† | ±0.50 | |||
RF | ±0.10† | ±1.00 | ±1.00 | ±0.017† | ±0.33 | |||
BS | ±0.10† | ±0.50 | ±1.00 | ±0.008† | ±0.10† |
The tolerances in tilt and misalingment of the optical components are compatible with the optomechanical design (Cuevas et al. 2016).
The tolerancing study uses two types of static compensators. In the blue channel we compensate with the position of the focal plane, and in the red channel we compensate with the position and the inclination of the focal plane. From the Monte Carlo simulations mentioned above, the maximum defocus in 90% of the cases was ±3 mm for the blue detector and ±20 mm for the red detector, with a maximum inclination of ±7° at the red detector. To compensate the defocus in the two channels, we have left the mechanical distance between the last two lenses of each camera free within a certain adjustment, so these distances will only be fixed when we have manufactured all the optics and we know their dimensional parameters such as curvature radii and thicknesses. According to the defocus obtained by the tolerancing study, these two distances will be left to adjustment within ±1.5 mm for the blue channel and ±5 mm for the red channel. Further fine tuning of the detector focus and tilt (in case of the red detector) will be made by measuring and manufacturing an appropriate mount for each detector.
To evaluate image quality degradation after the tolerancing study, we isolated and analyzed the 90th percentile of the Montecarlo simulations. (Hence we have a chance of 9 out of 10 to have a better image quality than this). The image quality is shown in Figure 7. Taking tolerances into account, the optical quality of the instrument remains within the requirements from Table 2. In the blue channel, band g achieves the requirements and goals inside a field of 5 arcmin in diameter, and the requirements in the whole area of the detector (including corners). In the red channel, bands riz achieve the goals in a field of 4.2 arcmin in diameter. The axial positions of the pupil planes shift from their nominal positions a maximum of ±1 mm for the tip-tilt mirror and ±0.5 mm for the deformable mirror. These are acceptable values to ensure the correct performance of the fast guiding and active optics systems.
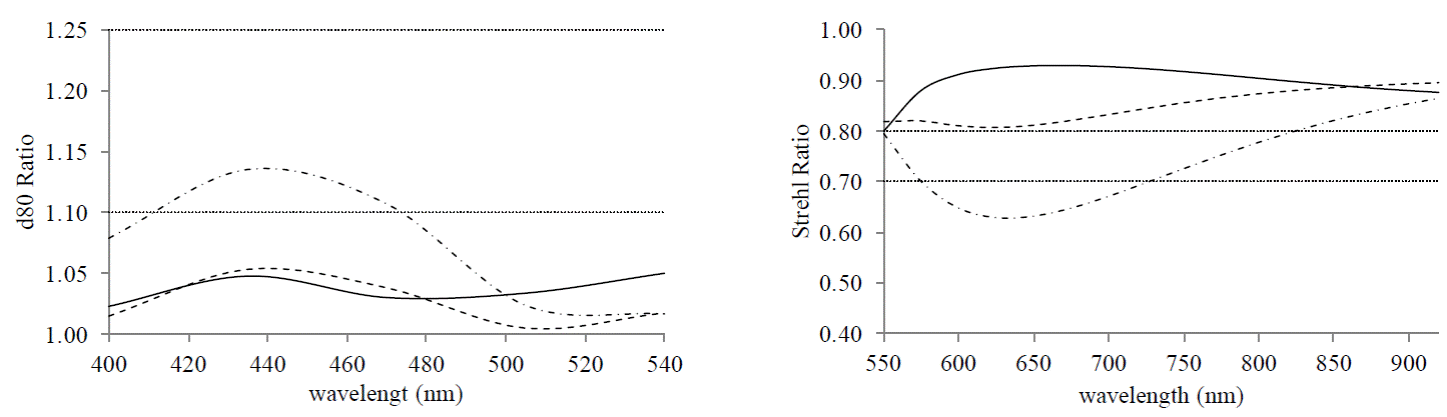
Fig. 7 Image quality in the red (left) and blue (right) channels, including tolerances, following the criteria stablished in Table 2. We show the values at the center of the detector (solid), at the edge (dashed) and at the corners (dot-dashed). In the blue channel, we show the g band (400-540 nm) and the B band (370-510 nm), refocusing with M2 for the latter.
7. Thermal behavior and ghosts
COATLI will operate on Sierra San Pedro Mártir at an altitude of 2790 m with typical pressures of 0.72 atm. Temperatures throughout the year range from -15 to 30 C. The optics of COATLI have been designed at an intermediate temperature of 5 C. The optomechanics have been designed so that the optics will not move beyond the tolerances listed above in the given temperature range, and a detailed thermal analysis in Zemax has shown that the instrument can be compensated by focusing with the secondary mirror of the telescope and the deformable mirror, while not affecting the image quality.
We have analyzed light at the blue and red detectors coming from secondary reflections in all the optical components of the instrument using a Zemax non-sequential model. No ghosts were found at the blue detector. On the other hand, internal reflections in the red filters and the beam splitter produce ghosts at the red detector. However, these are approximately five orders of magnitude weaker than the primary image.
Masks will be placed at all intermediate focal planes (telescope’s focal plane, pupil relay focal plane, before the blue filter wheel and before the red detector) and pupil planes (on the tip-tilt mirror and on the deformable mirror window), in order to reduce stray light.
8. Further science with the B band
In addition to what has been presented, there are some scientific cases which require following stars in the B band, but do not need a very good image quality. The image quality in the design of COATLI in the B band is significantly worse than in the g band due to chromatic aberration, and the instrument has not been optimized for the B band. However, we have checked that the image quality in the B band is acceptable by refocusing with the telescope’s secondary mirror, delivering a FWHM better than 0.75 arcsec inside a field of view of arcmin.
9. Conclusions
COATLI will produce images at the diffraction limit of a small telescope in the visible, in a wide field of view (4 arcmin) and in a relatively broad spectral range (550 to 920 nm), even in relatively poor seeing, with the only drawback of the telescope being relatively small (50 cm in diameter). In these terms, while being based on a standard collimatorcamera system using lens systems, the optical design has no precedent in astronomical instrumentation. The solution that we present here is compact, lowrisk, and offers an excellent performance.