1 Introduction
The break-up effect observed in many nuclear systems induced by loosely bound nuclei 6Li, 7Li and 9Be, and by exotic nuclei 6He (2n-halo nucleus), 8He (neutron skin nucleus), 11Be, and 12Be scattered by different targets attracted a plenary attention for decades. One of the most interesting nuclear systems where break-up effect could be observed is 6Li+208Pb system. Extensive experimental [1,2,3,4,5,6,7,8,9,10] and theoretical [11,12,13,14,15,16,17,18,19,20,21,22,23,24,25] studies were and still are being devoted to investigate 6Li+ 208Pb nuclear system at various energies both near and above the Coulomb barrier energy E c . Chun-Lei et al.[1] measured the elastic scattering angular distributions for 6Li+ 208Pb system at energies 25, 27, 29, 31, 33, 35, 37, 41, 43, and 46 MeV. These data were analyzed from the phenomenological point of view using optical model (OM). The extracted real and imaginary potential depths showed pronounced energy dependence. The behavior of the extracted potential is found to be quite different in comparison with other systems such as 19F, 16O+ 208Pb. This anomaly is a signature of break-up effect resulting from weak binding nature of 6Li and hence has obvious effect on optical potentials. Keeley et al., [2] measured the 6Li+ 208Pb elastic scattering angular distributions in the energy range Elab =25-39 MeV. The measured differential cross sections were analyzed using a real part derived based on double folding (DF) model in addition to an imaginary part of the conventional Woods-Saxon (WS) shape. The main finding of this study was the necessity to reduce the strength of the real folded potential by 43 % in order to reasonably reproduce the data, which is consistent with a repulsive polarization potential arising from the effects of break-up coupling to the continuum. In Ref. [3], the 6Li+ 208Pb angular distributions were measured in the energy range 23-48 MeV in order to find a unique description of the sequential d + α break-up of 6Li in the field of heavy target nuclei. The measured data were analyzed using central nuclear potential of three parts: real and imaginary volume terms in addition to an imaginary surface term each of WS shape. In Ref. [4], the authors tried to provide optical model parameters for the scattering of 6Li at energy 73.7 MeV on different medium and high mass targets: 58Ni, 90Zr, 124Sn, and 208Pb. The dependence of the potential depths on the Z and A of the considered targets and on the bombarding energy was investigated. Fulmer et al. [5] measured the angular distributions for 88-MeV 6Li ions elastically scattered from eleven targets ranging in mass from 24Mg to 208Pb. The elastic data were analyzed using the optical model, with potentials of both WS and DF forms. The analysis confirmed that the potentials for 6Li obtained from the DF model with the M3Y interaction need renormalizing by about 0.6. In Ref. [6], the differential cross section angular distributions for 99-MeV 6Li ions elastically scattered from 12C, 28Si, 40Ca, 58Ni, 90Zr, and 208Pb targets were measured. By increasing the target mass, the angular distributions showed progressively less structure which is characteristic of strongly absorbed particles. The analyzed data using OM exhibited both discrete and continuous ambiguities. The data also were analyzed using single folding 6Li potential based on d + α cluster model for 6Li, a satisfactory description of the data was obtained only after renormalization of the real potential by a factor of 0.5. J. Cook et al. [7] measured the elastic scattering angular distributions for 156- MeV 6Li ions from 12C, 40Ca, 90Zr, and 208Pb. The data were analyzed using various potential forms established by using WS, WS squared and density independent folded potentials. The data analysis emphasized the necessity to reduce the real folded potential strength by about 21-44 % according to the target mass. The experimental measurements were continued to test this behavior for nuclear reactions induced by 6Li at higher energies. In Ref. [8], the differential cross sections for the elastic scattering of 210- MeV 6Li ions on different target nuclei “12C, 28Si, 40Ca, 58Ni, 90Zr, and 208Pb”were measured. Most of the measured data were extended sufficiently into the rainbow region to enable the extraction of unique 6Li potentials. For the 208Pb target, Coulomb scattering dominates with only a slight evidence of nuclear diffraction at the largest angles. The data were analyzed in terms of a six-parameter phenomenological OM potential with WS form factors. The obtained unique potentials showed a weak target-mass dependence, which allowed the prediction of 208Pb potential parameters.
Furthermore, it is worth to underline that recent experimental evidences for Li-isotopes and a few of similar nuclei lying in the nuclide-chart close to 6Li seem to show the behavior looks similar [26,27] or at most with small differences, to the above described one, i.e. with enhancement of nuclear effects at large angles, mainly at energy around the Coulomb barrier. A partial response to the interesting question which could arise, if and how structure effects, such as in particular cluster configuration, could play a role in this respect for close nuclides in this region, can be found in [28] .
In addition to these extensive measurements, different theoretical studies [11,12,13,14,15,16] were also devoted to investigate 6Li+nucleus potential and the break-up effect of 6Li into d + α on the elastic scattering data. Yongli Xu et al. 11] established a systematic global phenomenological OM potential for 6Li projectile by studying the experimental data of elastic scattering angular distributions and reaction cross sections from 24Mg to 209Bi below 250 MeV. Based on the obtained 6Li global phenomenological OM, the theoretical calculations using the global OM potential was consistent with a large body of elastic-scattering data. In Ref. [14], the angular distributions for 6,7Li elastically scattered from 12C, 28Si, 40Ca, 58Ni, 90Zr and 208Pb targets at 12.5 — 53 MeV /u were analyzed utilizing a real part of potential constructed using Jeukenne, Lejeune and Mahaux (JLM) method and a Gaussian shape of the effective NN nucleon-nucleon interaction. An energy dependent reducing renormalization coefficient was required for the real component of the JLM optical potential in order to obtain successful predictions of the observed cross sections even when the projectile density-dependence was considered.
Several microscopic studies investigated the break-up effect in 6Li projectile [17,18,19,20,21,22,23,24,25], Watanabe et al. [17] investigated break-up dynamics in 6Li elastic scattering on 208Pb at E = 39 MeV using both three-body channel (6Li + 208Pb → d + α + 208Pb) and four-body channel (6Li + 208Pb → n + p + α + 208Pb). Both channels are precisely treated with the four-body version of the continuum-discretized coupled-channels method (four-body CDCC). The main finding of this study is that 6Li break-up is mainly induced by a three-body channel. In Ref. [24], elastic scattering of 6Li from various targets: 12C, 28Si, 58Ni, 120Sn and 208Pb nuclei at various incident energies up to 100 MeV per nucleon was investigated by the CDCC method based on a double folding model of the 6Li nucleus interaction with a realistic energy and density dependent NN interaction, called DDM3Y. The 6Li projectile break-up effect is found to diminish with increasing incident energies.
The current study aims to investigate the available 6Li+ 208Pb elastic scattering angular distributions in a wide energy range and at energies both near and above the Coulomb barrier energy E c using different potentials, and to observe the break-up effect of 6Li into d + α on the 6Li+ 208Pb elastic scattering data. This work supplements our previous studies for different nuclear systems induced by the weakly bound 6Li ions [29,30,31,32]. The paper is organized as follows. Section 2 demonstrates the different potentials used in theoretical calculations. Section 3 is devoted to data analysis, results and discussion. Summary is given in Section 4.
2 Theoretical methods
The elastic scattering angular distributions fo6r Li + 208Pb nuclear system in the energy range 25 - 210 MeV are subjected to detailed theoretical analysis using different potentials created based on phenomenological, semi-microscopic, and microscopic models. The current study aims to obtain the global potential that fairly reproduce the experimental data in this wide range of energies and to observe how the interaction mechanism differ as we go from near barrier energy to the region of relatively higher energies. In addition, the 6Li break-up effect was observed on the elastic scattering data as well as the various incorporated models.
2.1 Phenomenological OM potential
The data on elastic scattering were firstly analyzed from the phenomenological point of view within the framework of the standard optical model of the nucleus, where the influence of inelastic channels is taken into account by introducing a phenomenological imaginary absorptive part in the interaction potential between the two colliding nuclei. In this model the elastic scattering is described by a complex interaction potential with a radial dependence in the form of WS. For both the real and imaginary parts of the potential, the WS shape is taken in addition to the Coulomb potential of a uniformly charged sphere. So, the utilized interaction potential can be written as:
with radius
Parameters of optical potential (OP) were selected to achieve the best agreement between theoretical and experimental angular distributions. The
where N is the number of data points. The quantities
2.2 Sao Paulo potential
According to the different parameter ambiguities both discrete and continuous associated with the OM calculations, and the fact that phenomenological representations do not include a description of the projectile or target’s structure, the real part of potential was constructed using the microscopic double folding (DF) procedure extracted from the Sao Paulo potential (SPP) via the double convolution integral as described in Refs. [34,35,36].
where
In this model, the following two equations link the real part of the local-equivalent interaction to the DF potential V F (R) as
where V is the local relative velocity between the two nuclei and C is the speed of light. The new version of the Sao Paulo potential (SPP2) was calculated using the REGINA code [37] with nuclear densities obtained from the Dirac-Hartree-Bogoliubov model [38].
2.3 Cluster folding potential
The 6Li + 208Pb elastic scattering data are analyzed from microscopic point of view using the cluster folding model (CFM). The importance of various break-up mechanisms in nuclear systems induced by 6Li is of special interest due to its very low binding energy and the dissociation into α (core) + d (valence). Based on the cluster nature of 6Li, we describe the 6Li + 208Pb elastic scattering angular distributions using CFM where, both the real and imaginary parts of potential are created based on cluster folding. The real and imaginary cluster folding parts of 6Li + 208Pb potential can be defined based on α + 208Pb and d + 208Pb potentials as:
where (
3 Results and discussion
3.1 6Li + 208Pb data analysis using phenomenological OM potential
Within the framework of OM, the available angular distributions for 6Li elastically scattered from 208Pb in the energy range 25 - 210 MeV [1,2,3,4,5,6,7,8] have been reanalyzed. The potential parameters considered by C. Fulmer et al.
[5] for both the real and imaginary parts of potential are taken as starting parameters. The utilized central potential consists of Coulomb part as well as nuclear part of real and imaginary volume terms each of WS shape. In accordance with previous studies concerning nuclear processes induced by 6Li -projectile, the influence of spin orbit potential (V
so
) for 6Li is little and its effect can be excluded. Data are fitted using four varying parameters, namely, depth and diffuseness for the real (V
0
and a
V
) and imaginary (W
0 and a
W
) parts of the potential. Where, the radius parameters for the real (r
V
) and imaginary (r
W
) parts was fixed at 1.3 fm and 1.7 fm, respectively. The agreement between the experimental 6Li + 208Pb elastic scattering angular distributions and the theoretical calculations using OM is fairly good at the different considered energies as shown in Figs. 2-4. The extracted optimal OM parameters are listed in Table I, real (J
V
) and imaginary (J
W
) volume integrals as well as total reaction cross section
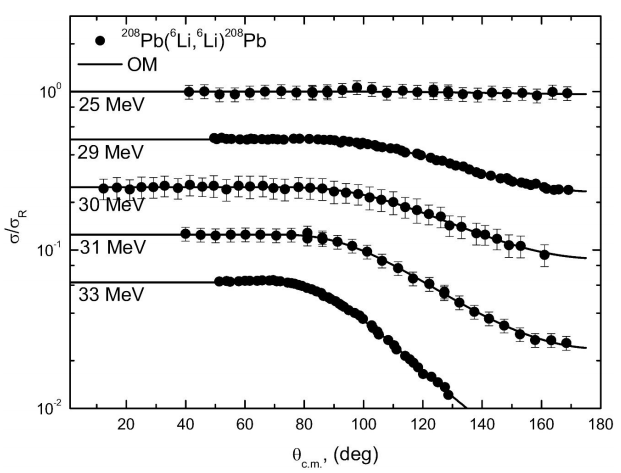
Figure 2 Comparison between 208Pb(6Li, 6Li) 208Pb elastic scattering angular distributions (solid circles) and OM (solid curves) fits at E lab = 25, 29, 30, 31, and 33 MeV. The data are displaced by 0.5 for the sake of clarity.
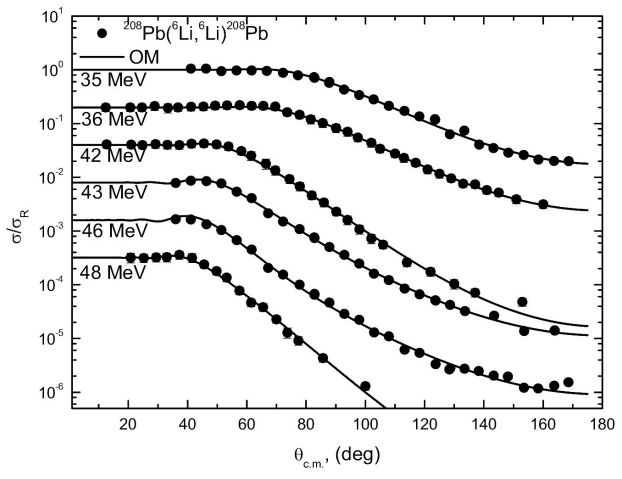
Figure 3 Same as Fig. 2 but at E lab = 35, 36, 42, 43, 46 and 48 MeV. The data are displaced by 0.2 for the sake of clarity.

Figure 4 Same as Fig. 2 but at E lab = 7307, 88, 99, 156 and 210 MeV. The data are displaced by 0.2 for the sake of clarity.
Table I Global potential parameters extracted from the OM analysis for the 6Li + 208Pb nuclear system with fixed rV = 1.3 fm and rW = 1.7 fm. Real and imaginary volume integrals as well as reaction cross-sections are also listed.
E (MeV) | V0 (MeV) | av (fm) | W0 (MeV) | aw (fm) |
|
|
Jv (MeV.fm3) | Jw (MeV.fm3) |
---|---|---|---|---|---|---|---|---|
25 | 259.9 | 0.73 | 5.93 | 0.749 | 0.05 | 10.16 | 433.96 | 21.43 |
29 | 217.49 | 0.73 | 14.8 | 0.749 | 2.08 | 222.9 | 363.14 | 53.50 |
30 | 166.42 | 0.73 | 13.82 | 0.749 | 0.016 | 299.4 | 277.87 | 49.59 |
31 | 215.36 | 0.73 | 14.87 | 0.749 | 0.044 | 439.4 | 359.58 | 53.75 |
33 | 201.83 | 0.73 | 11.86 | 0.749 | 9.14 | 618.3 | 336.99 | 42.87 |
35 | 216.02 | 0.73 | 10.08 | 0.749 | 0.94 | 807.7 | 360.69 | 36.43 |
36 | 176.71 | 0.73 | 11.52 | 0.749 | 0.09 | 922.2 | 295.05 | 41.64 |
42 | 178.45 | 0.73 | 14.49 | 0.749 | 0.38 | 1500 | 297.96 | 52.37 |
43 | 164.51 | 0.834 | 26.0 | 0.534 | 0.54 | 1419 | 281.47 | 91.65 |
46 | 164.58 | 0.849 | 22.01 | 0.489 | 2.24 | 1528 | 251.59 | 77.25 |
48 | 201.48 | 0.73 | 19.09 | 0.749 | 0.36 | 1982 | 336.41 | 69.01 |
73.7 | 88.24 | 0.819 | 10.39 | 0.831 | 0.37 | 2726 | 150.42 | 37.97 |
88 | 75.46 | 0.811 | 9.49 | 0.939 | 2.8 | 3085 | 128.39 | 35.22 |
99 | 93.52 | 0.836 | 12.41 | 0.781 | 0.414 | 3098 | 160.09 | 45.05 |
156 | 41.27 | 0.93 | 11.53 | 0.852 | 0.95 | 3480 | 72.37 | 42.26 |
210 | 56.09 | 0.93 | 11.02 | 0.767 | 3.08 | 3428 | 98.36 | 39.93 |
As shown from Figs. 2-4, 6Li + 208Pb data show unmistakable Coulomb rainbow phenomenon which results in the so called Fresenl peak. It is pronounced that the position of this peak is shifted toward smaller angles with increasing the bombarding energy. At lowest energy 25 MeV which is very close to Ec, this peak is not presented and it starts to be clearly appear at E > 33 MeV which is slightly above Ec.
3.2 6Li + 208Pb data analysis using Sao Paulo potential
The 6Li + 208Pb elastic scattering angular distributions are then analyzed semi-microscopically using SPP. Two approaches were used: in the first approach, the real part of the potential was derived using SPP and the imaginary part was taken as a factor times the real SPP. In other words, the calculations were performed using two free parameters, namely, NRSPP (renormaliztion factor for the real part created based on SPP) and NI SPP (renormaliztion factor for the imaginary part). The total potential in this case has the following form:
As shown in Figs. 5-7, the agreement between the experimental 6Li + 208Pb angular distributions at energies 25 - 210 MeV and the calculations using real and imaginary SPP is fairly good. The optimal extracted potential parameters using this approach are listed in Table II, (J
v), (Jw), and

Figure 5 Comparison between 208Pb(6Li, 6Li) 208Pb elastic scattering angular distributions (solid circles) and SPP (solid curves) fits at Elab= 25, 29, 30, 31, and 33 MeV. The data are displaced by 0.5 for the sake of clarity.
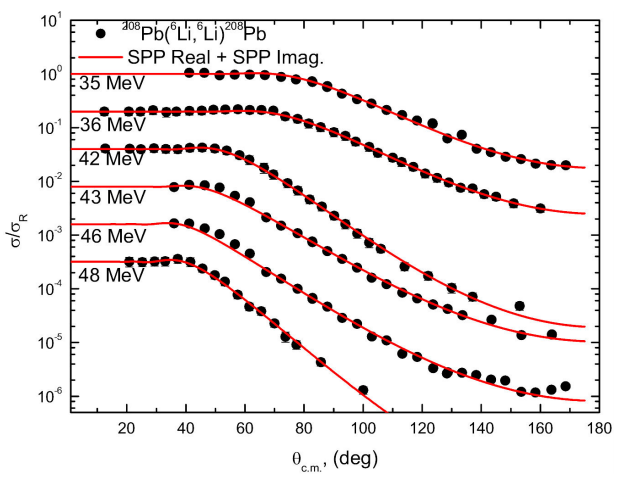
Figure 6 Same as Fig. 5 but at Elab = 35, 36, 42, 43, 46 and 48 MeV. The data are displaced by 0.2 for the sake of clarity.
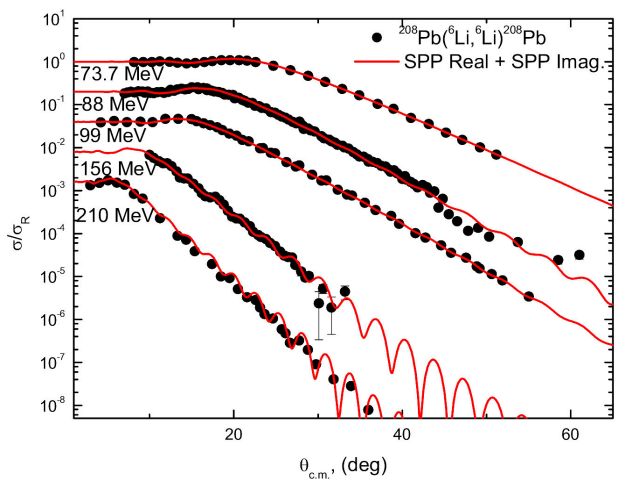
Figure 7. Same as Fig. 5 but at Elab = 73.7, 88, 99, 156 and 210 MeV. The data are displaced by 0.2 for the sake of clarity.
Table II Optimal potential parameters extracted from 6Li + 208Pb analysis using SPP for both real and imaginary parts. Real and imaginary volume integrals as well as reaction cross-sections are also listed.
E (MeV) | NRSPP | NISPP |
|
|
JV (MeV.fm3) | JW (MeV.fm3) |
---|---|---|---|---|---|---|
25 | 0.927 | 0.187 | 0.05 | 8.556 | 377.41 | 76.13 |
29 | 0.522 | 0.736 | 2.07 | 226.5 | 211.62 | 298.38 |
30 | 0.42 | 0.694 | 0.017 | 303.0 | 170.09 | 281.05 |
31 | 0.518 | 0.752 | 0.044 | 441.8 | 209.55 | 304.21 |
33 | 0.49 | 0.615 | 8.91 | 619.5 | 197.80 | 248.26 |
35 | 0.524 | 0.521 | 0.97 | 802.2 | 211.08 | 209.87 |
36 | 0.452 | 0.578 | 0.08 | 912.1 | 181.88 | 232.58 |
42 | 0.45 | 0.697 | 0.26 | 1468 | 179.93 | 278.69 |
43 | 0.172 | 1.478 | 1.12 | 1779 | 68.70 | 590.34 |
46 | 0.104 | 2.245 | 3.23 | 2160 | 41.41 | 893.85 |
48 | 0.478 | 1.00 | 0.29 | 1973 | 189.41 | 396.25 |
73.7 | 0.541 | 0.58 | 0.63 | 2610 | 209.17 | 224.25 |
88 | 0.576 | 0.428 | 4.1 | 2715 | 219.37 | 163.01 |
99 | 0.515 | 0.574 | 0.21 | 2941 | 189.25 | 210.93 |
156 | 0.537 | 0.467 | 1.48 | 3115 | 190.48 | 165.65 |
210 | 0.568 | 0.599 | 15.4 | 3320 | 190.56 | 200.96 |
The extracted NRSPP value at the different considered energies is close to each other with an average value 0.507 ± 0.05 except at energies 25, 43, and 46 MeV. At E = 25 MeV which is less than to E C , the N RSPP value is 0.927. At energies 43 and 46 MeV, an anomaly is observed where the N RSPP value is 0.172, and 0.104 respectively. These results clearly show that, in order to reproduce the 6Li + 208Pb data, the strength of the real SPP should be reduced by about 49 %.
This reduction is essential to reproduce the experimental data for nuclear system induced by weakly bound nuclei such as 6Li, 7Li, and 9Be.
The energy dependence on the obtained values for both the real JV and imaginary JW volume integrals from SPP calculations is illustrated in Fig. 8, which is based on the obtained values listed in Table II. The behavior deduced was fitted using the following equation:
With a = 269.09(49.1), b = -1186.6(21964.57), c = 303905.12(-489825.38) for real and imaginary volume integral respectively.
In the second approach, the real part of the potential was derived using SPP exactly as in the first approach in addition to an imaginary volume part in WS form to simulate the reduction in flux due to absorption, the radius parameter for the imaginary volume term was fixed at 1.7 fm similar to OM calculations. So, the calculations were performed using three parameters, namely, NRSPP (renormaliztion factor for the real part created based on SPP), depth (W0) and diffuseness (a W) of the imaginary volume part. The total potential in this case has the following form:
As shown in Figs. 9-11, the agreement between the experimental 6Li + 208Pb angular distributions at energies 25 - 210 MeV and the calculations using real SPP and an imaginary WS potential is fairly good. The optimal extracted potential parameters using this approach are listed in Table III, (JV), (JW), and

Figure 9 Comparison between 208Pb(6Li, 6Li) 208Pb elastic scattering angular distributions (solid circles) and SPP for the real part +WS imaginary part (solid curves) fits at E lab = 25, 29, 30, 31, and 33 MeV. The data are displaced by 0.5 for the sake of clarity.
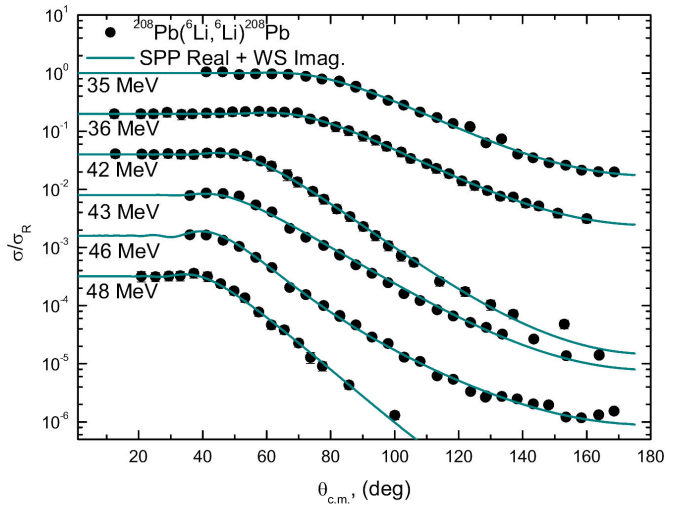
Figure 10 Same as Fig. 9 but at Elab = 35, 36, 42, 43, 46 and 48 MeV. The data are displaced by 0.2 for the sake of clarity.

Figure 11 Same as Fig. 9 but at Elab = 73.7, 88, 99, 156 and 210 MeV. The data are displaced by 0.2 for the sake of clarity.
Table III Optimal potential parameters extracted from 6Li + 208Pb analysis using SPP for the real part +WS imaginary part. Real and imaginary volume integrals as well as reaction cross-sections are also listed.
E (MeV) | NRSPP | W0 (MeV) | aW (fm) |
|
|
JV (MeV.fm3) | JW (MeV.fm3) |
---|---|---|---|---|---|---|---|
25 | 0.993 | 3.844 | 0.747 | 0.05 | 6.842 | 404.28 | 13.89 |
29 | 0.748 | 13.19 | 0.703 | 11.6 | 184.9 | 303.25 | 47.39 |
30 | 0.362 | 13.84 | 0.749 | 0.017 | 299.5 | 146.60 | 50.02 |
31 | 0.523 | 14.85 | 0.732 | 0.05 | 422.5 | 211.57 | 53.55 |
33 | 0.489 | 13.59 | 0.714 | 8.4 | 607.6 | 197.39 | 48.90 |
35 | 0.428 | 9.84 | 0.788 | 0.87 | 833.9 | 172.41 | 35.75 |
36 | 0.482 | 12.26 | 0.696 | 0.07 | 878.4 | 193.95 | 44.01 |
42 | 0.398 | 13.7 | 0.745 | 0.43 | 1470 | 159.14 | 49.49 |
43 | 0.267 | 25.9 | 0.695 | 2.47 | 1636 | 106.65 | 92.97 |
46 | 0.799 | 22.17 | 0.488 | 2.21 | 1526 | 318.12 | 77.81 |
48 | 0.445 | 19.09 | 0.749 | 0.37 | 1978 | 176.33 | 69.01 |
73.7 | 0.433 | 10.71 | 0.799 | 0.41 | 2694 | 167.42 | 38.97 |
88 | 0.413 | 10.14 | 0.889 | 3.2 | 3048 | 157.29 | 37.37 |
99 | 0.395 | 12.32 | 0.798 | 0.43 | 3115 | 145.15 | 44.82 |
156 | 0.569 | 14.69 | 0.863 | 1.39 | 3681 | 201.83 | 53.93 |
210 | 0.324 | 14.96 | 0.893 | 8.8 | 3833 | 108.70 | 55.16 |
3.3 6Li + 208Pb data analysis using CF potential
Motivating by the well-known d + α cluster structure for 6Li, we tried to reproduce the available experimental data for 6Li + 208Pb elastic scattering angular distributions using the fully microscopic cluster folding (CF) model. Within the framework of this model, the real and imaginary parts of potential ware constructed based on CF potential (Eqs. 6 and 7). The total potential in this case has the following form:
The comparisons between the experimental 6Li + 208Pb elastic scattering angular distributions in the energy range 25 - 210 MeV [1, 2, 3, 4, 5, 6, 7, 8] and the theoretical calculations within the framework of the CF model are shown in Figs. 12-14, with potential parameters listed in Table IV. The data are fitted using two parameters - NR CF and NICF - renormalization factor for the real and imaginary CF potentials. To obtain good fitting with the experimental data, the strength of the real cluster folding potential should be reduced by ∼ 62 %. In general, the observed reduction in the strength of the real part created based on either CF or DF is one of the signatures for 6Li+X nuclear systems. The extracted average NRCF is 0.378 ± 0.139 from our CFM calculations. At energies 43 and 46 MeV, the data require more reduction in the strength of the real CF potential since the extracted NRCF = 0.1 at these two aforementioned energies. Such anomaly was also observed in the calculations using SPP first approach.
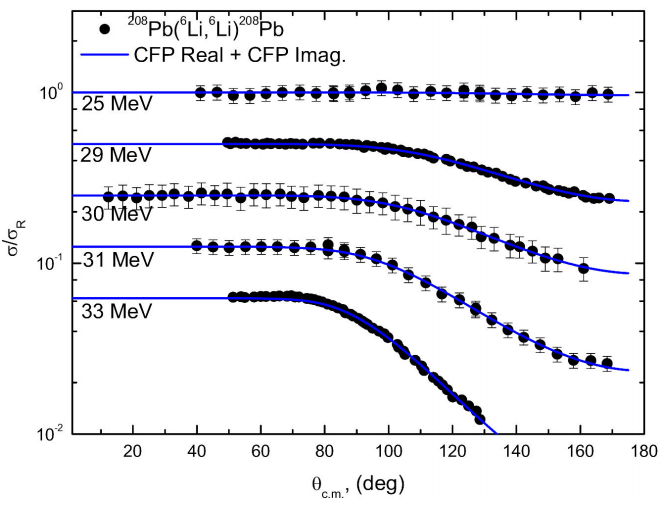
Figure 12 Comparison between 208Pb(6Li, 6Li) 208Pb elastic scattering angular distributions (solid circles) and CFP (solid curves) fits at Elab = 25, 29, 30, 31, and 33 MeV. The data are displaced by 0.5 for the sake of clarity.
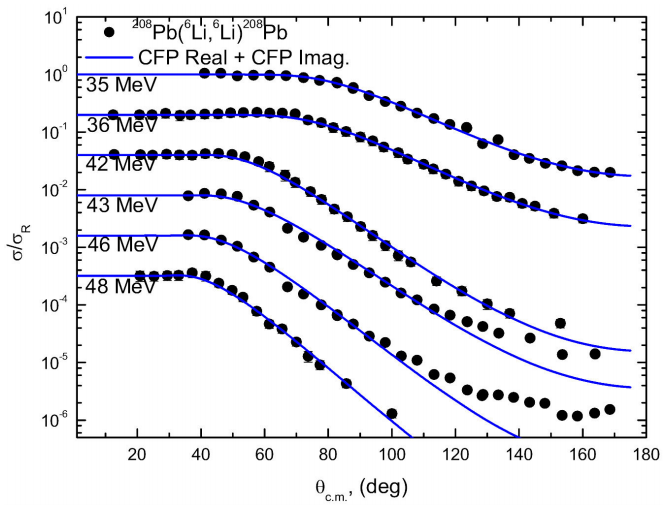
Figure 13 Same as Fig. 12 but at Elab = 35, 36, 42, 43, 46 and 48 MeV. The data are displaced by 0.2 for the sake of clarity.

Figure 14. Same as Fig. 12 but at Elab = 73.7, 88, 99, 156 and 210 MeV. The data are displaced by 0.2 for the sake of clarity.
Table IV Optimal potential parameters extracted from 6Li + 208Pb analysis using CFP for both real and imaginary parts. Real and imaginary volume integrals as well as reaction cross-sections are also listed.
E (MeV) | NR CF | NICF |
|
|
JV (MeV.fm3) | JW (MeV.fm3) |
---|---|---|---|---|---|---|
25 | 0.532 | 0.1 | 0.05 | 11.00 | 216.59 | 40.71 |
29 | 0.64 | 0.414 | 2.96 | 230.6 | 259.46 | 167.84 |
30 | 0.494 | 0.439 | 0.015 | 316.6 | 200.06 | 177.79 |
31 | 0.489 | 0.529 | 0.06 | 470.5 | 197.82 | 214.01 |
33 | 0.442 | 0.477 | 19.7 | 654.0 | 178.43 | 192.56 |
35 | 0.386 | 0.517 | 0.82 | 881.4 | 155.49 | 208.26 |
36 | 0.307 | 0.581 | 0.15 | 1006 | 123.54 | 233.79 |
42 | 0.205 | 0.844 | 0.52 | 1659 | 81.97 | 337.47 |
43 | 0.1 | 0.779 | 10.4 | 1665 | 39.94 | 311.15 |
46 | 0.1 | 0.854 | 25.6 | 1905 | 39.82 | 340.02 |
48 | 0.147 | 1.11 | 0.52 | 2178 | 58.2 5 | 439.84 |
73.7 | 0.29 | 0.768 | 0.486 | 2833 | 112.13 | 296.94 |
88 | 0.367 | 0.672 | 3.4 | 2991 | 139.78 | 255.94 |
99 | 0.238 | 0.992 | 0.49 | 3345 | 87.4 6 | 364.53 |
156 | 0.296 | 0.789 | 0.93 | 3502 | 104.99 | 279.87 |
210 | 0.464 | 1.22 | 3.19 | 3921 | 155.67 | 409.31 |
3.4 6Li + 208Pb data analysis using CFM plus a dynamical polarization potential
It is clearly shown from the current study and also previous studies concerning 6Li + 208Pb nuclear system that, the real real part of the potential constructed on microscopic procedures needs a renormalization by about 40-60 % in order to reproduce the experimental data. This reduction was assumed to be due to the break-up effect observed in the loosely bound 6Li nucleus. The analysis of 6Li + 208Pb elastic scattering data using real part of potential constructed on DF and CF procedures showed the same trend, in order to reproduce the data, NRCF should be reduced by ∼ 62 %. This well-known reduction in the real DF and CF potentials’ strength to reproduce the experimental data could be compensated by the introducing an additional dynamical polarization potential (DPP) of surface repulsive shape. This DPP simulates the polarization effects caused by the break-up of the weakly bounded projectiles. The data are then reanalyzed using the following total potential:
where the nuclear potential consists of three parts: a) real part derived from CF procedure without any renormalization (NRCF = 1), b) an imaginary CF potential with renormaliztion factor NICF fixed to the same values obtained from CFM analysis, and c) a dynamical polarization potential,
Table V Optimal potential parameters extracted from 6Li + 208Pb analysis using CFP for both real and imaginary parts with fixed NRCF =1.0 plus DPP “surface potential with a repulsive part”.
E (MeV) | NICF | Vpol | rpol | apol |
|
|
---|---|---|---|---|---|---|
25 | 0.1 | -24.42 | 1.18 | 0.99 | 0.05 | 11.00 |
29 | 0.414 | -20.88 | 1.18 | 0.98 | 3.08 | 231.0 |
30 | 0.439 | -29.72 | 1.18 | 0.98 | 0.015 | 317.2 |
31 | 0.529 | -30.1 | 1.18 | 0.98 | 0.06 | 471.1 |
33 | 0.477 | -33.24 | 1.18 | 0.98 | 20.5 | 655.9 |
35 | 0.517 | -36.67 | 1.18 | 0.98 | 0.82 | 884.1 |
36 | 0.581 | -41.75 | 1.18 | 0.98 | 0.15 | 1008 |
42 | 0.844 | -47.61 | 1.18 | 0.98 | 0.52 | 1661 |
43 | 0.779 | -59.07 | 1.18 | 0.98 | 9.6 | 1652 |
46 | 0.854 | -62.45 | 1.18 | 0.98 | 19.6 | 1882 |
48 | 1.11 | -50.41 | 1.18 | 0.98 | 0.53 | 2180 |
73.7 | 0.768 | -41.14 | 1.18 | 0.98 | 0.45 | 2844 |
88 | 0.672 | -35.38 | 1.18 | 0.98 | 4.1 | 3009 |
99 | 0.992 | -42.56 | 1.18 | 0.98 | 0.49 | 3358 |
156 | 0.789 | -38.57 | 1.18 | 0.98 | 1.74 | 3522 |
210 | 1.22 | -36.88 | 1.18 | 0.98 | 8.85 | 3920 |
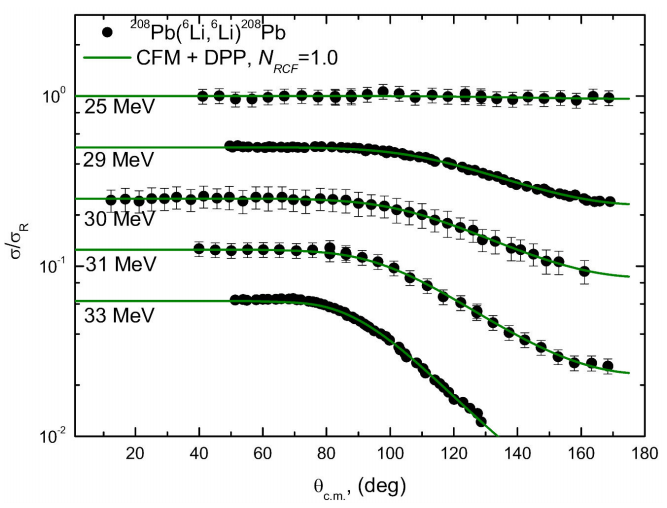
Figure 15 Comparison between 208Pb(6Li, 6Li) 208Pb elastic scattering angular distributions (solid circles) and CFP fits with non-renormalized real cluster folding potential (NRCF = 1) plus a DPP term (solid curves) at Elab = 25, 29, 30, 31, and 33 MeV. The data are displaced by 0.5 for the sake of clarity.
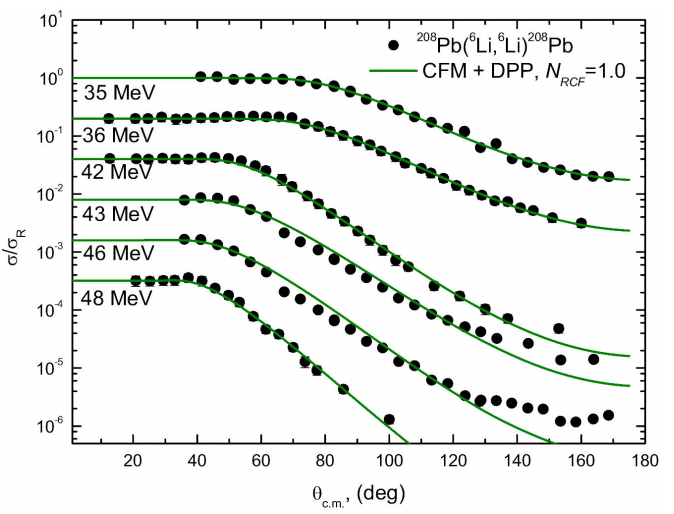
Figure 16 Same as Fig. 15 but at Elab = 35, 36, 42, 43, 46 and 48 MeV. The data are displaced by 0.2 for the sake of clarity.

Figure 17 Same as Fig. 15 but at Elab = 73.7, 88, 99, 156 and 210 MeV. The data are displaced by 0.2 for the sake of clarity.
The energy dependence on total reaction cross section
4 Summary
Experimental 6Li+ 208Pb elastic scattering angular distributions at sixteen energy sets ranging from 25 MeV and up to 210 MeV are subjected to detailed analysis using different potentials constructed based on phenomenological, semi microscopic, and microscopic models. Firstly, 4-varying parameters OM calculations were successful in reproducing the concerned data. The nuclear potential has two parts: real and an imaginary volume term each has a WS shape of fixed radius parameter (rV =1.3 fm and rW =1.7 fm).
Then, the semi microscopic calculation based on a real potential part constructed using SPP2 REGINA code was performed in order to eliminate the ambiguities inherited in the phenomenological OM. Two approaches were used: in the first approach, the real part of the potential was derived using SPP and the imaginary part was taken as a factor times the real SPP. In the second approach, the real part of the potential was derived using SPP exactly as in the first approach in addition to an imaginary volume part has a WS form to simulate the reduction in flux due to absorption. Calculations using the aforementioned two approaches showed the necessity to reduce the strength of the real part by ∼ 49 % and 52 % in order to reproduce the experimental data.
Finally, the fully microscopic CFM based on d + α cluster structure for 6Li is tested. Reasonable fitting could be obtained if the strength of the real CF potential is reduced by ∼ 62 %. The observed reduction in the real potential strength is mainly due to the 6Li break-up effect. CFM+DPP calculations are performed with non-renormalized real CF potential (NRCF = 1.0) in addition to a repulsive real surface potential which takes into account the dynamic contributions from all the allowed inelastic channels due to coupling.