PACS: 24.75.+i; 25.85.Ec; 21.10.Ft; 1.10.Dr
1. Introduction
Positive even-odd effects, in proton and neutron numbers distributions, in higher windows of kinetic energy of fragments from thermal neutron induced fission of actinides are well stablished1.
The even-odd effect of charge distribution is defined by the relation
where
However, when C. Signarbieux et al. 2 found the evidence of the existence of cold fission, corresponding to highest kinetic energy windows, for which the excitation energy is not enough for fragments to emit neutrons, they did not find a significant even-odd effect in the distribution of the mass numbers. This set a controversy in those authors that, based on the even-odd effects in proton and neutron number distributions, respectively, observed in light fragment kinetic energy, supported the hypothesis that the fission process is superfluid 2. However, in 1981, M. Montoya 3,4 deduced the relation
which is confirmed by H. Nifenecker 5. After this relation there is no contradiction between null even-odd effects in mass distribution and positive even-odd effects in proton and neutron number distributions, respectively.
In 1991 F. Gönnenwein and B. Börsig 6 show that the minimum excitation energy is lower for the odd than for the even
In this work we review experimental data to show that there is no contradiction between positive even-odd effects in total maximal kinetic energy and negative effects in minimal excitation energy of fragments.
2. Formalism for even-odd effects in cold fission
In order describe even-odd effects in cold fission is useful to recall some definitions related to them. See Ref. 6. Let be a fissile nucleus with charge Z f and mass A f that splits in a light fragment with Z L protons, N L neutrons (number of nucleons A L = Z L + N L ) and a heavy fragment with Z H protons, N H neutrons (number of nucleons A H = Z H + N H ). These numbers obey the following relations:
and
In order to simplify notations, Z L , N L , and A L will be renamed Z, N and A, respectively.
After scission, light and heavy fragments acquire kinetic energies K L , K H , and excitation energies X L , X H , respectively. Thus, the total kinetic energy (K) and the total excitation energy (X) are
and
respectively. These quantities are limited by the energy balance equation:
where Q is the available energy of the reaction.
At the scission point, the available energy is spent into deformation energy (D), Coulomb interaction energy (C) and free energy (F), according to relation
The free energy is partitioned into intrinsic energy (X *) and total pre-scission energy of fragments (K sc ):
One assumes that, for a given fragmentation corresponding to proton and mass numbers Z and A, respectively, the maximum total kinetic energy (K max) is reached by a configuration with X * = 0, maximum Coulomb interaction energy (C max), and a minimum total deformation energy (D min), limited by the equation
Because Coulomb repulsion between fragments is the unique force after scission, Coulomb interaction potential energy at scission becomes the final total kinetic energy, so that:
Let be A an odd nucleon number of the light fragment, the local even-odd effect in the maximum Q-value (
In general
Because the even-odd effect of charge and mass distribution, respectively, increases with the fragment kinetic energy 1, a positive local even-odd effect in the maximum total kinetic energy as a function of mass
and positive values of
3. Even-odd effects in the maximum total kinetic energy
In 1986 J. Trochon et al. 10 present the curve of the maximum total kinetic energy as a function of light fragment mass from the reaction 235U(n th , f). For each A they identify the charge Z that maximizes the total kinetic energy K. See Fig. 1.

Figure 1. Thermal neutron induced fission of 235U. Curve of the maximum total kinetic energy (K max) as a function of the light fragment mass number is presented. The measured odd charges that maximize K max for several mass fragmentations are indicated. The other cases correspond to neighboring even charge fragmentations. Taken from Ref. 10.
The K
max value corresponding to an odd mass are generally below the average of the values corresponding to two neighboring even masses, which means that
In general, for each A, an even charge maximizes K, except in transitions between two neighboring even charges. These cases are the following:
Applying the definition of

Figure 2. Even-odd effects on maximal total kinetic energy (K max) as a function of charge (Z) of fragments from the reaction 235U(n th , f).
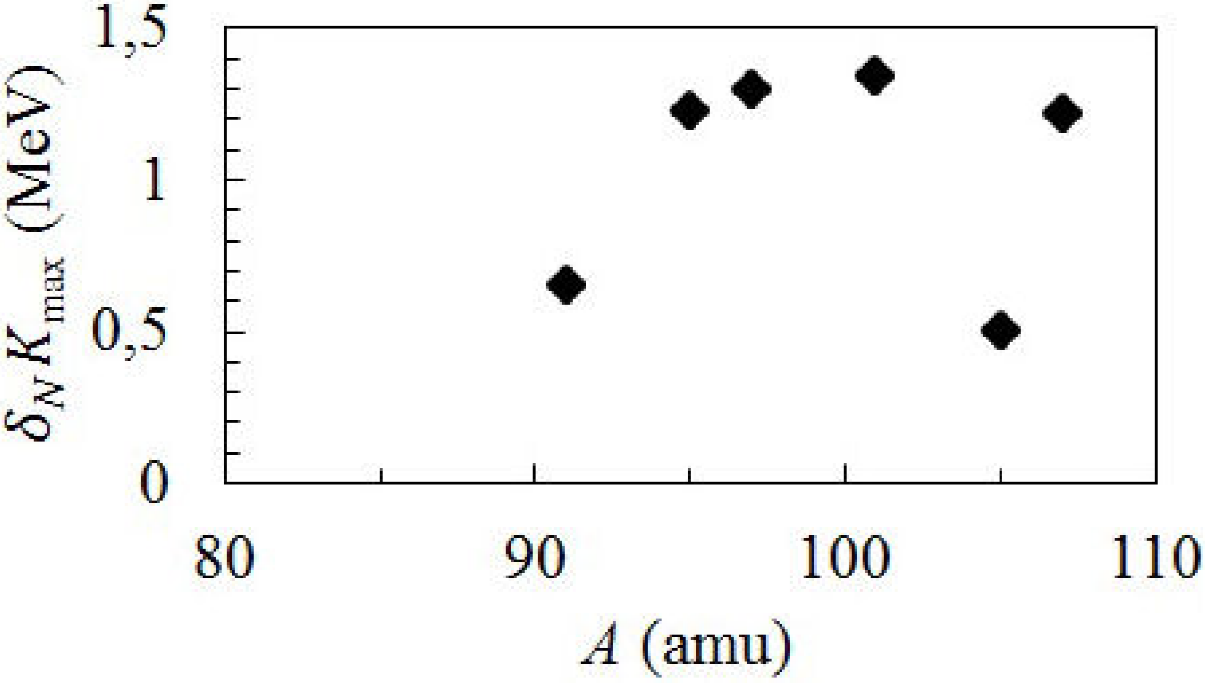
Figure 3. Even-odd effects on maximal total kinetic energy (K max) as a function of neutron number (N) of fragments from the reaction 235U(n th , f).
are approximately the same (189.3 MeV). See Fig. 4.

Figure 4. The available energy (Q) as a function of charge (Z) and mass (A) of fragments from the reaction 235U(n th , f). Atomic masses values are taken from Ref. 11.
Taking regions with even Z, one also observes positive
One must notice that
From experimental result one can observe that
and
To interpret this result one must take into account that
and
regarding which it follows that
which suggests that the even-even fragments are harder than odd A fragments, they need higher deformation energy to get the most compact configuration that obeys the relation
A positive even-odd effect in D min implies that an odd charge or neutron number splits will reach K values closer to their corresponding Q-values than the even splits do, as it was observed by F. Gönnenwein 6,8 and F.-J. Hambsch 7.
4. Discussion
In this work positive even-odd effects on maximal kinetic energy as a function of Z, N and A, respectively, of light fragments from the reaction 235U(n th , f), were put in evidence.
One must notice that, in 2013, F. Gönnenwein and B. Börsig 10 show that, for isobaric fragmentations 104/132, the kinetic energy associated to the charge fragmentation 41/51 reach the Q-value of the reaction, while the corresponding to the fragmentation 42/50 reaches a total maximum kinetic energy below 3 MeV the corresponding Q-value. These authors suggest that this is due to the fact the charge split 41/51 corresponds to odd fragment charges. However, we should note that charge fragmentation 41/51 is more asymmetric than the 42/50 fragmentation. Therefore that result is also consistent with the Coulomb effect after which for neighboring masses with similar values of energy available, the more asymmetric fragmentation reaches the higher values of total kinetic energy 12,13.
The fact that the charge split 41/51 reaches the Q-value means that both fragments are in their respective ground states (X = 0) whose corresponding scission configuration is so that
With a same configuration the charge split 42/50 with fragments in their corresponding ground states have not necessarily a similar relation. It means that at least one fragment must be deformed out of its ground state, then
and
Moreover, the isobaric mass fragmentation 104/132 corresponds to a pronounced turning point in the Q-values. See Fig. 4. The highest Q-value and the highest K
max correspond to the transitional deformed nucleus
5. Conclusion
Based on the Coulomb effect hypothesis 12,13 we have demonstrated that, in thermal neutron induced fission of 235U, there is no contradiction between the positive even-odd effects in the maximal kinetic energy (measured by J. Trochon et al. 10), and the negative even-odd effects in the minimal excitation energy of fragments (shown by F. Gönnenwein 8) as a function of fragment neutron and proton numbers, respectively. Assuming that the maximum Coulomb energy configuration corresponds to the minimum excitation energy one, the deformation energy explains both seemingly contradictory mentioned results.