1. Introduction
The metallogenic potential of the mid-Miocene to Recent Trans-Mexican Volcanic Belt (TMVB) and Miocene to Recent ore deposits in Mexico have been traditionally set aside in regional exploration endeavours and metallogenic overviews. However, advances in the last decades have begun to stress the importance of the Miocene to Recent metallogeny of Mexico, including the TMVB (Camprubí, 2009, 2013; Clark and Fitch, 2009; Poliquin, 2009; Jansen et al., 2017; Camprubí et al., 2019, 2020; Fuentes-Guzmán et al., 2020). The mineralization in the Xoconostle prospect is associated with volcanic and hypabyssal rocks of the Michoacán-Guanajuato volcanic field (part of the TMVB), which range in age between the late Miocene and Holocene (Pasquarè et al., 1988; Pérez-Orozco et al., 2018). Such ages are consistent with the youngest regional metallogenetic episodes proposed by Camprubí (2009, 2013). These deposits were earlier described as Au-Ag-Hg hot spring-style epithermal low sulfidation ore bodies that were mined at a small scale for mercury (Arredondo et al., 1989; Morales-Alvarado, 1996).
This paper aims to contribute to the characterization of the metallogeny of the TMVB, which is one of the youngest metallogenic provinces in the region. In this case, alunite from a deep hypogene advanced argillic assemblage is dated. This paper is circumscribed to a long-standing program that aims to understand the geochronological characterization of Mexican mineral deposits and the geologic events with which they are associated in time, space and genesis (Camprubí et al., 2003, 2015, 2016a, 2016b, 2017, 2018, 2019, 2020; Farfán-Panamá et al., 2015; Martínez-Reyes et al., 2015; González-Jiménez et al., 2017a, 2017b; Enríquez et al., 2018; Fuentes-Guzmán et al., 2020), to better constrain the metallogenic evolution of Mexico, as essayed by Camprubí (2009, 2013, 2017).
2. Geology
The Xoconostle epithermal deposit (Servicio Geológico Mexicano, 2002) is associated with the E-W regional-scale Morelia-Acambay normal fault zone (or Acambay graben; Martínez-Reyes and Nieto-Samaniego, 1990; Garduño-Monroy et al., 2009; Pérez-Orozco et al., 2018). The activity of this fault zone probably started at ~10 Ma as left-lateral faults (Garduño-Monroy et al., 2009) followed by extensional displacement with the hanging walls tilted northwards due to listric faults (Israde-Alcántara and Garduño-Monroy, 1999).
During the Quaternary, these faults show normal displacements and a left-lateral strike slip component characterized by a left-lateral transtensive deformation (Suter et al., 1992, 2001; Ego and Ansan, 2002). Some of the segments in the Morelia-Acambay normal fault zone show evidence for movement between the late Pleistocene and the Holocene (Langridge et al., 2000; Suter et al., 2001; Garduño-Monroy et al., 2009, in Pérez-Orozco et al., 2018). A noteworthy geological feature in the region is the Amealco caldera, northeast of the Xoconostle deposit.
In the study area, Pliocene to Holocene volcanic and subvolcanic rocks of the TMVB crop out. From older to younger, these rocks include: the El Terrero ignimbrite (andesitic-dacitic; 6.12 ± 0.60 Ma), the Siete Cruces dome complex (rhyolites; 5.45 ± 0.50 Ma), and the Amealco unit (basalts, andesites, and ash-fall deposits; ca. 3 Ma; Murillo-Muñetón and Torres-Vargas, 1987; Pasquarè et al., 1988; Aguirre-Díaz and McDowell, 2000). Such units overlie Miocene volcanic rocks geologically circumscribed to the Sierra Madre Occidental, and late Miocene basalts and andesites constituiting the earliest manifestations of the TMVB (Pasquarè et al., 1988). Pliocene ages were previously deduced for the Xoconostle deposit based on the age of the host dacitic and rhyolitic rocks of the El Terrero ignimbrite and the Siete Cruces dome complex (Camprubí, 2009, 2013). Locally, an intermediate stock was pervasively altered with advanced argillic assemblages (Morales-Alvarado, 1996), but no clear genetic relationship between this stock and the epithermal mineralization can be determined.
The epithermal ore bodies of the Xoconostle prospect (also known as San Antonio, or El Cinabrio) are located approximately at the 20°01’06’’N and 100°20’42’’W geographic coordinates, in the Epitacio Huerta municipality in the northeasternmost tip of the state of Michoacán in south-central Mexico (Figure 1).
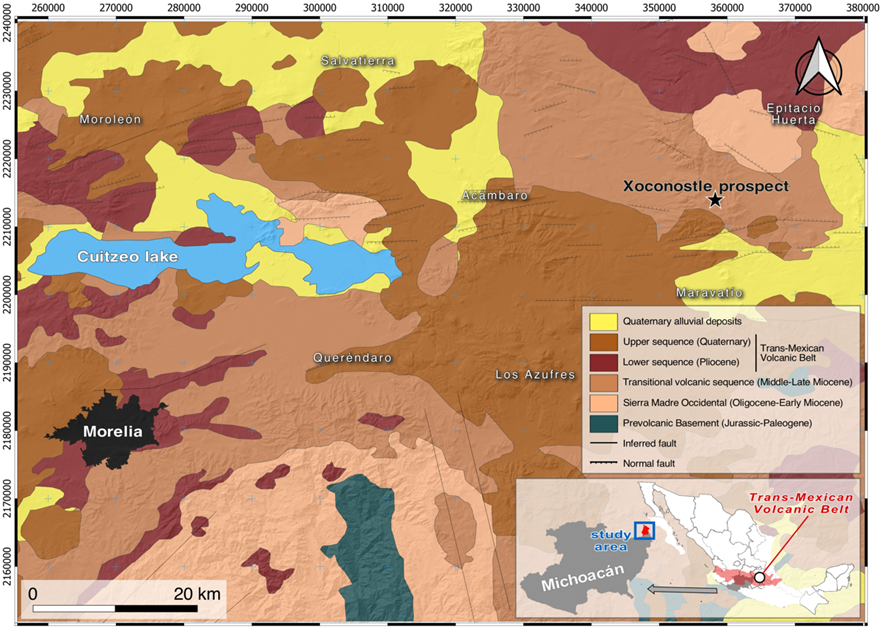
Figure 1 Regional geological map including the Xoconostle high sulfidation epithermal deposit, modified from Pasquarè et al. (1988).
These bodies consist of breccias (3 to 20 m wide, and undetermined vertical extent) predominantly cemented by massive aggregates and stockworks of silica minerals (chalcedony, opal, quartz, cristobalite and tridymite, determined by XRD), associated with three prominent alteration zones (Arredondo et al., 1989; Prol-Ledesma, 1990; Morales-Alvarado, 1996) from inner to outer: (1) silicification, comprising quartz, opal, tridymite, cristobalite and anatase, grading into (2) advanced argillic zone, comprising kaolinite, alunite, quartz, chalcedony, and pyrite, up to ~400 m wide, and into (3) argillic zone including kaolinite, cristobalite, and quartz. Propylitic alteration including chlorite, epidote, illite, and calcite occurs farther from the ore bodies in isolated patches. The ore-bearing assemblages contain pyrite, sphalerite, hematite, cinnabar, auricupride [Cu3Au] and supergene iron oxihydroxides within massive to crudely banded silica (quartz, opal and chalcedony) ore bodies (Prol-Ledesma, 1990; Morales-Alvarado, 1996). Despite its depiction in early works as a low sulfidation deposit, we consider, based on our studies, that the characteristics of the Xoconostle prospect are more compatible with a high sulfidation epithermal deposit. Exploration surveys indicate grades up to 3 ppm Au and up to 1.3 wt.% Hg in the mineralized area (Arredondo et al., 1989; Morales-Alvarado, 1996). Induced polarization (IP) surveys allow determining prospective areas where breccias and stockworks were later found (Arredondo et al., 1989). The microthermometric study of fluid inclusions in the Xoconostle deposit yielded temperatures of homogenization that range between 140° and 240 °C, and calculated salinities at ~0.1 wt.% NaCl equiv. (Prol-Ledesma, 1990).
3. Methods and results
Two pure alunite mineral separates from outcropping epithermal alteration material from the Xoconostle prospect were dated by 40Ar/39Ar geochronology (Table 1). The analyzed samples correspond to alunite crystals separated from deep hypogene advanced argillic alteration assemblages adjacent to the main massive quartz ore body in the high sulfidation epithermal deposits. These samples were massive, not pulverulent, aggregates of white to slightly rose-hued fine-grained and euhedral to subhedral platy alunite crystals. Hence we classify the advanced argillic alteration assemblage to which this alunite belongs as deep hypogene (e.g., Sillitoe, 1993, 2015).
Table 1 40Ar/39Ar determination dataset for two alunite samples from deep hypogene advanced argillic alteration assemblages in the Xoconostle high sulfidation epithermal deposit.
EH21 Alunite | |||||||||||||||
---|---|---|---|---|---|---|---|---|---|---|---|---|---|---|---|
Laser | Isotope Ratios | ||||||||||||||
Power (%) | 40>Ar/39Ar | 2σ | 36Ar/39Ar | 2σ | 39Ar/40Ar | 2σ | 36Ar/40Ar | 2σ | Rho | K/Ca | %40Ar rad | f 39Ar | 40Ar*/39ArK | Age | 2σ |
2.1 | 329.34 | 7.90 | 1.4950 | 0.0915 | 0.0030 | 0.0001 | 0.0045 | 0.0003 | 0.097 | 13.63 | 8.31 | 1.25 | 27.355 | 7.80 | ± 5.35 |
2.2 | 223.01 | 4.50 | 1.0208 | 0.0607 | 0.0045 | 0.0001 | 0.0046 | 0.0003 | 0.102 | 12.52 | 7.54 | 2.55 | 16.827 | 4.80 | ± 3.62 |
2.3 | 140.87 | 6.11 | 0.6059 | 0.0417 | 0.0071 | 0.0003 | 0.0043 | 0.0002 | 0.101 | 12.77 | 13.13 | 5.96 | 18.502 | 5.28 | ± 2.17 |
2.4 | 113.49 | 2.11 | 0.4665 | 0.0272 | 0.0088 | 0.0002 | 0.0041 | 0.0002 | 0.055 | 7.65 | 16.98 | 8.92 | 19.271 | 5.50 | ± 1.61 |
2.5 | 99.73 | 4.61 | 0.4043 | 0.0300 | 0.0100 | 0.0005 | 0.0041 | 0.0002 | 0.021 | 8.12 | 18.13 | 10.46 | 18.085 | 5.16 | ± 1.48 |
2.6 | 84.44 | 1.94 | 0.3157 | 0.0187 | 0.0118 | 0.0003 | 0.0037 | 0.0002 | 0.102 | 5.95 | 24.49 | 17.93 | 20.685 | 5.90 | ± 1.12 |
2.6 | 71.81 | 1.62 | 0.2551 | 0.0153 | 0.0139 | 0.0003 | 0.0036 | 0.0002 | 0.087 | 6.88 | 28.25 | 21.27 | 20.291 | 5.79 | ± 0.91 |
2.7 | 73.08 | 1.28 | 0.2699 | 0.0158 | 0.0137 | 0.0002 | 0.0037 | 0.0002 | 0.064 | 10.00 | 25.42 | 15.46 | 18.578 | 5.30 | ± 0.95 |
2.8 | 77.87 | 1.56 | 0.2882 | 0.0168 | 0.0128 | 0.0003 | 0.0037 | 0.0002 | 0.086 | 11.91 | 25.27 | 16.20 | 19.677 | 5.61 | ± 1.01 |
J = 0.00015610 ± 0.00000023 Volume 39ArK = 0.400
Integrated Date = 5.57 ± 0.44 Ma
Age = 5.57±0.44 Ma (2s, including J-error of .5%) MSWD = 0.27, probability = 0.98 100% of the 39Ar steps 1 through 9
Inverse isochron (correlation age) results: Model 1 Solution (±95%-conf.) on 9 points
Age = 5.4±1.0 Ma Initial 40Ar/36Ar =202.5±9.3 MSWD = 0.34 Probability = 0.53
EH21 Alunite | |||||||||||||||
---|---|---|---|---|---|---|---|---|---|---|---|---|---|---|---|
Laser | Isotope Ratios | ||||||||||||||
Power (%) | 40Ar/39Ar | 2σ | 36Ar/39Ar | 2σ | 39Ar/40Ar | 2σ | 36Ar/40Ar | 2σ | Rho | K/Ca | %40Ar rad | f 39Ar | 40Ar*/39ArK | Age | 2σ |
2.00 | 56.13 | 17.02 | 0.17 | 0.07 | 0.02 | 0.005 | 0.0031 | 0.0009 | 0.111 | 5.68 | 7.94 | 0.01 | 4.459 | 30.89 | ± 108.87 |
2.30 | 10.75 | 0.15 | 0.035 | 0.0018 | 0.09 | 0.001 | 0.0033 | 0.0002 | 0.027 | 6.34 | 2.28 | 2.94 | 0.245 | 1.71 | ± 3.70 |
2.80 | 1.35 | 0.02 | 0.003 | 0.0001 | 0.75 | 0.011 | 0.0021 | 0.0001 | 0.048 | 20.56 | 37.96 | 47.41 | 0.511 | 3.57 | ± 0.28 |
3.10 | 1.41 | 0.02 | 0.003 | 0.0001 | 0.71 | 0.013 | 0.0021 | 0.0001 | 0.139 | 24.63 | 38.31 | 31.16 | 0.541 | 3.78 | ± 0.31 |
3.40 | 2.73 | 0.06 | 0.007 | 0.0004 | 0.37 | 0.008 | 0.0026 | 0.0001 | 0.208 | 22.61 | 21.35 | 6.36 | 0.583 | 4.07 | ± 0.82 |
3.90 | 13.37 | 0.20 | 0.043 | 0.0022 | 0.07 | 0.001 | 0.0032 | 0.0002 | 0.029 | 12.94 | 4.01 | 4.18 | 0.537 | 3.75 | ± 4.51 |
4.40 | 13.93 | 0.21 | 0.046 | 0.0022 | 0.07 | 0.001 | 0.0033 | 0.0002 | 0.062 | 14.65 | 1.88 | 4.26 | 0.261 | 1.82 | ± 4.52 |
4.80 | 16.47 | 0.26 | 0.054 | 0.0027 | 0.06 | 0.001 | 0.0033 | 0.0002 | 0.063 | 11.73 | 1.28 | 3.69 | 0.211 | 1.47 | ± 5.56 |
J = 0.00381690 ± 0.00000573 Volume 39ArK = 2.515 x E-13 cm3 NPT
Integrated Date = 3.67 ± 0.20 Ma
Plateau age = 3.67 ± 0.20 Ma (2s, including J-error of .2%) MSWD = 0.65, probability=0.71 Includes 100% of the 39Ar steps 1 through 8
Inverse isochron (correlation age) results, plateau steps: Model 1 Solution (±95%-conf.) on 8 points
Age = 3.70 ± 0.26 Ma Initial 40Ar/36Ar =295.1 ± 7.4 MSWD = 0.62 Probability = 0.71
The alunite samples were crushed in a ring mill, washed in distilled water and ethanol, and sieved when dry to -40+60 mesh. Appropriate mineral grains were picked out of the bulk fraction. The samples were wrapped in aluminum foil and stacked in an irradiation capsule with similar-aged samples and neutron flux monitors (Fish Canyon Tuff sanidine [FCs], 28.201 ± 0.046 Ma; Kuiper et al., 2008). The samples were irradiated in October 2017 at the McMaster Nuclear Reactor in Hamilton, Ontario, in a shielded can for 6 MWH in the medium flux site 8E. Analyses (n = 39) of 13 neutron flux monitor positions produced errors of <0.5% in the J value. The samples were analyzed at the Noble Gas Laboratory, Pacific Centre for Isotopic and Geochemical Research (PCIGR) of The University of British Columbia in Vancouver, BC, Canada. The mineral separates were step-heated at incrementally higher powers in the defocused beam of a 10W CO2 laser (New Wave Research MIR10) until fused. The gas evolved from each step was analyzed by a VG5400 mass spectrometer equipped with an ion-counting electron multiplier. All measurements were corrected for total system blank, mass spectrometer sensitivity, mass discrimination, radioactive decay during and subsequent to irradiation, as well as interfering Ar from atmospheric contamination and the irradiation of Ca, Cl and K. The isotope production ratios were: (40Ar/39Ar)K = 0.0005 ± 0.00006, (37Ar/39Ar)Ca = 1048 ± 0.9, (36Ar/39Ar)Ca = 0.3952 ± 0.0004, Ca/K = 1.83 ± 0.01(37ArCa/39ArK).
Details of the analyses, including plateau (spectrum) and inverse isochron plots, are presented in Table 1 and Figure 2. Initial data entry and calculations were carried out using the software ArArCalc (Koppers, 2002). The plateau and correlation ages were calculated using Isoplot v.3.09 (Ludwig, 2003). Errors are quoted at the 2-sigma (95% confidence) level and are propagated from all sources except mass spectrometer sensitivity and age of the flux monitor. The best statistically justified plateau and plateau age were picked based on the following criteria: (1) three or more contiguous steps comprising more than 60% of the 39Ar, (2) probability of fit of the weighted mean age greater than 5%, (3) slope of the error-weighted line through the plateau ages equals zero at 5% confidence, (4) ages of the two outermost steps on a plateau are not significantly different from the weighted-mean plateau age (at 1.8σ six or more steps only), and (5) outermost two steps on either side of a plateau must not have nonzero slopes with the same sign (at 1.8σ nine or more steps only). The analyzed samples yielded, as best estimates, plateau ages at 5.57 ± 0.44 and 3.67 ± 0.20 Ma, that we interpret as the ages of deep hypogene hydrothermal alteration associated to the mineralization of the Xoconotle prospect (Figure 2).
4. Discussion and conclusions
As the host rocks to the Xoconostle high sulfidation epithermal deposits are the El Terrero ignimbrite (andesitic-dacitic; 6.12 ± 0.60 Ma; Pasquarè et al., 1988) and the Siete Cruces dome complex (rhyolites; 5.45 ± 0.50 Ma; Pasquarè et al., 1988), both 40Ar/39Ar alunite ages obtained in this study constitute a reasonable approximation to the age of the deposit, despite the ~2 Myr discrepancy between the 5.57 ± 0.44 Ma (Messinian, latest Miocene) and the 3.67 ± 0.20 Ma (Zanclean, early Pliocene) ages (Figure 3). In high sulfidation epithermal deposits, their genetically associated magmatic rocks and the subsequent hydrothermal activity that generated the deposits are expected to span a short period of time of about a few kyr (e.g., Arribas et al., 1995; Jansen et al., 2017). Also, the Amealco caldera, whose rim is located ~15 km to the northeast of the Xoconostle deposit, begun its activity at ~4.7 Ma with trachyandesites and trachydacites (Aguirre-Díaz and McDowell, 2000; Figure 3).

Figure 3 Summary of alunite ages in this study along with available ages for volcanism with which the Xoconostle deposit is more likely to be associated within the Trans-Mexican Volcanic Belt (TMVB).
Therefore, even the 5.57 ± 0.44 Ma age could account for the formation of the deposit considering its standard deviation. In case the undated intermediate stock partially hosting the deposit (Morales-Alvarado, 1996) were younger than the 5.45 Ma dome complex, the likeliest age for the mineralization would be Zanclean, not Messinian. Among several episodes in its evolution since its inception, the Amealco caldera produced bimodal andesite-basalt volcanism at ~3.7 Ma, during an episode that also produced rhyolitic domes in its southwest rim (Aguirre-Díaz and McDowell, 2000; Figure 3). Such age would agree with subsequent hydrothermal activity at 3.67 ± 0.20 Ma in the Xoconostle deposit (Figure 3). Despite the geological likelihood of both ages in this study, to the present reckoning it would be premature to favoring one above the other (or not, thus implying hydrothermal activity for over 2 Myr), and further research would be instrumental for such decision.
The Morelia-Acambay normal fault zone (or Acambay graben) ranges in age between 4.7 and 2.2 Ma (Martínez-Reyes and Nieto-Samaniego, 1990; Aguirre-Díaz and McDowell, 2000; Aguirre-Díaz et al., 2000; Garduño-Monroy et al., 2009; Pérez-Orozco et al., 2018), and the formation of the Xoconostle epithermal deposit is circumscribed to its characteristically E-W structural features (Servicio Geológico Mexicano, 2002). In that case, a Zanclean age for this deposit would fit better the known age constraints for regional geological features. The obtained ages in this study also compare well with the third stage of evolution of the Trans-Mexican Volcanic Belt (TMVB) of Gómez-Tuena et al. (2005, 2007), which is characterized by acid and bimodal volcanism, and predate the Late Pliocene-Present arc in the TMVB (Figure 3).
Setting aside the above considerations, the Xoconostle deposit is firmly circumscribed to the youngest manifestations of magmatism in the TMVB, and adds up to other magmatic-hydrothermal deposits in this geological province (Camprubí, 2009, 2013; Poliquin, 2009; Camprubí et al., 2020; Fuentes-Guzmán et al., 2020), confirming its significant metallogenic potential. Besides Xoconostle, at least another high sulfidation epithermal deposit occurs in the TMVB: the Caballo Blanco deposit in Veracruz, with possible late Miocene to Pliocene age (Cuttle and Giroux, 2017). A relatively small number of low sulfidation epithermal deposits in the eastern half of the TMVB has also been dated late Miocene to Pliocene in age (Poliquin, 2009; Camprubí et al., 2020). Among them, it is worth noting the Ixtacamaxtitlán deposit in Puebla. As mentioned by Camprubí et al. (2020) and Fuentes-Guzmán et al. (2020), the TMVB contains one of the youngest metallogenic provinces in Mexico. Beyond the possibility of finding early Miocene magmatic-hydrothermal deposits in the TMVB (Camprubí, 2013; Fuentes-Guzmán et al., 2020), this study, along with those of Poliquin (2009) and Camprubí et al. (2020) emphasizes the occurrence of late Miocene to Pliocene deposits in the region. Each group of deposits would be associated with the first and third stages in the magmatic evolution of the TMVB, according to Gómez-Tuena et al. (2005, 2007), which enhance its metallogenic potential. Also, recent magmatism originated several geothermal fields associated with the fourth stage of the TMVB, like the Pathé-Yexthó in Hidalgo and Querétaro, Los Azufres in Michoacán, or Acoculco in Puebla and Hidalgo (Camprubí, 2013, and references therein; Sosa-Ceballos et al., 2018).