Introduction
The evolutionary processes that maintain and generate variation are important, particularly so for endemic species, since an adequate level of heterozygosity may improve the adaptive potential of populations in case of environmental change (Jamieson 2007); thus, genetic diversity is positively related to the reduction of extinction risk (Frankham et al. 2002). The amount of allelic or haplotypic variability is influenced by several factors, such as gene flow among demes (connectivity), natural selection, and population size (Bolnick et al. 2011); consequently, a clear understanding of these processes is crucial for conservation efforts.
There is a wide range of studies on the use of genetic information to guide conservation efforts (Lancaster et al. 2009), many of which focus on the relation between allelic variability and the condition of endemic species, in many cases residing in marine protected areas or under exploitation. The Gulf of California is one of the areas in which this approach has been more successful. For example, it has been suggested that the survival of the only vaquita population (Phocoena sinus Norris and McFarland, 1958) depends more on human activities than on its genetic diversity, which is extremely low (Munguia-Vega et al. 2007). In commercial species, such as the leopard grouper, Mycteroperca rosacea (Streets, 1877), genetic information has been used to design no-take zones in the upper Gulf of California, which hosts high genetic diversity and could replenish nearby fishing grounds due to larval dispersal (Jackson et al. 2015). Furthermore, genetic data and oceanographic modeling have been used to highlight the importance of established marine protected areas in sustaining nearby fisheries while preserving populations of species such as the brown sea cucumber, Isostichopus fuscus (Ludwig, 1875); the leopard grouper, M. rosacea; and the snappers Hoplopagrus guentheri Gill, 1862 and Lutjanus argentiventris (Peters, 1869) (Munguia-Vega et al. 2014, Soria et al. 2014).
The coral genus Porites Link, 1807 is one of the most important reef builders in the world, containing more than 150 extant species distributed in tropical and subtropical regions (Hoeksema 2015). There are 9 Porites species in the Pacific coast of Mexico (Reyes-Bonilla 2002, Glynn et al. 2017a), including the endemic scleractinian coral of the Gulf of California: Porites sverdrupi Durham, 1947 (Chiriboga et al. 2008). The distribution of this species ranges from Ángel de la Guarda Island (29ºN) to Marías Islands (20ºN), at depths from 1 to 40 m (Fig. 1, Chiriboga et al. 2008). In the 1990s the species started disappearing in the south (Reyes- Bonilla 1993), and in the following decade the extinction of all populations south of 24ºN was confirmed (Chiriboga et al. 2008, Reyes-Bonilla and López-Pérez 2009) and most likely due to increasing seawater temperature (Paz-García and Balart 2015). Currently there are only 3 areas where healthy populations can be found: the San Lorenzo Channel in La Paz Bay (24ºN), Santa Catalina Island in Loreto Bay (25ºN), and El Requesón Beach in Concepción Bay (26ºN) (Fig. 1). Among eastern Pacific Porites, P. sverdrupi is the only species with a branched morphology (López-Pérez et al. 2003), and it is usually associated with rhodolith beds in the form of free-living colonies (Reyes-Bonilla et al. 1997).
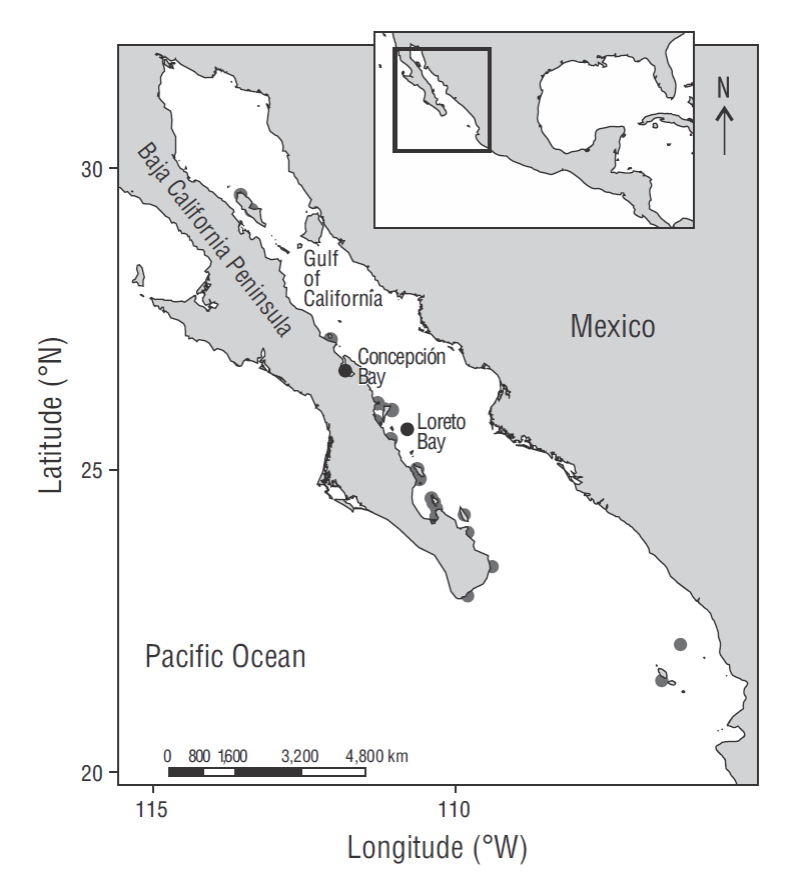
Figure 1 Distribution of Porites sverdrupi. Gray shaded areas represent the recorded historical distribution. Study sites are marked with black circles.
According to the Red List of the International Union for Conservation of Nature, P. sverdrupi is listed as vulnerable due to its limited distribution range and its high susceptibility to disease, bleaching, and habitat degradation (Chiriboga et al. 2008). In spite of the species’ vulnerability to extinction, research has mostly focused on its taxonomy and biogeography, and much information on its biology, ecology, and genetics is therefore still missing; this lack of data complicates the establishment of efficient conservation strategies. In a first attempt to fill this information gap, we have assessed the genetic diversity and gene flow between 2 of the most important extant populations of P. sverdrupi.
Materials and methods
Coral samples were collected in Concepción and Loreto bays. Corals from La Paz Bay could not be sampled because a cyclonic event prevented our team from finding them (Fortunately, we were able to find colonies in 2016, which means this population is still extant.). At each sampling site, 50 coral fragments were collected at 10 and 20 m depth by hand using scuba gear. Divers took care to sample from different colonies that were separated by at least 2 m to avoid collecting genetically identical ramets. Samples were immediately fixed in 96% alcohol and taken to the laboratory. There, lithium chloride extraction of DNA from each sample was performed, followed by amplification of the internal transcribed spacer (ITS) region (F-primer ITS4 TCCTCCGCTTATTGATATGC, R-primer ITS5 GGAAGTAAAAGTCGTAACAAGG; White et al. 1990). The resulting polymerase chain reaction (PCR) amplicons of the ITS region were digested with restriction enzymes MboI, RsaI, DpnII, and TaqI to perform a restriction fragment length polymorphism (RFLP) analysis. Restriction sites were coded for presence or absence to form composite haplotypes, from which nucleotide and haplotype diversities were computed (Nei and Tajima 1981) using REAP 4.0 software (McElroy et al. 1992). An analysis of molecular variance (AMOVA) using a matrix of interhaplotypic distances (Nei and Tajima 1981) was performed to estimate genetic structure in Arlequin (Excoffier et al. 1992). Significance was computed by permutation tests (10,000 iterations).
Results
DNA extractions were successful for all samples (N = 100). However, ITS amplifications were successful only in 62 fragments: 33 from Concepción Bay and 29 from Loreto Bay. A total of 10 composite haplotypes were observed in both study regions; 3 of them were exclusive to Concepción Bay, while the rest were present at both sites. There were 3 dominant haplotypes (H4, H8, and H9): H4 was dominant in Loreto Bay (in 41% of specimens); H9 was the most common in Concepción Bay (in 39% of specimens); and H8 was present at both sites (Table 1).
Table 1 Haplotype frequency of Porites sverdrupi colonies at Concepción (CO) and Loreto (LO) bays.
Haplotype number | Composite haplotype |
CO (N) | % | LO (N) | % | Total (N) | % |
H1 | AAAA | 1 | 3.03 | 1 | 3.45 | 2 | 3.23 |
H2 | AAAB | 1 | 3.03 | 4 | 13.79 | 5 | 8.06 |
H3 | AABA | 1 | 3.03 | 1 | 3.45 | 2 | 3.23 |
H4 | AABB | 5 | 15.15 | 12 | 41.38 | 17 | 27.42 |
H5* | AABC | 1 | 3.03 | 0 | 0 | 1 | 1.61 |
H6* | ABAA | 1 | 3.03 | 0 | 0 | 1 | 1.61 |
H7 | ABAB | 2 | 6.06 | 1 | 3.45 | 3 | 4.84 |
H8 | ABBA | 6 | 18.18 | 9 | 31.03 | 15 | 24.19 |
H9 | ABBB | 13 | 39.39 | 1 | 3.45 | 14 | 22.58 |
H10* | ABBC | 2 | 6.06 | 0 | 0 | 2 | 3.23 |
Total | 33 | 100 | 29 | 100 | 62 | 100 |
* Private haplotypes.
Dominant haplotypes in bold.
Concepción Bay had the highest haplotype diversity (h = 0.79), whereas nucleotide diversity was highest in Loreto Bay (π = 0.20). Nucleotide diversity was several orders of magnitude higher among populations (π = 0.22) than within populations (π = 0.001, Table 2). Finally, AMOVA results showed significant genetic structure between geographic populations (Φst = 0.067, P = 0.029, d.f. = 61; Table 3). This result was supported by a midpoint rooting neighbor-joining phylogram (Fig. 2), which divided haplotypes into 2 clades, each containing one of the haplotypes with the highest frequencies (H4 and H9, representative of Loreto Bay and Concepcion Bay, respectively).
Table 2 Haplotype and nucleotide diversities in Porites sverdrupi.
Concepción Bay | Loreto Bay | |
Coral colonies (N) | 33 | 29 |
Haplotypes (N) | 10 | 7 |
Haplotype diversity (h) | 0.7888 ± 0.0369 | 0.7211 ± 0.0372 |
Nucleotide diversity (π) | 0.0199 | 0.0206 |
Nucleotide diversity (among populations) |
0.0217 | |
Divergence (among populations) |
0.0015 |
Table 3 Molecular analysis of variance for Porites sverdrupi populations (significance level based on 10,000 permutations).
Source of variation | d.f. | Sum of squares | Variance component | Percentage of variation |
Among populations | 1 | 0.033 | 0.0007 | 6.71 |
Within populations | 60 | 0.607 | 0.0101 | 93.29 |
Total | 61 | 0.640 | 0.0109 | |
Fixation index (ΦST): 0.0671 (P = 0.0290) |
Discussion
Although the taxonomic status of P. sverdrupi has been discussed thoroughly (López-Pérez et al. 2003), this is the first study addressing the species’ genetic variation. Previous phylogenetic research on Porites from the eastern, central, and western Pacific, and from the Caribbean, has shown that P. sverdrupi is closely related to Porites panamensis, forming reciprocally monophyletic groups (Forsman et al. 2009). Unlike P. sverdrupi, P. panamensis is a scleractinian coral that is widely distributed along the eastern tropical Pacific and forms encrusting and massive colonies over rocky bottoms (Veron 2000).
Genetic diversity is expected to be low in endemic species with small population sizes and restricted geographical distribution or in cases where each population is isolated from others (Hobbs et al. 2013). While all of these conditions apply to P. sverdrupi, the high levels of genetic diversity in both studied populations were unexpected (h > 0.7, Table 2). Similar diversity values were observed in an analysis of P. panamensis populations from Loreto Bay, La Paz, and Cabo Pulmo (23.5ºN) using 2 nuclear DNA loci and 5 alloenzymatic loci as markers (Saavedra-Sotelo et al. 2013). The authors point out that populations in the Gulf of California were the most diverse in the Pacific coast of Mexico, with h > 0.6. The described patterns suggest that high haplotype diversity in nuclear ribosomal loci may be characteristic of the genus along the western coast of the Americas.
Since the examined populations of P. sverdrupi were separated by over 100 km, some level of structure was expected to be found, as the same pattern of genetic structure between relatively close populations has also been found in mollusks, marine mammals, and fish in other Pacific regions (Wood and Gardner 2007, Lancaster et al. 2009, Hobbs et al. 2013). This was indeed the case, as shown by the level of genetic distinction between local populations and the neighbor-joining phylogram (Table 3, Fig. 2). For marine species, genetic connectivity can be affected by various parameters, such as physical barriers (topography), the predominant direction and speed of marine currents, the occasional influence of anomalous movement patterns caused by events such as El Niño/Southern Oscillation, and the oceanographic conditions (nutrients, food, water transparency) favoring the transit and colonization of a coral propagule (Wood et al. 2016). Concepción Bay receives water from the central Gulf of California, and its elongated geomorphology produces a cyclonic flow in the central area (Martínez-López and Gárate-Lizárraga 1997). This produces a retention zone, making it difficult for gametes and larvae to leave the bay and recruit elsewhere. These circumstances change during fall with an outflow from the bay because of changes in wind direction (Martínez-López and Gárate-Lizárraga 1997). This limited potential for dispersal may contribute to the observed genetic structure.
Previous research involving eastern Pacific corals has shown that some species, such as Pocillopora damicornis and Pavona gigantea, do not exhibit genetic structure in the Gulf of California (Chávez-Romo et al. 2008, Saavedra-Sotelo et al. 2011), whereas other species, like P. panamensis, have shown significant structure inside and outside the gulf (Saavedra-Sotelo et al. 2013). Biogeographic and systematic patterns in sexual reproduction have been detected in corals, and lecithotrophic larvae produced from brooding colonies are known to have a lower dispersal potential than planktotrophic larvae produced by broadcast spawning colonies (Harrison 2011). The different reproductive traits in coral species inhabiting the same region can explain why some species such as P. sverdrupi and P. panamensis experience significant population genetic structuring while others such as P. damicornis and P. gigantea do not.
Studies on P. panamensis suggest that its reproductive biology is a proximal cause of the genetic differences found among populations (Paz-García et al. 2012, Saavedra-Sotelo et al. 2013). This is a gonochoric species with large short-lived planulae (>300 µm), which remain in the water column for less than 2 weeks and usually settle near the parental colony (Glynn et al. 2017b). All of these features contribute to limit long-distance dispersal and therefore favor the existence of genetic differences among local populations. The pelagic larval duration of P. sverdrupi is unknown, but the species is phylogenetically close to P. panamensis, is also gonochoric, and produces large planulae (over 300 µm) for a short period of time (about 3 months, unpublished data). Taking all of this into consideration together with our results, particularly the presence of haplotypes specific to Concepción Bay, we suggest that P. sverdrupi larvae may also have a short free-living period, which would be consistent with the limited genetic exchange between populations in the central Gulf of California. The finding of 7 shared haplotypes between Loreto Bay and Concepción Bay supports some level of gene flow between populations but insufficient to homogenize the gene pool and produce a single panmictic population. According to particle dispersal models of the Gulf of California (Marinone et al. 2016), at least 10% of particles released from Concepción Bay between August and December will arrive to the central-southern region of the Gulf of California one month later. This, along with the predominant outflow from Concepción Bay during the fall (Martínez-López and Gárate-Lizárraga 1997), supports our hypothesis that genetic exchange probably exists but at low levels.
Geographic isolation is not the only force that contributes to genetic structure among populations. Even in the face of gene flow, selective pressures are able to create differentiation due to local adaptation (Kawecki and Ebert 2004). This scenario can explain the structure found in P. sverdrupi, for which the most relevant factor would be the particular oceanographic conditions of Concepción Bay. First, the bay has a temperature range of 18 to 35 ºC, about 10 ºC wider than the one for Loreto Bay (26 to 36 ºC, Mateo-Cid et al. 1993), and shallower depths. Second, the physiography of Concepción Bay makes for a long residence time of water (about 34 days, Mendoza-Salgado et al. 2006). Third, the high local primary productivity can generate suboxic and even anoxic episodes in the central part of the bay. In addition, these conditions can promote the liberation of hydrogen sulfide and generate a toxic environment for several days (Rodríguez-Meza et al. 2009). Fourth, the west coast of the bay has hydrothermal vents with continuous emissions of water with very high temperature (normally over 30 ºC, but up to 87 ºC) enriched with metals such as arsenic, boron, barium, cesium, iron, mercury, iodine, lithium, silicon, and strontium (Oporto-Guerrero et al. 2016). This environmental setting, to which the colonies of P. sverdrupi must adapt, may act as a selective barrier for the survival of individuals from other areas that may present very different physiological traits. Migrants from elsewhere would be able to arrive and settle inside Concepción Bay, but their survival, recruitment, and successful reproduction may be compromised. In short, the remarkably distinct environments prevailing in the 2 study areas could also be contributing to the observed genetic distinction of populations evolving independently.
The coral P. sverdrupi is an endemic, vulnerable species, and one of the purposes of this research was to link our results with conservation efforts. Although the original geographic range of P. sverdrupi spanned over 500 km (Fig. 1), by the 1990s the species started disappearing in the south (Reyes-Bonilla 1993). In the following decade, the extinction of all populations south of 24ºN was confirmed (Chiriboga et al. 2008, Reyes-Bonilla and López-Pérez 2009). Our results do not evidence low genetic diversity in the species (Table 2) and suggest that gene flow, although limited, must exist between populations. These are not typical conditions of a species undergoing extinction or one that has suffered a severe contraction of its effective population size, where low demographic sizes generally lead to a loss of genetic variability due to drift and to the high probability of inbreeding involving further depression of genetic variability. This discrepancy may mean that P. sverdrupi has a high rate of effective sexual reproduction and a small proportion of clones. Further research on this subject must be done to confirm our hypothesis.
Due to the genetic differentiation between the studied populations, and the high risk of extinction of this species in the Gulf of California, these populations should be considered important for conservation purposes. Strategies to improve their condition, such as restoration, must be developed. Also, we strongly recommend the incorporation of this coral species into the NOM-059-SEMARNAT-2010 (the official Mexican regulation for endangered species) because it fits the conditions for inclusion due to its low genetic connectivity, limited range of distribution, small population sizes, and high susceptibility to disease. Along with official regulations and restoration, research on the biology and ecology of this endemic coral is essential in any attempt to preserve the species. Fortunately, some of these studies are currently being carried out and new biological data for this species are being obtained in the field.
In conclusion, the 2 studied populations of P. sverdrupi exhibited high genetic diversity. A total of 10 haplotypes were found in 62 analyzed colonies, all of which appeared at Concepción Bay (3 of them are unique to the area) and 7 were shared between this area and Loreto Bay. We suggest that the genetic structure between populations is probably caused by a combination of short pelagic larval duration, potentially low connectivity among populations, and local adaptation. Although the species populations have been suffering a steady decline in recent decades, genetic data do not show signs of genetic erosion, and further investigation is needed to fully understand the population dynamics and processes of this species.