Introduction
Pepper is a vegetable grown year-round in almost all of Mexico, and is one of the main fruit and vegetable products consumed. An estimated 80 % of pepper production is for the domestic market, which determines its importance as food. In addition to possessing minerals and vitamins, it is a condiment that is included in most Mexican dishes. The most widely cultivated species of pepper is Capsicum annuum L. (jalapeño, serrano, pasilla, guajillo, poblano, red pepper; Aguilar-Rincón, 2012).
Leaf area index (LAI) is a key variable to study crop development and growth. In addition, it is the basis for estimating water, nutritional and bioenergy efficiency requirements, and to determine potential phytosanitary damage. There is a close relationship between LAI and the interception of solar radiation, associated with photosynthesis and transpirative processes, aspects strongly linked to biomass accumulation and productivity. Therefore, LAI is a useful variable for quantifying the growth and agronomic yield of crops (Elings, 2000; Hernández-Hernández et al., 2011).
Direct LAI determination is generally destructive and requires instruments to integrate the leaf area (White, Asner, Nemani, Privette, & Running, 2000). As a variant of this method, Ovando (1999) and Rodríguez (2000) used digital photographs and image interpretation techniques to measure leaf area and determine LAI in potato. On the other hand, there are indirect non-destructive methods that allow rapid measurement in the field; the operating principle of these methods is the close relationship between radiation penetration and canopy structure.
The electromagnetic spectrum region of greatest agricultural interest is photosynthetically active radiation (PAR), whose wavelength is between 400 and 700 nm. Because plants perform photosynthesis and PAR is their energy source, knowing the spatial and temporal distribution of crop PAR interception is essential for the analysis of associated biological processes (Grossi-Gallegos, 2004).
De la Casa et al. (2008) used a ceptometer in potato and obtained promising results when comparing the LAI estimates with measurements obtained from digital photographs. Steven, Biscoe, Jaggard, and Paruntu (1986) showed that the ratio between the fraction of the intercepted photosynthetically active solar radiation (fIPAR) and the cover percentage (f) in several crops is sufficiently close to 1:1, which makes it possible to estimate fIPAR from cover, which is an easier measurement to obtain. Other researchers estimated the LAI in wheat and rice using Beer's law (Monsi & Saeki, 1953).
There is limited information on the development and growth of poblano pepper for the purposes of irrigation and nutrient scheduling. One of the variables of agronomic management associated with its productivity is the number of stems. The greater the number of stems, the greater the leaf area; however, production, in terms of quantity and quality, may be affected. Therefore, it is necessary to know the development behavior of the crop under different management conditions for the purposes of irrigation and nutrition scheduling.
The aim of this study was to estimate the leaf area index of poblano pepper (Capsicum annuum L.) with a ceptometer, compare the results with the destructive method to determine if the former is a reliable method, and analyze the relationship that exists between the LAI and crop yield.
Materials and methods
Description of the experiment
The experiment was carried out in a greenhouse at the Colegio de Postgraduates, Montecillo campus, State of Mexico (19.96 ° North latitude and 98.90 ° West longitude, at 2,244 masl). The greenhouse used was a triple tunnel with metal structures, covered with polyethylene plastic with 75 % transmissivity and anti-insect mesh on the side walls. In addition, it had a passive ventilation system through side vents and manually-opened skylights.
The cultivation of indeterminate-growth poblano pepper variety Capulín F1 was studied. It was planted in trays on February 21, transplanted on April 21 and the harvest ended on November 11, 2014. The planting framework was staggered, with a separation of 35 cm between plants and 45 cm between lines. The seedlings were transplanted in 35 x 35 cm plastic bags containing red tezontle.
The treatments (T) consisted of three management conditions based on the number of stems per plant: T1 (two stems), T2 (three stems) and T3 (without pruning). In T1 and T2, the main stem and the secondary ones were allowed to grow. T3 was allowed to grow uncontrolled in number of stems.
The area of each treatment was 53 m2, together covering a total area of 159 m2. Each main treatment was established in plots with two beds of 20 m in length, spaced 1.35 m apart. A planting density of 3 plants·m-2 was used. According to the usual modality in the region, the average density used ranges from 3 to 5 plants·m-2. Treatments were distributed in split plots (10 m2 each) in randomized blocks with four replicates.
A drip irrigation system was used, with a surface irrigation line of 16 mm in diameter. Each line had separate self-compensating drippers at 40 cm and a flow of 4 L·h-1 per dripper, with an operating pressure of 0.7 kg·cm-2. Irrigation was applied throughout the growing season. In the first 30 days after transplanting (dat), five irrigations were applied per day (10:00, 12:00, 13:00, 14:00 and 15:00 h) with duration of 3 min. They were then increased to eight irrigations (9:00, 10:00, 11:00, 12:00, 13:00, 14:00, 15:00 and 16:00 h) with duration of 4 min.
Description of the method of estimating leaf area index with a ceptometer
LAI measurement was performed with an AccuPAR LP-80 ceptometer (RFA/IAF Ceptometer, Decagon Devices, Pullman, Washington, USA), which estimates PAR under field conditions using a 1-m-long bar with 80 sensors divided into eight segments. Readings were taken every 15 days from 50 dat (date on which stem number division and treatments began) to the last fruit harvest (November 11, 2014). Six replicates were performed per treatment, above and below the canopy. The intercepted PAR values were estimated from two positional radiation levels, one above the foliage to obtain the incident PAR (PARa) and the other under the foliage to obtain PAR reaching the ground (PARd) in µmol·m-2·s-1 (Equation 1).
Once PAR was obtained, it was combined with other variables, such as the leaf angle distribution factor, beam zenith angle and the extinction coefficient for radiation, and an adjustment was made to estimate the LAI of the plant according to Equation 2 proposed by Campbell and Norman (1989).
Where K is the extinction coefficient for radiation, which when considering a spherical angular distribution parameter x = 1, fb is the fraction of direct radiation with respect to global solar radiation (0.25) and A is a general absorption coefficient of the canopy that is equal to 0.86 (Campbell & Norman, 1989).
Measurements with the ceptometer were performed only under clear sky conditions and during the period of maximum insolation (the hours close to noon). In this way, the zenith angle was the lowest possible and the fb always corresponded to high fractions of direct solar radiation, which allowed homogenizing the data.
To compare the LAI data obtained with the ceptometer, leaf area measurements were made with the destructive method. This method consisted of extracting the plant from the pot, then separating the leaves and measuring the leaf area with an electronic area meter (Area Meter Model LI-3100, Decagon Device, Inc). They were performed in four replicates of each treatment. Finally, the LAI of the plant was calculated with Equation 3, described by (Reis, de Azevedo, Albuquerque, & Junior, 2013).
Where the LAI is in m2·m-2, LA is the average leaf area of three plants (m2), NP is the number of plants per m2 and TA is the total area considered (1 m2).
Description of variables
For dry weight, stem, root, leaf and fruit parts were separated, weighed fresh, then placed in a drying oven at 70 °C for 72 h for dehydration and finally weighed again.
Temperature (°C) and relative humidity (%) were measured with a sensor located on a data logger (Data Logger Hobo U12-011) that was installed inside the greenhouse in the central part, 2 m from the ground.
The development of many organisms is mainly controlled by the temperature of the environment. Growing degree days (GDD) are an indirect measure of the growth and development of plants and insects. They represent the integration of the ambient temperature between two threshold temperatures, which define the interval at which an organism is active. Outside this range, the organism does not show appreciable development or can die.
The GDD concept resulted from observations indicating: 1) there is a base temperature below which plants do not grow (Neild & Smith, 1997), 2) the rate of growth increases with temperature above this base, 3) poblano pepper varieties require different values of accumulated days (AD) of the degree days (DD). The accumulated values for n days elapsed are expressed by Equation 4.
Where i is the number of days elapsed from an initial day of interest, usually the date of planting or the start date for a crop phonological stage.
To estimate daily GDD with this method requires the average environmental temperature (Equation 5; Ojeda-Bustamante, Sifuentes-Ibarra, Slack, & Carrillo, 2004).
Where Ta is the daily air temperature, and Tc-max and Tc-min are the minimum and maximum ambient temperatures, which are the thresholds at which poblano pepper grows (29 to 10 ºC; Roose, 1994).
On the other hand, to estimate yield and number of fruits, eight plants were selected per treatment.
Results and discussion
Photosynthetically active radiation
The PAR intercepted above the canopy is relatively constant throughout the cycle, compared to radiation penetrating below the canopy. It is observed that at 1,167 accumulated degree days (ADD), which corresponds to 37 dat, the amount of radiation reaching the lower leaves begins to decrease, indicating an increase in the leaf area of the plant. As the days go by, the degree of PAR interception increases. Most of the radiation that arrives above the foliage is used by the plant to perform all processes related to photosynthesis (Figure 1). This behavior is similar in the three treatments, with respect to the radiation that arrives below the canopy, and begins to vary from 1,000 ADD due to the increase in leaf area.
In T3 it was found that at the end of the cycle the radiation reaching below the canopy is scarce. This is because no foliage control was performed, resulting in a greater degree of PAR interception. These data are consistent with the results obtained by Leguizamón and Verdelli (2011) in corn and soybean grown under field conditions.
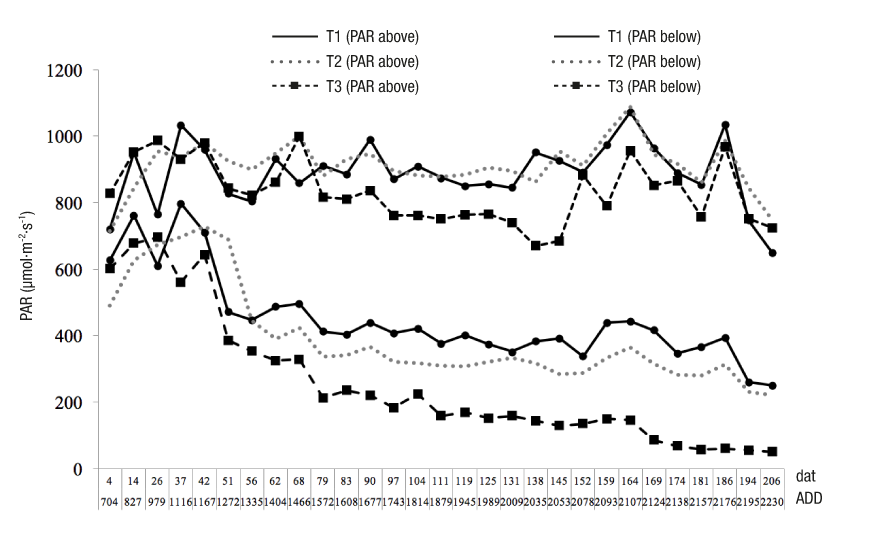
Figure 1 Photosynthetically active radiation (PAR) intercepted above and below the canopy of the plant in all three treatments during the crop cycle.
Figure 2 shows the behavior of incident PAR above the canopy every 10 min inside the greenhouse for four days from 8:00 a.m. to 8 p.m. It can be seen that the maximum radiation occurred from 1:00 to 2:00 p.m. On day four, there was a variation in radiation due to the recording of a cloudy day. Ayala-Tafoya et al. (2015) obtained similar results in red pepper cultivated under different types of shade-net colors. In addition, Samaniego-Cruz et al. (2002) reported similar PAR results with different types of polyethylene for the production of tomato and red pepper seedlings.
Leaf area index
The LAI of the poblano pepper crop was highest in T3, due to the greater number of stems per plant. This leads to the increase in LAI to a certain point, after which it begins to decrease.
From 1,246 ADD, which corresponds to 48 dat, LAI begins to differ among treatments, as the difference in the number of stems among treatments begins to be noticeable. Figures 3, 4 and 5 show the IAF obtained with the ceptometer and the destructive method. The maximum LAI occurred at 2,099 ADD with 0.93, 1.26 and 2.78 for T1, T2 and T3, respectively. Once the maximum growth and development of the plant is reached, the LAI begins to decrease due to senescence and the fall of the lower dry leaves. Escalante-Estrada (1999) obtained similar results in sunflower cultivated under residual moisture conditions. Aguilar-García, Escalante-Estrada, Rodríguez-González, and Fucikovsky-Zak (2002) and Vega-Muñoz, Escalante-Estrada, Sánchez-García, Ramírez-Ayala, and Cuenca-Adame (2001) also reported similar results in sunflower cv. Victoria cultivated under highland rainfed conditions. This methodology, in addition to confirming the results of Steven et al. (1986), allowed obtaining LAI estimates with the ceptometer.

Figure 3 Comparison of measurements of leaf area index (LAI) obtained with destructive method and its estimation using a ceptometer for treatment 1 (two stems).
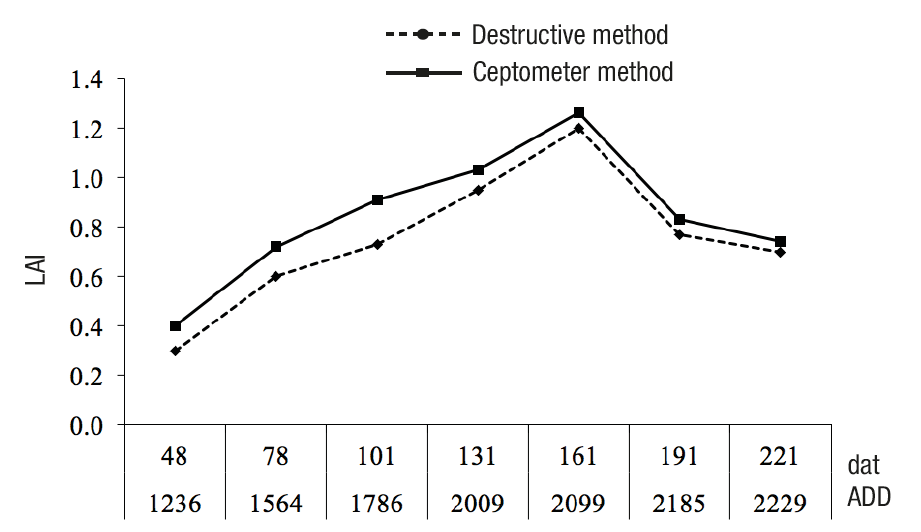
Figure 4 Comparison of measurements of leaf area index (LAI) obtained with destructive method and its estimation using a ceptometer for treatment 2 (two stems).
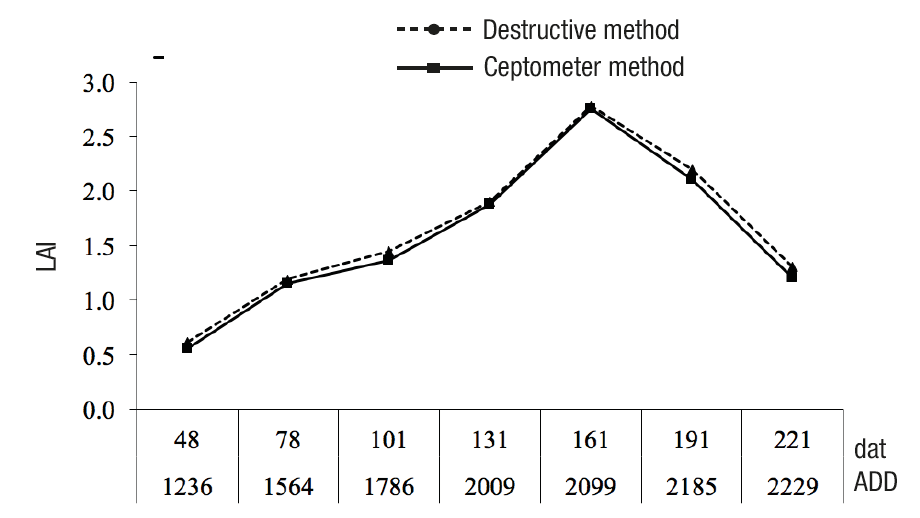
Figure 5 Comparison of measurements of leaf area index (LAI) obtained with destructive method and its estimation using a ceptometer for treatment 3 (without pruning).
Table 1 presents the phenological stages of greenhouse-grown poblano pepper variety Capulín F1, as a function of GDD.
Table 1 Phenological stages of poblano pepper in terms of growing degree days (GDD).
Stages | Duration of stage (days) | Cumulative period per stage (days) | GDD |
---|---|---|---|
Planting | 0 | 0 | 0 |
Transplant | 1 | 59 | 665 |
Vegetative development | 38 | 97 | 1116 |
Flowering and fruit development | 48 | 145 | 1394 |
Production and harvest | 107 | 252 | 1638 |
End of production | 12 | 264 | 2230 |
The correlation coefficient (R2) between the two methods was calculated. The representation shows the linear character, with the coefficient of the regression line close to unity. This coefficient, with both methods, was 0.83, 0.94 and 0.99, for T1, T2 and T3, respectively (Figure 6). In the results, point dispersion in the line is observed, and this is because the destructive determination of LAI, compared to the effects of measuring with a ceptometer, comes from a single sector (1 m2); this is in contrast to more repeated measurements that are obtained with the ceptometer. It should also be noted that the dispersion tends to increase as the sample size becomes larger, which may be a consequence of the loss of ceptometer precision under conditions of light saturation. De la Casa, Ovando, Bressanini, Rodríguez, and Martínez (2007) obtained similar results in potato, with a correlation coefficient between the two methods of R2 = 0.80.
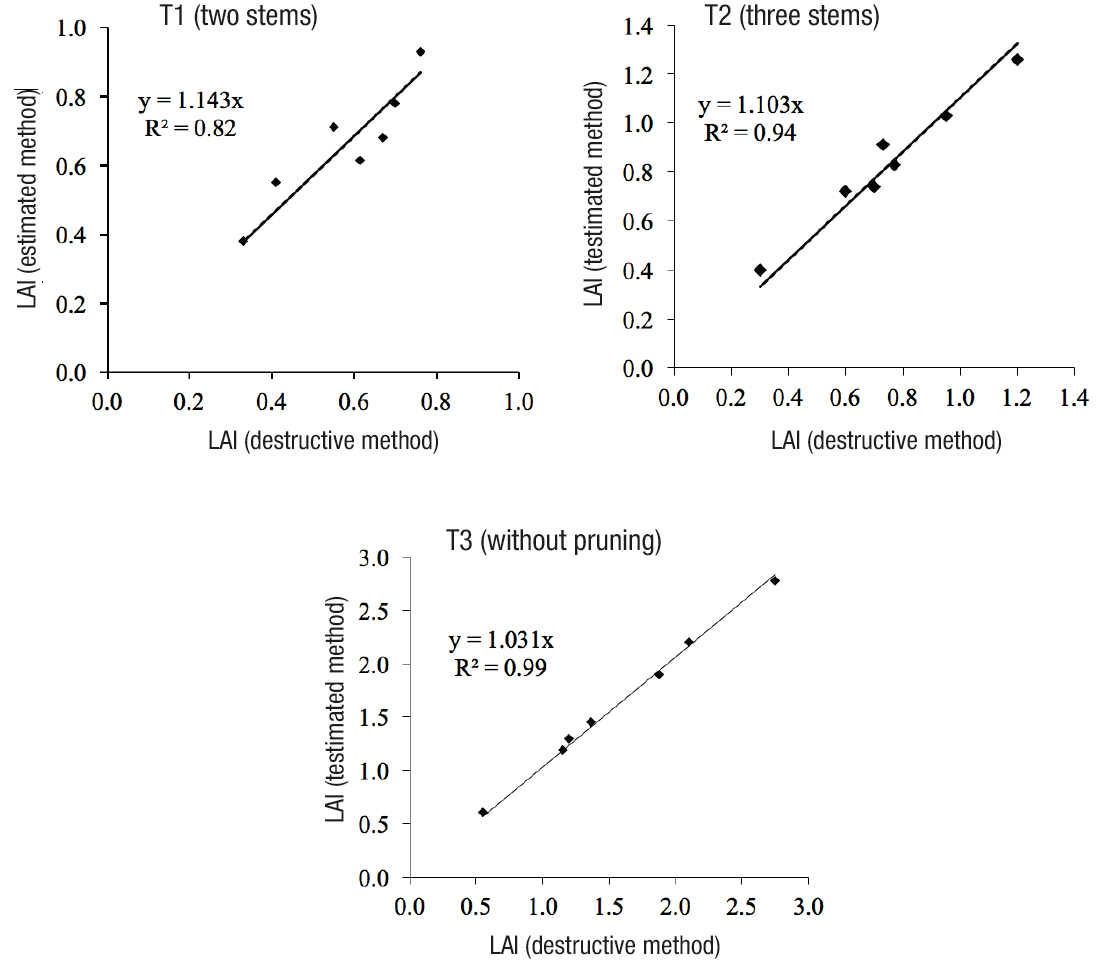
Figure 6 Relationship between measurements of the leaf area index (LAI) obtained with destructive method and its estimation using a ceptometer for the three treatments.
It was also found that T3 had a better correlation adjustment; this was due to the fact that when the foliage is larger it allows less radiation to pass, reducing the light saturation and thus the ceptometer readings are more similar to those obtained with the destructive method.
Figure 7 shows the behavior of the temperature inside the greenhouse from the time of planting to the end of cultivation. At first, the temperature was above 20 °C and remained so until 1,325 ADD. Subsequently, it began to descend (< 18 °C), coinciding with the rainy months (June to August) and cold season (< 15 °C, September to November).
Relationship between leaf area index and yield of poblano pepper
Figures 8, 9, 10 and 11 show that from 1,564 ADD, which corresponds to 78 dat, the accumulated dry biomass begins to increase in the four organs of the plant. At 2,099 ADD there is an increase in fruit production in all three treatments, which corresponds to the stage in which the fruit harvest begins. The behavior is similar in all three treatments, varying only in the degree of accumulation. This accumulation trend is similar to the results obtained by Chamú-Baranda, López-Ordaz, Ramírez-Ayala, Trejo-López, and Martínez-Villegas (2011) in red pepper in partial drying of the root under protected conditions.
Managing stem numbers is one of the most recommended agricultural practices to achieve an increase in productivity and greater interception of solar radiation in crops. This is because with an appropriate number of individuals per unit area, a better use of light, water and nutritional resources is achieved.
By increasing the number of stems per plant, a positive productive response was observed, as it increased the number of fruits per plant. This is because the foliage intercepts more photosynthetically active radiation, thereby increasing its capacity to perform photosynthetic activity, which increased fruit production.
Figure 12 shows the yield obtained in each treatment. The highest value was obtained with T3 (10.75 kg·m-2), followed by T2 (8.29 kg·m-2) and T1 (5.49 kg·m-2). T3 had the highest number of fruits with 264, followed by T2 with 129 and T1 with 102 fruits per m2. The results of this work are similar to those reported by Hernández-Palomo et al. (2016) in poblano pepper variety Capulín F1 planted under protected conditions. According to means comparison tests (data not shown), a significant difference was found among treatments in yield and number of plants.
It was also observed that while T3 had the best yield and number of fruits per plant, the disadvantage of this treatment was the uncontrolled foliage management; in addition, it was very susceptible to pests and diseases because of the poor air circulation in the foliage.
Conclusions
Estimating the leaf area index of greenhouse-grown poblano pepper using a ceptometer proved to be a suitable and reliable method. In addition, this index was positively related to yield, as increasing the number of stems per plant increased the amount of photosynthetically active radiation intercepted in the foliage; therefore, the plant increases its photosynthetic capacity and thus its production.
In addition, increasing the number of stems per plant increased leaf area index, yield and number of fruits; however, fruit size decreased.