Introduction
As habitat loss and fragmentation became a prominent focus of conservation biology in recent decades, so did the responses of organisms to habitat edges (“edge effects”; Murcia 1995; Lidicker 1999; Lidicker and Peterson 1999; Ries et al. 2004). Forests can be fragmented, which creates more edges in a landscape, by timber harvesting, conversion of land to agriculture, urban development, and a variety of other human activities (Fahrig 2003; Haddad et al. 2015). In fact, Haddad et al. (2015) estimated that 20 % of the world’s remaining forest was within 100 m of a forest edge, and 70 % was within 1 km of an edge. Pfeifer et al. (2017) examined the global landscape-level abundance of 1,304 forest-dwelling vertebrate species in relation to the amount of forest edge in the landscape. They found that 85 % of the species examined showed edge effects; 39 % showed a negative relationship and 46 % showed a positive relationship with the amount of forest edge. For mammals, the abundance of 57 % of the species examined (n = 519) showed strong declines near forest edges (Pfeifer et al. 2017).
Small mammals show a variety of responses to habitat edges (Lidicker and Peterson 1999). One response is an ecotonal effect, where a species typically associated with one habitat type (i. e., forest or matrix in this case) can occupy peripheral areas of an adjacent habitat type, creating greater species diversity in edges, novel assemblages, and perhaps even edge specialists (Lidicker 1999; Lidicker and Peterson 1999). An alternative response is a matrix effect, where a species’ distribution does not change, and the species remains in its respective habitat (e. g., remains in either forest or matrix; Lidicker 1999). In this case, assemblages along edges are similar to those in the habitat interior, whether forest or matrix, if all species show matrix effects. A strong matrix effect might indicate species with poor dispersal through the matrix, and thus high sensitivity to isolation and reduction of habitat area caused by fragmentation. Differences in population density or vital rates between edge and interior habitats could occur under either ecotonal or matrix effects, and these differences are what are usually of concern to ecologists and conservation biologists (e. g.,Morris 1989; Morris and Davidson 2000; Klein and Cameron 2012; Hannebaum 2017). Species that show a strong avoidance of edges could be especially susceptible to risk of extirpation because as forest becomes fragmented, the edge-to-interior ratio increases, and the amount of core area decreases.
For small mammals, the type of edge effect observed varies by species, and even within species it can vary by habitat type, area of the habitat patch, and season. Mills (1995) reported that Myodes (formerly Clethrionomys) californicus (red-backed voles) showed a negative effect of forest edges on abundance, possibly because the drier and warmer microclimate in these edges were less conducive to the hypogeous fungal sporocarps that the voles feed upon. However, in a later study at the same sites, Tallmon and Mills (2004) could not duplicate the previous findings and suggested that capture probabilities for voles vary over space and time; thus, replication of studies is needed to confirm the generality of findings from a single snapshot. Microtus pennsylvanicus (meadow voles) showed avoidance of forest edges in old field habitats, likely due to the possibility that the trees could provide perches for avian predators (Manson et al. 1999; Nickel et al. 2003). Pardini (2004) found one species with a positive association and three species with negative associations with forest edges out of 20 species of rodents and marsupials detected in Atlantic Forest fragments in Brazil. In contrast, Di Napoli and Caceres (2012) did not find evidence of an edge effect in abundance among 12 species examined in woodland savannah remnants in Brazil. Mazzamuto et al. (2018) reported that Myodes glareolus (bank voles) were more abundance along forest edges in Northern Italy, but that Apodemus sylvaticus (wood mouse, a species morphologically and ecologically similar to North American Peromyscus, see below) showed no relationship between abundance and edges. Forest edges in their study also were neutral in terms of species richness, survival, and personality of individual small mammals, although foraging by both species yielded lower giving-up densities (GUDs) in seed trays near edges, suggesting lower perceived predation risk.
In the midwestern United States and southern Canada, studies of the responses of Peromyscus leucopus (white-footed mice) to forest edges have yielded a variety of results (Table 1). In general, the density of P. leucopus has been found to be higher in small fragments than large fragments or extensive forest (Nupp and Swihart 1996, 1998; Krohne and Hoch 1999; Anderson and Meikle 2006; Wilder and Meikle 2006). Similarly, positive (greater abundance) edge effects have been reported several times, but always in small woodlots (i. e., <2 ha; Cummings and Vessey 1994; Anderson et al. 2003; Wilder and Meikle 2006). Peromyscus leucopus is known to select areas with more complex vertical structure of the vegetation and greater cover by fallen logs, stumps, and other downed woody debris (Kaufman et al. 1983; Barnum et al. 1992; Moore and Swihart 2005), and this has been suggested as one reason density might be higher in small fragments and edges (Anderson and Meikle 2006). Habitat quality for P. leucopus has been suggested to be both higher in forest interior (Morris and Davidson 2000; Wolf and Batzli 2002, 2004; Moore and Swihart 2005) and higher along forest edge (Wilder et al. 2005; Klein and Cameron 2012; Hannebaum et al. 2017). Additionally, P. leucopus is a primary reservoir of Lyme disease (Borrelia burgdorferi; Lane et al. 1991) and research suggests a positive relationship between black-legged tick (Ixodes scapularis) infection with Lyme disease and fragmented landscapes (Allan et al. 2003). Peromyscus leucopus also is a reservoir of hantavirus in the United States, which can result in fatal pulmonary failure (e. g.,Hjelle et al. 1995; Monroe et al. 1999). Thus, the relationships between white-footed mice and habitat fragmentation/edges may have important implications for human health.
Studies of edge effects on the other common forest-dwelling species in our study area (southern short-tailed shrew, Blarina carolinensis; eastern chipmunk, Tamias striatus) are few. Constantine et al. (2005) reported no edge effect for B. carolinensis (or any of the five rodent species included in their study) in loblolly pine (Pinus taeda) forest in South Carolina. Manson et al. (1999) found no edge effect on B. brevicauda (northern long-tailed shrew), but their study was in an old field rather than forest. King et al. (1998) reported a weak trend for more observations of chipmunks near a forest-clearcut edge in New Hampshire, but the relationship was only marginally significant (P = 0.0876; King et al. 1998:153). Heske (1995) found no difference in captures for all three species (P. leucopus, B. carolinensis, T. striatus) when comparing randomly located transects in forest interior versus forest-agricultural field (corn, Zea mays; soybeans, Glycine max) edges in southern Illinois. Thus, it has been difficult to come up with generalities given such conflicting or sparse results.
Our goal was to evaluate the generality of the findings of Heske (1995) for southern Illinois. We opted to do this for two reasons: 1) most studies cited above and in Table 1, especially those detecting edge effects in abundance, were conducted in highly fragmented landscapes whereas the study by Heske (1995) was conducted in a moderately fragmented landscape with extensive forest areas remaining, which may yield different results; and 2) given the lack of duplication of results reported by Tallmon and Mills (2004), we wanted to determine if the finding of neutral effects of forest edge reported for these forests was repeatable. In Heske (1995), transects in forest interior and forest-agricultural edge were not associated, and distributed throughout the eastern Shawnee National Forest. Here, we compare numbers of small mammals in forest edges to those in parallel transects in forest interior, which we consider a more rigorous experimental design, and a more natural matrix consisting of adjacent old field or successional field. Both studies were conducted during the late spring - summer months. If the findings of Heske (1995) are general, we expect no differences between forest edge and interior, although differences between forest and matrix may occur.
We had three main goals for this study. 1) We evaluated ecotonal effects by comparing the identities of small mammal species in forest edges, forest interior, and matrix. If ecotonal effects are manifest, assemblages along edges should prove different from those in interior or matrix. 2) We compared abundances of forest-dwelling small mammal species between forest edges and interior. Edge avoidance or attraction by species could indicate species at greater risk from habitat fragmentation, or species that may cause unanticipated ecological problems. 3) Finally, because many studies in Table 1 report differences between edge and interior in aspects of the behavior or reproductive success of females, we conducted separate analyses on numbers of reproductively active, adult female P. leucopus. During the reproductive season, females should show the strongest responses to differences in habitat quality, whereas males respond to the distribution and density of females (e. g.,Ostfeld 1985). Thus, a difference between forest edge and interior in the abundance of adult females should indicate which habitat provides greater fitness (e. g.,Morris and Davidson 2000).
Table 1 Studies of edge effects on P. leucopus in the central U.S. and southern Canada. Positive indicates density or some vital rate (e. g., reproduction) greater in forest edges than forest interior, negative indicates the reverse. No response indicates no significant differences detected between edge and interior. Multiple references in a row indicate related studies (e. g., authors reporting different aspects of a study in different papers).
Reference(s) | Positive | Negative | No response |
---|---|---|---|
Morris 1989; Morris and Davidson 2000 | Density, litter size, longevity, % reproductive adults not different, but recruitment greater in interior | ||
Cummings and Vessey 1994 | Density higher in edge of small woodlot (2 ha), reproduction greater in forest edge | ||
Heske 1995 | Captures in forest interior and agricultural edges similar | ||
Nupp and Swihart 1996, 1998 | No difference in density between forest edge and interior in either small or large patches | ||
Wolf and Batzli 2002, 2004 | Higher density in interior, no differences in litter size or survival | ||
Anderson et al. 2003 | Higher density in small (<10 ha) vs large (>100 ha) patches, edge effect in small patches only | ||
Moore and Swihart 2005 | Density lower in edges, but positively correlated with downed woody debris | ||
Wilder et al. 2005; Wilder and Meikle 2005, 2006 | Density not different between edge and interior in spring and summer, but higher density and reproductive effort in edges of small patches (<2 ha) only in autumn | ||
Klein and Cameron 2012 | Movement distances not different between edge and interior for males, but greater in interior for females, suggesting lower quality habitat | ||
Transitions from forest interior to edge locations were greater than transitions from edge to interior. Assumed transitions would be to higher-quality habitat (but see text). | |||
Hannebaum et al. 2017 |
Materials and Methods
We conducted this study from late May through late July 2003 at the Cache River State Natural Area (CRNA; 37° 21’ 44’ N, 88° 57’ 20” W), Johnson County, in southern Illinois, United States. CRNA comprises 5,793 ha of upland and bottomland forest and Tupelo-cypress swamps. Our survey transects were all located in upland forest or adjacent matrix; dominant canopy trees were maples (Acer spp.), oaks (Quercus spp.), and hickories (Carya spp.). Our study was conducted in extensive forest, avoiding confounding the influences of patch area and edge (Fletcher et al. 2007; Banks-Leite et al. 2010).
We live trapped small mammals on 500-m transects, each with 50 trap stations spaced 10 m apart. At each station, we set two folding aluminum Sherman live traps (10 x 11.25 x 37.5 cm; H. B. Sherman Co., Tallahassee, Florida USA) approximately 2 m apart, baited with sunflower seeds, for a total of 100 traps per transect. Each transect was trapped once per month (May, June, July), with traps set on day 1, checked and re-set on the morning of day 2, and checked and removed on the morning of day 3. Traps were placed to maximize capture of terrestrial small mammals (e. g., along fallen logs, at the base of large trees, in runways). We identified all small mammals captured to species, weighed them to the nearest g with a Pesola spring balance (Pesola, Schwyz, Switzerland), determined sex, assigned reproductive status (testes descended or abdominal for males; visibly pregnant, lactating, enlarged nipples indicating previous reproduction, or non-reproductive for females), and categorized them as adult, subadult, or juvenile based on size and pelage. We marked each captured animal with a small fur clip to identify recaptures. We did not identify individuals, but animals were marked with a distinctive fur clip (right haunch, left haunch, or top of rump) for each of the three surveys on a transect. Blarina carolinensis were an exception to these procedures, as their fur was too short to rely on fur clips for identification, and it was difficult to determine sex or age in the field. All captured individuals were released at their capture site. Animal capture and handling procedures conformed to guidelines approved by the American Society of Mammalogists (Sikes et al. 2016) and were approved by the University of Illinois IACUC.
We conducted our surveys along 18 transects. Fifteen transects were arrayed in five groups of three parallel transects each: one in forest edge (< 5 m from edge, under the canopy), one in forest interior (approximately 100 m from the canopy edge), and one in the adjacent field (distance from the canopy edge was constrained by size of the fields, but was always >25 m and usually 50 to 100 m; Figure 1). Four of the adjacent fields (matrix) were in a mid-successional state (dense saplings up to 2 m tall, shrubs, vines, but also with some grassy or weedy patches) and one was an old field (predominantly grass and forbs) that had been released from cattle grazing about 10 years prior to our study. Groups of transects were at least 500 m apart to assure independence. The remaining three transects included a second, nearby old field with a similar history of livestock grazing to obtain a replicate sample of old field small mammals, and two transects deeper in the forest interior (> 1 km from the nearest edge) for samples of small mammals distant from edges. We live trapped six transects simultaneously, taking approximately two weeks for a complete series of surveys (the last two weeks of each month), and always included all three transects in a group in each survey.

Figure 1 Photos of the four habitat types included in our study at Cache River State Natural Area, Illinois USA, in May - July 2003. A: Forest interior (at least 100 m from the edge); B: Old field; C: Successional field; D: Forest edge (within 5 m of the tree canopy edge).
We used principal component analysis (PCA) to compare the species composition of small mammal assemblages on each type of transect (forest edge, forest interior, successional field, old field) using data from all 18 transects. If forest edges show an ecotonal effect resulting in higher diversity or unique assemblages, we expect edge transects to map distinctly from other habitat types on a biplot of PCA axes. Similarly, PCA should reveal if the two deep-forest interior transects differ in species composition from our transects 100 m from forest edge.
We evaluated edge effects in abundance by comparing the number of individuals of each species captured on each transect, using only the 15 grouped transects and considering three categories: forest edge, forest interior, and “field” combining both successional and old field transects. As each transect was surveyed three times, we used a repeated measures analysis of variance (rmANOVA). Initially, we included month as a factor along with habitat; month was highly significant for all species examined, with fewer captures in May than in June or July, but there were no significant interactions (month*habitat, all P > 0.10) so we only report the results for habitat below. Separate analyses were conducted for each species for which there was sufficient data; only P. leucopus provided sufficient data for rmANOVA of adult females. When rmANOVA indicated significant differences among transect types, we used Tukey’s HSD to identify pairwise differences. PCA was conducted in Stata ver. 9.2 (StataCorp LLC, College Station, Texas, USA). All other analyses were conducted using R ver.2.10.1 (R Development Core Team 2009).
Results
We captured 11 species of small mammals during our surveys: P. leucopus (n = 782 individuals), B. carolinensis (n = 613 captures), Microtus ochrogaster (prairie voles; n = 105 individuals), T. striatus (n = 40), Ochrotomys nuttalli (golden mice, n = 34), Synaptomys cooperi (southern bog lemmings, n = 34), Microtus pinetorum (woodland voles, n = 33), Peromyscus maniculatus (deer mice, n = 20), Glaucomys volans (southern flying squirrels, n = 13), Zapus hudsonius (meadow jumping mice, n = 9), and Oryzomys palustris (marsh rice rats, n = 3). PCA yielded 2 axes explaining 37 % and 27 % of the total variation, respectively. Old fields were distinctly separated from the other habitat types along PCA1, and were most strongly associated with M. ochrogaster, S. cooperi, P. maniculatus, and O. palustris (Figure 2). Successional fields were distinctly separated from other habitat types along PCA2, and were most strongly associated with O. nuttalli, Z. hudsonius, and M. pinetorum (Figure 2). The deep forest interior transects, forest interior transects, and edge transects clustered together, and were most strongly associated with P. leucopus, T. striatus, and G. volans. Blarina carolinensis were associated with both forest and successional habitats (Figure 2).

Figure 2 Biplot of the first two axes uncovered by principal components analysis (PCA) of the species composition of small mammals on each transect. Note that edge, interior (100 m from edge), and deep interior (>1 km from edge, same symbol as interior) all cluster together. Species: Ts = Tamias striatus, Pl = Peromyscus leucopus, Gv = Glaucomys volans, Bc = Blarina carolinensis, Mp = Microtus pinetorum, On = Ochrotomys nuttalli, Zh = Zapus hudsonicus, Mp = Microtus ochrogaster, Pm = Peromyscus maniculatus, Sc = Synaptomys cooperi, Op = Oryzomys palustris.
Peromyscus leucopus differed in abundance among transect types (F 2,12 = 46.62, P = 0.0001). White-footed mice were less abundant on field transects (Tukey tests: edge versus field, P = 0.003; interior versus field, P < 0.0001) but did not differ in relative abundance between transects on forest edge and interior (Tukey test: edge versus interior, P = 0.59; Figure 3A). Tamias striatus also differed in abundance among transect types. Chipmunks were never captured on transects in fields, but did not differ in relative abundance between transects on forest edge and interior (F 1,8 = 0.06, P = 0.82; Figure 3B). Blarina carolinensis did not differ in the number of captures among transect types (F 2,12 = 0.90, P = 0.43; Figure 3C). There were too few captures of G. volans for analysis, and all other species were only captured in the open habitats (old field, successional field) with the exception of a single M. pinetorum on a forest interior transect.
The number of adult female P. leucopus captured on forest interior transects was greater than that on forest edge transects (F 1,8 = 5.34, P = 0.05; Figure 4). Captures of adult females of other forest-dwelling species were too sparse for analysis of edge effects.
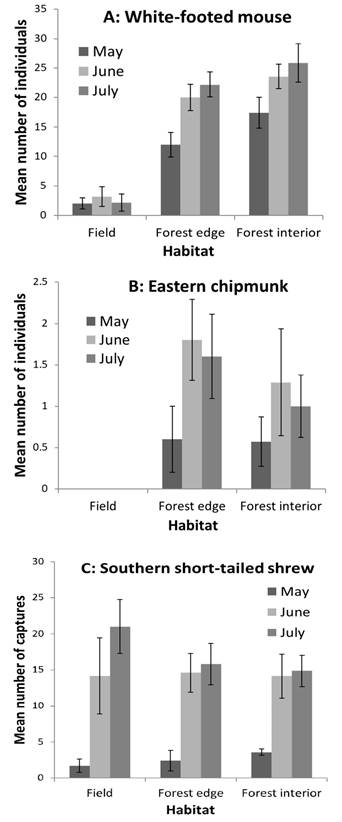
Figure 3 Mean (± SE) number of captures (individuals for P. leucopus and T. striatus, total captures for B. carolinensis, see text) for the three most abundant species of forest-dwelling small mammals on our transects at CRNA in May, June, and July 2003. A: White-footed mice, P. leucopus. B: Eastern chipmunks, T. striatus. C: Southern short-tailed shrews, B. carolinensis.
Discussion
Species richness and diversity were not greater along forest edge than in forest interior in spite of differences in the species composition of assemblages in forest and matrix (successional field, old field; Figure 2). Forest edge and interior transects (including the two deep-interior transects) were identical in terms of species composition, but differed strongly from both old field and successional field assemblages (Figure 2). Thus, most species captured in the open habitats showed a matrix effect (sensuLidicker 1999), with the forest taking the role of “matrix” in this case. One species, B. carolinensis, occurred in both forest and matrix similarly; slightly greater numbers of captures in successional fields and the old field were not significantly different from those in forest edge or interior (Figure 3C). We did not have transects along edges outside of the forest canopy, so we cannot evaluate an ecotonal effect there; however, some P. leucopus were captured in successional fields where there was woody cover (Figure 3A; see also Manson et al. 1999), so this species likely showed an ecotonal effect wherein the edge was not a hard barrier but density in the matrix was lower. Tamias striatus appeared to show a strong matrix effect as no captures of chipmunks were made in the matrix (Figure 3B), but we do not know if chipmunks occupying the forest edge might venture out into adjacent matrix for short distances.
These differences are reflected in the distribution of these species in highly fragmented Midwestern forests: P. leucopus and B. brevicauda (closely related to B. carolinensis) were ubiquitous in forest remnants and woodlots, whereas T. striatus were less so (Rosenblatt et al. 1999; Nupp and Swihart 2000), and survival of chipmunks was lower in small woodlots (Nupp and Swihart 1998). Although P. leucopus is associated with wooded or at least brushy habitats (Lackey et al. 1985), it can be found in a variety of Midwestern habitats (e. g.,Cummings and Vessey 1994) and is likely very good at dispersing even through highly fragmented landscapes. Similarly, B. carolinensis can be found in a variety of habitats (Genoways and Choate 1998; McCoy 2001), and Constantine et al. (2004) reported no effects of pine forest edges on this species. In contrast, T. striatus is much more strictly associated with closed-canopy woodlands and forests, including forest edges, although it may often occur in residential areas as well (Snyder 1982; Bowers 1995).
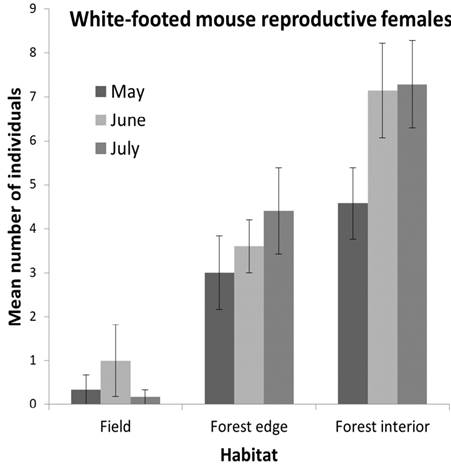
Figure 4 Mean (± SE) number of captures (individuals) of reproductive, adult female P. leucopus on forest edge and interior transects at CRNA in May, June, and July 2003.
We did not detect differences in abundance between forest edge and interior for the three most common species of forest-dwelling small mammals at our study area. Studies comparing relative abundance or density of P. leucopus have reported data showing greater abundance in some forest edges (Cummings and Vessey 1994; Anderson et al. 2003; Wilder et al. 2005; Anderson and Meikle 2006), greater abundance in forest interior (Wolf and Batzli 2002), and no difference (Heske 1995; Nupp and Swihart 1996, 1998). Anderson and Meikle (2006) found density of P. leucopus to be positively related to structural complexity of the understory, with structural complexity greater in small forest patches. Our study area was a large, extensive forest. We did not measure cover, but woody cover such as shrubs and bushes did not seem greater along the edges we surveyed; most increased ground cover was due to greater amounts of poison ivy (Toxicodendron radicans) within about 5 m of the edge (E. Heske, personal observation). We also did not observe obvious differences in downed woody debris, stumps, or logs; selective logging decades earlier had left many such items scattered throughout the forest (see Figure 1A), and traps could readily be set at the bases of large trees, stumps, or next to features providing cover or travel routes (e. g.,Barnum et al. 1992). Four of the five edges would be called “soft” given the growth of saplings, vines, briars, and weeds in the successional fields. The forest-old field edge was more “hard,” with grassy habitat transitioning abruptly to an open understory with little structural complexity. The type of edge did not affect the patterns detected, however.
Density can be a misleading indicator of habitat quality (e. g.,Van Horne 1983). In most small mammals, the distribution of reproductive females is more likely to reflect habitat quality than that of other demographic categories. As with density, studies of the distribution of reproductive, adult P. leucopus, or of reproductive parameters such as litter size, number of litters, and recruitment, have produced varied results. Morris (1989) and Morris and Davidson (2000) did not find differences between forest edge and interior in litter size, proportion breeding, or adult longevity, but did note that the success of recruiting at least one offspring per litter and the number of recruits per litter was greater in forest interior, leading them to conclude that fitness of adult females was greater in forest interior than on forest edge. Wolf and Batzli (2004) did not find any edge effects on reproductive variables, and also noted that the distribution of seeds and arthropods was not related to distance from edge. They instead found higher predation risk and higher rates of parasitism (e. g., from botflies, Cuterebra fontinella; Wolf and Batzli 2001) near edges and also concluded that forest interior was the higher-quality habitat. In contrast, Wilder and Meikle (2005) reported a greater number of litters and proportion of females producing litters in edges versus interior, and in small versus large woodlots.
Taking a behavioral approach, Klein and Cameron (2012) found that MSD (mean squared distance from the center of activity, a measure of movements related to home range size) increased for females, but not for males, along a gradient from forest edge to interior. They suggested that greater MSD reflected lower-quality habitat, such as fewer nesting sites or diet items, inducing greater movement. Hannebaum et al. (2017) took another novel behavioral approach to the question of habitat quality: transitions of individuals from one habitat type to another (e. g., from interior transects to edge transects). Their analyses showed greater numbers of males than females making transitions, body mass negatively related to the probability of transition, and more transitions from interior to edge than vice versa, although numbers of such transitions were small (e. g., males showed a 0.112 probability of transitioning from edge to interior and a 0.359 probability of transitioning from interior to edge; females showed a 0.037 probability of transitioning from edge to interior and a 0.077 probability of transitioning from interior to edge; Hannebaum et al. 2017, Table 3). Females showed higher survival than males and more fidelity to their habitat type. Oddly, hind foot size was positively related to the probability of transition; interpretations of this result can only be speculated upon. Assuming that transitions should be from lower- to higher-quality habitats, Hannebaum et al. (2017) interpreted their findings to imply edges were the better habitat. However, an alternate hypothesis is that greater transitions to edge by smaller males suggest this may be less-preferred habitat, as these could be the more subordinate individuals in a population and are more likely to be dispersers (Clobert et al. 2012). The approach to assessing habitat quality taken by Hannebaum et al. (2017) is intriguing, but motivations for transitions (acquiring better home ranges or coercion out of former ranges) needs further investigation. For example, Mergey et al. (2011) concluded that overrepresentation of subadult European pine martens (Martes martes) in fragmented forests indicated this was suboptimal habitat. Additional behavioral and demographic analyses to assess habitat quality would be interesting and informative.
Our finding of greater abundance of reproductive, adult females in forest interior supports the hypothesis that interior is higher-quality habitat than edges at our study site; the extensive forest at our site more closely resembles the study sites of Morris (1989) and Wolf and Batzli (2004) as well. It is likely that site-specific factors such as understory cover, diameter at breast height of canopy trees (Klein and Cameron 2012), food resources, and predation risk contribute to the variation observed in the studies cited above. At our site, a concurrent study of habitat use by black rat snakes (Elaphe obsoleta) and blue racers (Coluber constrictor), which are sometimes predators of small mammals, found greater use of edges than either forest or matrix interiors during summer due to the thermoregulatory advantages they offered (either basking or shading sites; Carfagno et al. 2006).
Conclusions. Our findings support the pattern of edge effects on abundance of P. leucopus, and perhaps other small mammals, only in small (e. g., < 2 ha) forest fragments (Anderson et al. 2003), and perhaps only seasonally (Wilder et al. 2005; Wilder and Meikle 2006). Peromyscus leucopus is the most abundant and widespread small mammal in Illinois (Hoffmeister 1989), and certainly not at risk of extinction. However, factors influencing the abundance and distribution of small mammals are still of concern for a variety of reasons. These include their potential influence on vegetation as seed predators (e. g.,Ostfeld et al. 1997), their role as potential predators of songbird nests (Maxson and Orig 1978; Schmidt et al. 2001), because they may attract the activity of predators who might then depredate nests incidentally (for reviews of edge effects on songbird nests, see Heske et al. 2001; Lahti 2001; Chalfoun et al. 2002), and because they are important reservoirs for the human pathogen Lyme disease (Lane et al. 1991, Allan et al. 2003) and viruses responsible for potentially fatal hantavirus pulmonary syndrome (e. g.,Monroe et al. 1999).
Edge effects on abundance, particularly negative relationships, have been widely demonstrated (Pfeifer et al. 2017) and remain a concern for the conservation of affected species (e. g.,Laurance et al. 2011). Although we did not detect edge effects on abundance at our study site, resource gradients and idiosyncratic attributes (e. g., biased distribution of downed woody debris) may produce edge effects in forest-dwelling small mammals at other locations. An important caveat of our study is that it was only conducted in one season (summer) of one year (although results supported conclusions of another study in southern Illinois in the same season, Heske 1995). Resource distributions and environmental conditions may change after mast production in fall or during cold periods in winter when edges may be more exposed (Wilder et al. 2005). However, without supporting data one should not assume that edge effects are a consistent, general feature of small mammal abundance.