Introduction
Prawns belonging to the genus Macrobrachium are widely distributed in tropical and subtropical regions of South America and inhabit rivers that drain into the Atlantic Ocean (New, 1980; Pileggi & Mantelatto, 2010). In Brazil, 3 native species of this genus have a significant commercial importance: Macrobrachium carcinus (Linnaeus, 1758), M. acanthurus (Wiegmann, 1836) and M. amazonicum (Heller, 1862). M. amazonicum is considered the freshwater prawn of greatest economic importance in the South American subcontinent (Maciel & Valenti, 2009; Valenti, 1985).
M. amazonicum has a wide ecological and morphological plasticity. They are found in continental and coastal environments, across a wide salinity gradient (Pileggi & Mantelatto, 2010; Rodriguez, 1982; Vergamini et al., 2011). According to Vergamini et al. (2011), the Brazilian populations of M. amazonicum can be categorized into 3 groups: continental hololimnetic populations in the Amazon region, populations in the Paraná and Paraguay basins, and coastal amphidromous populations in the north and northeast regions. It is speculated that some populations of M. amazonicum were introduced by anthropic action into the Upper Paraná (São Francisco basin) and the coastal river basins of northeastern and southeastern Brazil (Magalhães et al., 2005; Ramos-Porto & Coelho, 1998; Torloni et al., 1993; Vergamini et al., 2011). Due to their wide geographical distribution, many local populations are probably genetically isolated (Maciel & Valenti, 2009). In fact, one of the genetic groups of M. amazonicum proposed by Vergamini et al. (2011) has been already described as a new species, Macrobrachium pantanalenseDos Santos, Hayd and Anger, 2013.
In some populations of M. amazonicum, a morphological process occurs among males that results in the existence of different morphotypes. The main difference between them is related to the morphology of the second pair of pereopods (Moraes-Riodades & Valenti, 2004; Pantaleão et al., 2014). The existence of morphotypes affects the hierarchical social structure of these populations, as it results in the establishment of dominant and submissive males. Until recently, the presence of morphotypes was thought to be an exclusive characteristic of coastal populations (Dos Santos et al., 2013; Vergamini et al., 2011). However, the occurrence of this phenomenon in interior continental environments has been now recorded (Pantaleão et al., 2014; Paschoal et al., 2019). Thus, studies on the population ecology of such populations are essential, as they allow further comparisons with the other populations of M. amazonicum (Vergamini et al., 2011) and help to understand the phenotypic plasticity of this species.
Knowledge about reproductive traits such as fecundity, egg size, reproductive investment and reproductive period helps to evaluate the potential for aquaculture, reproductive potential, and stock sizes of natural populations (Lobão et al., 1985; Valenti et al., 1989; Vazzoler, 1982; Zimmermann et al., 2015). The reproduction of M. amazonicum can be influenced considerably by environmental factors in each geographical scenario such as distance from the sea. In fact, in continental habitats fecundity is influenced by the availability of dissolved salts and nutrients (Maciel & Valenti, 2009). In addition, knowledge on the reproductive investment of adult females is necessary to define reproductive cycles (Thessalou-Legaki & Kiortsis, 1997).
We analyzed the reproductive period, fecundity, egg size, and reproductive output of M. amazonicum, as well as the influence of environmental factors on these traits. The population under study has all 4 male morphotypes described for the species (Moraes-Riodades & Valenti, 2004), and it is located in a water reservoir in the municipality of Severínia, in the State of São Paulo, Brazil. M. amazonicum was introduced there to serve as food for fish. We hypothesized that their establishment in an isolated environment without connections with other rivers may have led to the divergence of reproductive characteristics, compared to populations from natural environments already studied, such as egg number and volume and reproductive output.
Materials and methods
The samplings were carried out monthly, during the morning, from May 2017 to April 2018, in the municipality of Severínia (20º49’31.92” S, 48º49’39.55” W; Fig. 1), São Paulo State, Brazil. The sampling site is a water reservoir (area = 132,985 m²) that has marginal vegetation along its entire border and is surrounded by a mosaic of forest fragments and sugarcane plantations; the sediment where sampling took place was muddy. The depth in the margins varies from 40 to 60 cm. The prawns were collected from the marginal vegetation using a 60 × 60 cm sieve (2 mm mesh), at depths up to 50 cm. The ovigerous females (= females carrying eggs) were separated and stored individually in ziplock bags with 70% ethanol, to maintain the egg mass intact until the fecundity analysis. The animals were transported to the Laboratory of the Biology of Marine and Freshwater Shrimps (LABCAM) in Bauru, SP. The water temperature was obtained with a mercury thermometer (0.1 °C), and the rainfall from the database of the Department of Water and Electricity of the State of São Paulo (DAEE-SP). The study region has 2 seasons, defined as: rainy, from October to March and dry, from April to September (Franchito et al., 2008).
The reproductive period was estimated based on the percentage of ovigerous females (OF) in relation to the total number of females per month. Twenty ovigerous females from each month were randomly selected for analyses of fecundity, egg size and reproductive output. In the months where less than 20 ovigerous females were found, all were used. Fecundity was taken as the number of eggs fixed in the abdomen (according to Anger & Moreira, 1998). The carapace length (CL) was taken as the distance between the orbital angle and the posterior margin of the carapace using a digital caliper (0.01 mm).
Developmental stages of embryos (fertilized eggs) were classified as follows: early, when eggs have a homogeneous color and lack visible eye pigmentation; intermediate, when eye pigmentation was barely visible; and late, with fully developed eyes (Anger & Moreira, 1998; García-Guerrero & Hendrickx, 2009; Wehrtmann, 1990) (Fig. 2). Fifteen embryos were carefully removed from the pleopods of each ovigerous female and measured (length and width) using a compound microscope with 100 x magnification (Leica ICC50 HD, Wetzlar, Germany). The Leica Application Suite (LAS) version 4.1.0 was used to acquire the images. The females were immersed in a hypochlorite solution (0.5%) and all embryos of each female were carefully extracted with fine forceps and counted. The fecundity of each female was calculated as F = total number of embryos per female.
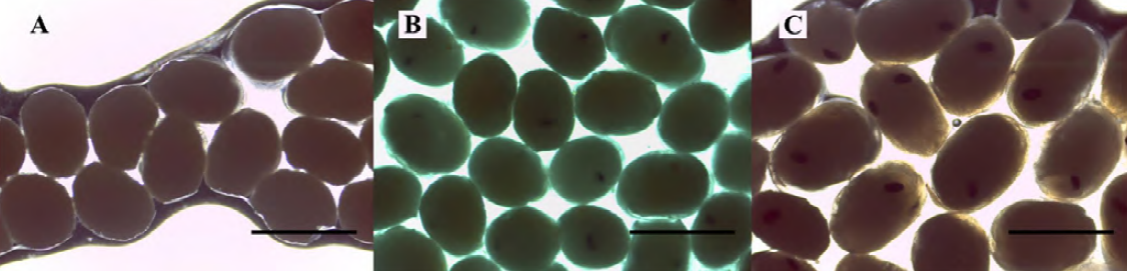
Figure 2. Macrobrachium amazonicum (Heller, 1862). Embryonic developmental stages: early (A), intermediate (B) and late (C). Bar scale: 0.8 mm
The egg volume (EV) was calculated as: EV = π * l * h *(h)²; where ‘‘l’’ is length; ‘‘h’’ width in mm and π = 3.14 (Wehrtmann, 1990). To estimate the reproductive output (RO) the whole egg mass and the females were dried at 60º for 48 hours. The RO was calculated by dividing the total egg dry mass (EW) by the dry mass of females without eggs (FW), which were determined with an analytical scale (accuracy of 0.0001 mg) (Clarke et al., 1991). The RO was estimated only for females carrying eggs in the early stage, since the mass gain during the egg incubation may lead to an underestimation of RO (Zimmermann et al., 2015). The estimate of egg loss was calculated by comparing the average number of eggs in the early and late stages of OF (Anger & Moreira, 1998).
The complete dataset was checked for normality using the Shapiro-Wilk test (Zar, 1999). The variation of environmental factors (rainfall and temperature) and total number of ovigerous females per season (dry or rainy) was compared using a T-test or Mann-Whitney test, when necessary. For the correlations (Spearman’s) with environmental factors, an adjusted fecundity was used in order to mitigate the effect of female size on fecundity, as proposed by Nicola and Almodóvar (2002) and Pantaleão et al. (2018). The relationship between fecundity and carapace length (CL) was assessed using linear regression by season and embryonic stage (early, intermediate, and late). Analysis of covariance (ANCOVA) was used to test for differences in fecundity between seasons and embryonic stages. In addition, the following relationships were investigated using linear regressions: EV (per embryonic stage) vs. CL, RO vs. CL, and FW vs. EW. All linear regressions were done using Statistica 7.0, StatSoft, Inc. The RO was compared between seasons using the Mann-Whitney test (Zar, 1999). The variation in egg volume between seasons was tested with the Kruskal-Wallis test, and Dunn's post-hoc test was used to determine which stages and seasons differed significantly from each other.
Results
There was a significant difference between the temperature of the rainy and dry seasons (U = 270, p < 0.001). The highest temperatures were recorded in the rainy season (24.85 ± 0.98 °C; 23.4-26.3 °C) while in the dry season the average was 22.45 ± 1.09 °C (21.4 -24.5 °C) (Fig. 3). The rainfall varied from 0 to 313.3 mm (107.6 ± 112.7 mm), and, as expected, differed significantly between seasons (Mann-Whitney, U = 0.89, p < 0.001). The average rainfall was 194.3 ± 91.02 mm in the rainy season (108.8-313.3 mm) and 20.9 ± 40.20 mm in the dry season (0-101.6 mm).
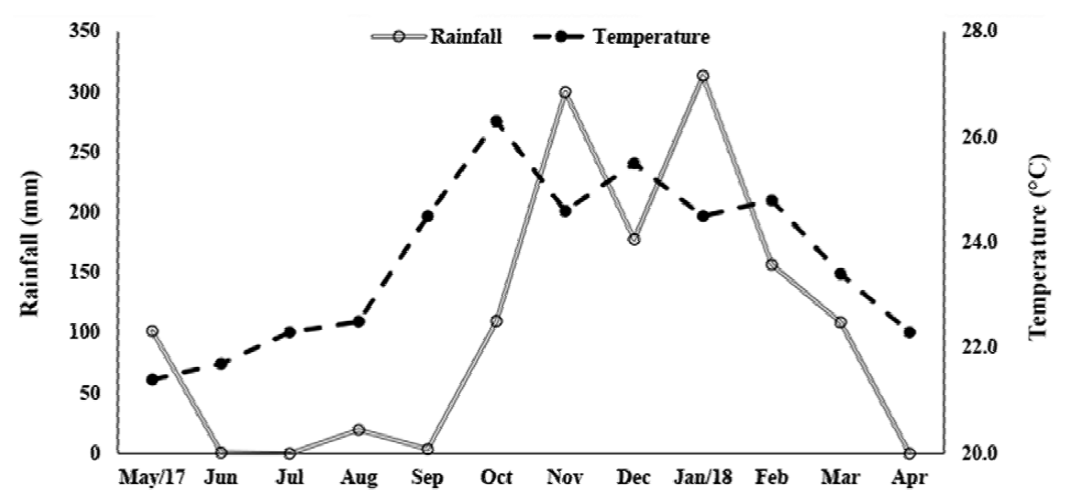
Figure 3. Monthly variation of rainfall and water temperature, from May 2017 to April 2018, in Severínia, São Paulo, Brazil.
In total, 1,412 females were collected, with 316 OF among them. The average female carapace length was 10.52 ± 2.27 mm (4.4-17 mm, Fig. 4). The highest OF relative abundance was observed in July (50.96%), May (49.35%), and September (46.34%) (Fig. 5). OF were significantly more abundant in the dry season (dry = 205, rainy = 111; T test, t = 2.23, p < 0.05) (Fig. 5). There was no correlation between OF abundance and environmental factors (Spearman, p < 0.3).
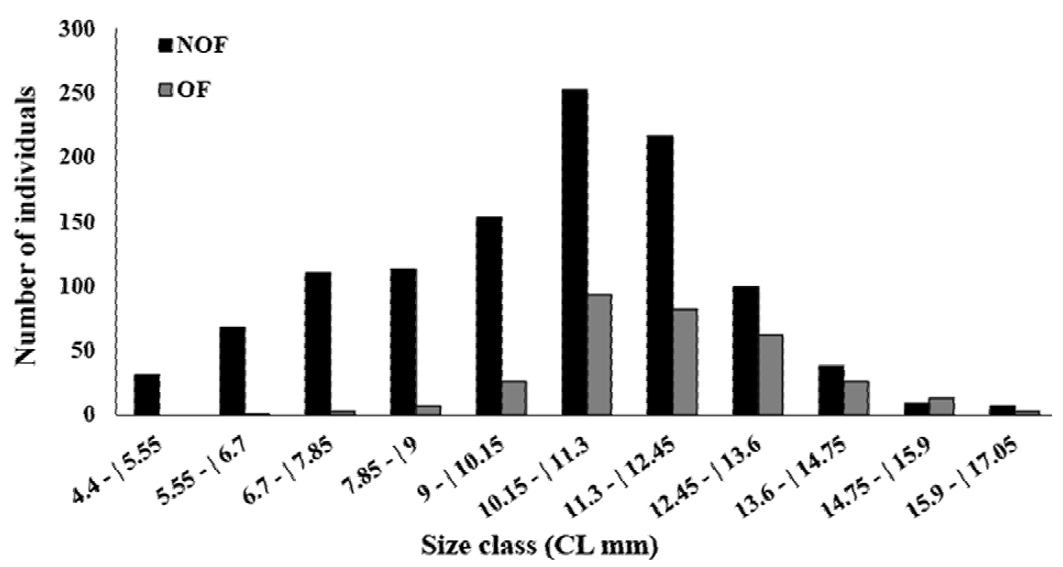
Figure 4. Size-frequency distribution of the carapace length of the non-ovigerous females (NOF) and ovigerous females (OF) of Macrobrachium amazonicum caught in Severínia, São Paulo, Brazil.
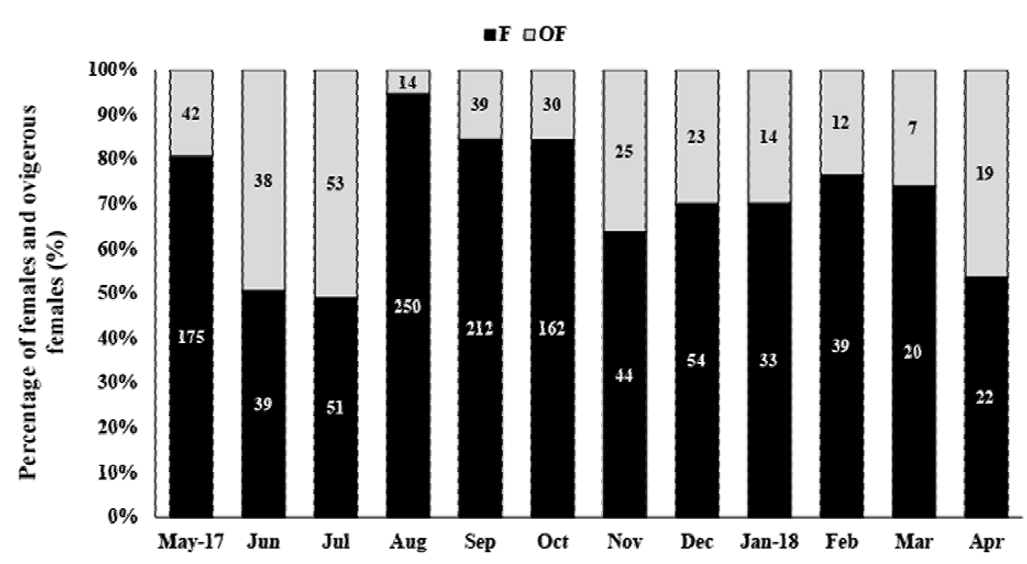
Figure 5. Macrobrachium amazonicum (Heller, 1862). Relative frequency of ovigerous females (OF) and non-ovigerous females (F), from May 2017 to April 2018, in the water reservoir of Severínia, São Paulo, Brazil. The numbers above the bars indicate the absolute number of OF + F per month.
A total of 204 ovigerous females were considered for the fecundity analysis, with 104 of these bearing early stage, 29 with intermediate, and 71 with late stage embryos. The months that had less than 20 organisms were August/2017, and January-April/2018 (Fig. 5). The average carapace length of ovigerous females was 11.88 ± 1.71 mm (8.57-15.35 mm) in the rainy season, and 12.15 ± 1.97 mm (6.86-17 mm) in the dry season, but these differences were not significant (Mann-Whitney, U = 4738, p > 0.05). There was a significant correlation between carapace length and fecundity across the embryonic stages and seasons (linear regression, p < 0.001) (Fig. 6). The overall average fecundity was 600 ± 306 eggs (92-1,692 eggs), and it was not correlated with environmental factors (rainfall and water temperature) (Spearman, p < 0.3). However, fecundity varied significantly between seasons (ANCOVA, p < 0.05), with females bearing more eggs in the dry season: 613 ± 306 eggs (121-1,692 eggs) and 585 ± 304 eggs (92-1,351 eggs) in the rainy season (Table 1). There was no significant difference in the number of eggs that the females incubated during the embryonic development (Table 2, Fig. 6). The average number of eggs per embryonic stage was 624 ± 306 (early), 621 ± 307 (intermediate), and 558 ± 300 (late) embryos. There was a 10.6% decrease in the number of eggs, i.e. egg loss, between the early and late stage.
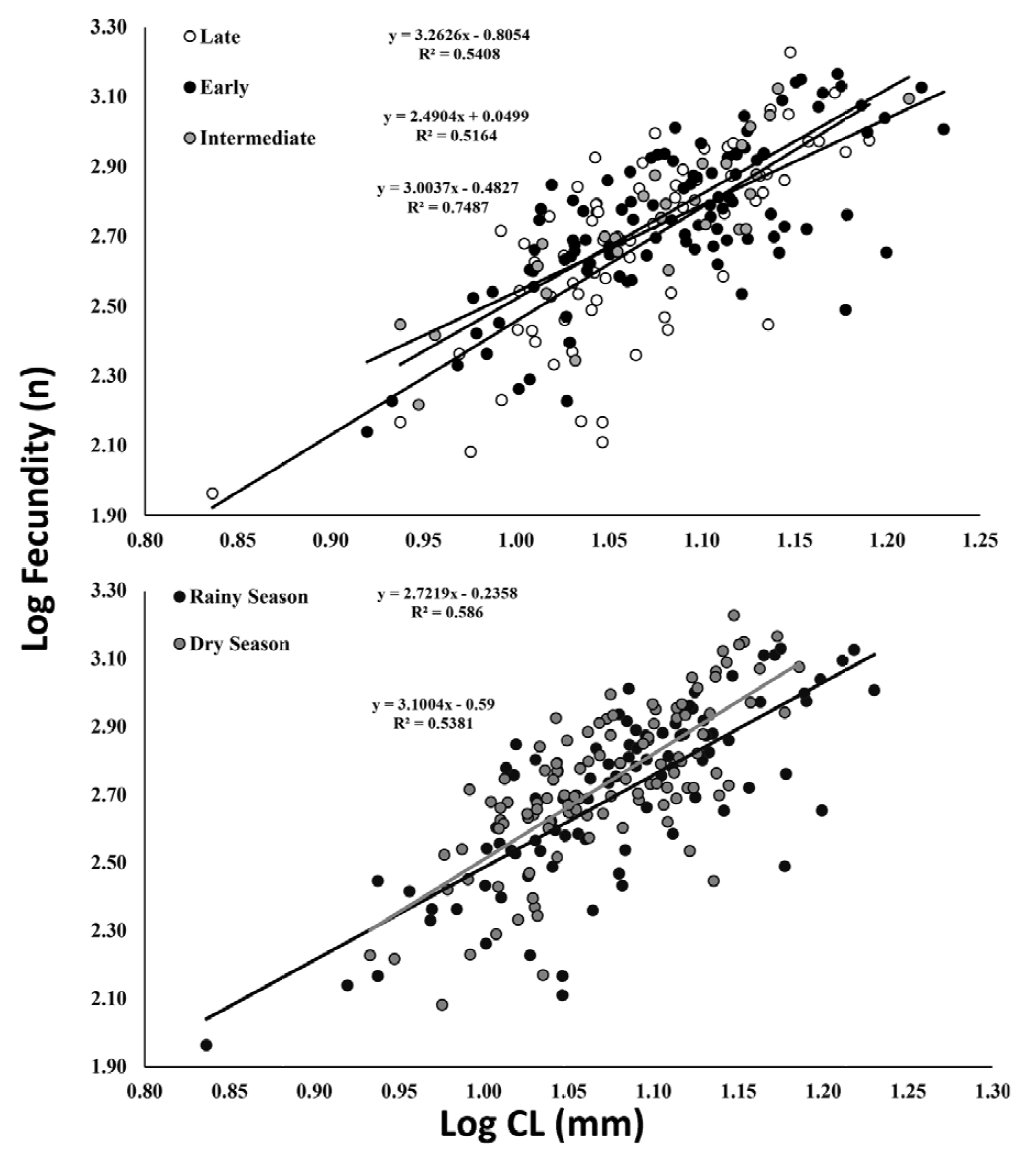
Figure 6. Macrobrachium amazonicum (Heller, 1862). Relationship between fecundity and carapace length of females (CL), by embryonic developmental stages and season.
Table1. Macrobrachium amazonicum (Heller, 1862). Size-class distribution of ovigerous females per season. For each size class, the fecundity and frequency of females according to the embryonic developmental stage (EDS) is also shown. Results expressed as mean ± standard deviation. Embryonic developmental stages: E = early, I = intermediate, L = late.
Season | Size classes (mm) | N | Mean fecundity | Minimum fecundity | Maximum fecundity | EDS | ||
Rainy | 6.86 - | 8.01 | 1 | 92 | - | - | - | - | L: 100% |
8.01 - | 9.16 | 4 | 207 ± 74 | 138 | 280 | E: 25% | I: 50% | L: 25% | |
9.16 - | 10.31 | 11 | 319 ± 122 | 183 | 601 | E: 54% | I: 36% | L: 9% | |
10.31 - | 11.46 | 18 | 393 ± 163 | 129 | 705 | E: 28% | I: 6% | L: 67% | |
(n = 92) | 11.46 - | 12.61 | 24 | 590 ± 200 | 229 | 1,028 | E: 39% | I: 13% | L: 48% |
12.61 - | 13.76 | 18 | 727 ± 156 | 385 | 1,006 | E: 50% | I: 11% | L: 39% | |
13.76 - | 14.91 | 7 | 906 ± 349 | 450 | 1,293 | E: 43% | - | L: 57% | |
14.91 - | 16.06 | 7 | 818 ± 378 | 309 | 1,351 | E: 86% | - | L: 14% | |
16.06 - | 17.21 | 3 | 1,201 ± 166 | 1017 | 1,340 | E: 67% | I: 33% | - | |
Dry | 6.86 - | 8.01 | 0 | - | - | - | - | - | - |
8.01 - | 9.16 | 2 | 167 ± 3 | 165 | 169 | E: 50% | I: 50% | - | |
9.16 - | 10.31 | 15 | 349 ± 131 | 121 | 559 | E: 60% | - | L: 40% | |
10.31 - | 11.46 | 33 | 464 ± 154 | 148 | 843 | E: 48% | I: 18% | L: 33% | |
(n = 111) | 11.46 - | 12.61 | 23 | 655 ± 177 | 375 | 991 | E: 65% | I: 17% | L: 17% |
12.61 - | 13.76 | 26 | 704 ± 246 | 280 | 1,157 | E: 50% | I: 27% | L: 23% | |
13.76 - | 14.91 | 10 | 1,167 ± 395 | 500 | 1,692 | E: 70% | I: 10% | L: 20% | |
14.91 - | 16.06 | 2 | 1,034 ± 223 | 876 | 1,191 | E: 50% | - | L: 50% | |
16.06 - | 17.21 | 0 | - | - | - | - | - | - |
Table 2. Results of the analysis of covariance (ANCOVA) between female size (CL) and fecundity by season, and by embryonic developmental stage.
Relationship | Group | Par. (log) | F | P |
Season | Dry vs. rainy | a | 5.41 | 0.02 |
b | 1.06 | 0.3 | ||
Stages | Early vs. intermediate | a | 0.41 | 0.52 |
b | 1.08 | 0.3 | ||
Early vs. late | a | 1.11 | 0.29 | |
b | 3.42 | 0.07 | ||
Intermediate vs. late | a | 1.48 | 0.23 | |
b | 0.18 | 0.67 |
The reproductive output differed between seasons (Mann-Whitney, U = 780, p < 0.05) and was higher in the dry season, 13.9 ± 5.4% (3.23-28.9%) than in the rainy season, 12.5 ± 4.8% (3.23-28.9%) (Table 2). The mean egg volume, according to the embryonic stage, was 0.11 ± 0.02 mm³ (early), 0.15 ± 0.04 mm³ (intermediate), and 0.18 ± 0.03 mm³ (late), in the rainy season. In the dry season, it was 0.11 ± 0.02 mm³ (early), 0.13 ± 0.03 mm³ (intermediate) and 0.14 ± 0.04 mm3 (late). The average volume of late-stage eggs differed significantly between seasons (Kruskal-Wallis, p < 0.001; Table 3). There was no significant correlation between EV (in any stage) and CL, between RO and CL, and between FW and EW (linear regression, p > 0.05).
Table 3. Macrobrachium amazonicum (Heller, 1862). Comparison of egg volume between embryonic developmental stages and seasons. The values are Dunn’s test results; ns = non-significant.
Dry season | Rainy season | ||||||
Stages | Early | Intermediate | Late | Early | Intermediate | Late | |
Dry season | Early | - | ns | > 0.001 | ns | > 0.05 | > 0.001 |
Intermediate | ns | - | ns | > 0.05 | ns | ns | |
Late | > 0.001 | ns | - | > 0.001 | > 0.05 | > 0.05 | |
Early | ns | > 0.05 | > 0.001 | - | 0.001 | > 0.001 | |
Rainy season | Intermediate | > 0.05 | ns | > 0.05 | 0.001 | - | ns |
Late | > 0.001 | ns | > 0.05 | > 0.001 | ns | - |
Discussion
Contrasting data on reproductive traits were obtained for a hololimnetic population of M. amazonicum from an environment without connections with the surrounding rivers. An unusual pattern was observed in this population: higher fecundity and reproductive output in the dry season instead of in the rainy season, which is when the higher reproductive effort normally occurs in this species (Bialezki et al., 1997; Lima et al., 2014; Lucena-Frédou et al., 2010; Pantaleão et al., 2018). Moreover, even though reproduction was characterized as continuous, as reported for other M. amazonicum populations (Bialezki et al., 1997; Costa e Silva et al., 2019; Lima et al., 2014; Lucena- Frédou et al., 2010; Pantaleão et al., 2018), the reproductive peak occurred in the dry season, and not in the rainy season, which is more common.
For various aquatic organisms, from cnidarians to vertebrates, including crustaceans, the intensity of reproductive effort is correlated with the primary productivity of the environment and, consequently, with the availability of food for larvae and juveniles (Gori et al., 2012; Kamal et al., 2020; Liberman et al., 2018; Nicola & Almodóvar, 2002; Pantaleão et al., 2018). In freshwater environments, the rainy season normally has a higher primary productivity, which favors a greater reproductive effort (Pantaleão et al., 2018). However, as our study site was located among sugarcane plantations, it may have been influenced by the discharge of fertilizers used in these monocultures that altered the natural fluctuations in productivity. It is common in this type of plantation to use fertigation (which consists of using vinasse and other effluents for irrigation) which can input large amounts of organic matter to the water table and water bodies (Kiang et al., 2017; Martineli & Filoso, 2008).
The average fecundity of M. amazonicum recorded in this study, 600 eggs per female, is relatively low compared to other hololimnetic populations that also have male morphotypes, where the average varies from 814 to 921 eggs, once in these populations the females also attain bigger carapace sizes (Pantaleão et al., 2018; Paschoal et al., 2019). These results reflect the biological plasticity of M. amazonicum, especially when taking into account that amphidromous populations of M. amazonicum have a maximum fecundity of 5,706 eggs, with an average of 2,237 eggs (Meireles et al., 2013; Odinetz-Collart & Magalhães, 1994). These differences may be related to the geographic isolation of these populations, which could result in an incipient speciation process.
Compared with the other species of Macrobrachium that have extended larval development and occur in Brazil, we observed that hololimnetic populations of M. amazonicum have the lowest fecundity, together with M. surinamicum Holthuis, 1948, which has up to 4,264 eggs per female (Pantaleão et al., 2018). On the other hand, M. amazonicum (amphidromous populations), and the amphidromous species M. acanthurus, M. carcinus, M. heterochirus (Wiegmann, 1836) and M. olfersii (Wiegmann, 1836) have a maximum fecundity between 7,417 and 242,473 eggs (Anger & Moreira, 1998; Bertini & Baeza, 2014; Lara & Wehrtmann, 2009; Lima et al., 2014, 2015; Mejía-Ortiz et al., 2001). In crustaceans, female size and fecundity are known to be highly correlated (Somers, 1991). Thus, the fact that M. acanthurus, M. carcinus, M. heterochirus and M. olfersii are larger than M amazonicum and M. surinamicum (Anger & Moreira, 1998; Bertini & Baeza, 2014; Lara & Wehrtmann, 2009; Lima et al., 2014; 2015; Mejía-Ortiz et al., 2001), could explain these large differences in fecundity.
The decrease in the number of eggs during the incubation period is a common characteristic of crustaceans, which can affect the reproductive potential of several species. It may be caused by parasitism or by environmental stressors that can affect the females (Balasundaram & Pandian, 1982; Kuris, 1991; Oh & Hartnoll, 2004; Perkins, 1971). In this study, there was no significant egg loss over the incubation period, similar to Macrobrachium lanchesteri (de Man, 1911), which had an egg loss of 10-20% (Phone et al., 2005). In other species, such as M. acanthurus, M. nobilii (Henderson & Matthai, 1910), M. olfersii, M. potiuna (Müller, 1880) and M. tenellum (Smith, 1871), values above 20% have been recorded (Anger & Moreira, 1998; Balasundaram & Pandian, 1982; Nazari et al., 2003; Vargas-Ceballos et al., 2018). In addition, no parasites or possible stressors that could affect the fecundity were detected, which explains the low eggs loss. The egg loss during the embryonic development may also be caused by removal of non-viable eggs, wear and tear due to contact with substrate, and/or increase in egg volume during the development (Oh & Hartnoll, 1999).
Fecundity was not correlated with environmental factors such as rainfall or water temperature, however, it varied significantly between seasons, as did the egg volume. In the dry season, fecundity was higher and the eggs were smaller, whereas the opposite occurred in the rainy season. This negative correlation between fecundity and egg volume is well-known from other studies (Maciel & Valenti, 2009; Meirelles et al., 2013). Since females produce more eggs during the dry season, these need to be smaller due to the space available in the abdomen. In addition, the relationship between egg number and size can reflect some characteristics of the environment such as primary productivity (Clarke, 1993; Hancock, 1998; Mashiko, 1990). All larvae need food resources after hatching, so the fluctuation of primary productivity rates throughout the year may explain the differences in egg volume between seasons. The strategy of ovigerous females may be to invest in a greater amount of yolk in times of low primary productivity, and vice-versa (Clarke, 1993; Hancock, 1998).
In this M. amazonicum population, as in other species of the family Palaemonidae, the reproductive output was not related to the female size (Anger & Moreira, 1998; Mantel & Dudgeon, 2005; Pantaleão et al., 2018; Zimmermann et al., 2015). The observed reproductive output is within the average reported for some species of Palaemonidae (10.5-26.3%, see Zimmermann et al., 2015), with values very close to the lowest reported for individuals of this family. However, when compared to other congeners (Table 4), the reproductive output of M. amazonicum is very similar to that of most species of Macrobrachium. The small variation that exists between these species can also be a consequence of several environmental characteristics related to the nutritional condition of the females and availability of food, which can directly influence the reproductive output over the seasons (Clarke et al., 1991; Sastry, 1983).
Table 4. Average, minimum and maximum reproductive output (RO) of species of the genus Macrobrachium.
Species | RO mean (%); (min-max %) | Reference |
M. acanthurus | 19 (14-30) | Anger and Moreira (1998) |
M. amazonicum | 13.3 (3.2-28.8) | Present study |
M. amazonicum | 17.7 (4.8-21.8) | Lima et al. (2014) |
M. amazonicum | 10.3 | Meireles et al. (2013) |
M. amazonicum | 7.4 | Pantaleão et al. (2018) |
M. carcinus | 12 (4-21) | Lara and Wehrtmann (2009) |
M. haianense | 10.5 (3.7-17.1) | Mantel and Ddgeon (2005) |
M. lamarrei lamarrei | (6.8-39.4) | Hussain and Manohar (2016) |
M. macrobrachion | 14 | Koussovi et al. (2019) |
M. nipponense | (6-12) | Mashiko (1983) |
M. olfersi | 22 (7-38) | Anger and Moreira (1998) |
M. surinamicum | 14.3 (4.3-35.5) | Lima et al. (2015) |
M. tenellum | 8.5 (4.1-16) | Vargas-Ceballos et al. (2018) |
We conclude that the habitat restrictions imposed on this hololimnetic population, which is isolated from the fluvial continuum, may be affecting their reproduction and leading to different reproductive patterns, compared to those of non-isolated populations.