Introduction
For many years the idea to replace oil-based plastics by biodegradable materials has attracted the attention of specialists and several alternatives have been promoted (Netravali, & Chabba, 2003). One of these alternatives is materials based on fungal mycelium composites. This novel kind of agglomerates is made with lignocellulosic particles using a mycelial matrix as binder agent. Colonized particles are molded, pressed, and dried, obtaining agglomerates with a desired form (Jones, Huynh, Dekiwadia, Daver, & John, 2017). Materials obtained are relatively light and compostable, which made them appropriate for ephemeral uses. One convenient feature in this matter is the feasibility of to take advantage of agro-industrial wastes as substrates like barley and wheat straw, cotton gin, soy husks, sugar cane bagasse or corn stover among others, that could represent a pollution problem when burned, rot in situ or threw on water bodies (Chen, Yang, & Zhang, 2009; Chen, 2014).
Previous works show the functionality of materials based on mycelium composites as acoustic insulators, construction or packing materials, and even substitutes of leather or fabrics (Pelletier, Holt, Wanjura, Bayer, & McIntyre, 2013; Pelletier et al., 2017; Roman-Ramos, Luna-Molina, & Bailón-Pérez, 2014; Dschida, 1997). Currently, these kinds of materials are produced in an industrial way by some companies (https://ecovativedesign.com/), also designers and architects are involved in the development of this technology and its applications (Camere & Karana, 2018). Albeit the potential for improving this type of fungal application is important, research on this topic is still scarcely published. Some studies have evaluated properties performing tests in the materials to determine variables like compressive strength, acoustic insulation, modulus of elasticity, dimensional stability, and others (Jones et al., 2017; Tudryn, Smith, Freitag, Bucinell, & Schadler, 2017; Yang, Zhang, Still, White, & Amstislavski, 2017). In some cases, size and shape of the test specimens did not follow standardized specifications even when this is critical for more accurate comparisons.
A variable of importance should first be the selection of the fungal species for the mycelial matrix and the characteristics to choose them. Ganoderma lucidum and Pleurotus ostreatus have been largely used in various works for different purposes (Holt et al., 2012; López, Méndez-González, Ruelas, & Nájera, 2016; Haneef et al., 2017; Jones et al., 2017; Pelletier et al., 2017). Coriolus versicolor (Lelivelt, 2015), Irpex lacteus (Yang et al., 2017) and Daedaleopsis confragosa-Ganoderma resinaceum (Bajwa, Holt, Bajwa, Duke, & McIntyre, 2017) have also been studied. Based on the kind of hyphal systems (Corner, 1932), the selection of taxa with a trimitic hyphal composition could be adequate to create composites with stronger structure and a more resistant composition than other with dimitic or monomitic hyphal system, considering the inner features exhibited by species with such anatomy.
In a previous research, César (2018) made puncture resistance tests on some strains isolated from wild mushrooms. A strain of L. crinitus ECC16, after evaluations, was selected to develop fungal composites. The species produces a trimitic hyphal system which could be an adequate characteristic used for such purposes. Besides its hyphal characterization, L. crinitus is distributed worldwide, it has a steady growth on PDA medium (Petersen, Nicholl, & Hughes, 1997; Senthilarasu, 2015) and creates mycelium matrices with a leathery texture.
The processes to manufacture mycelium composites are diverse and still improving. The compression forces to which the mycelium matrices are exposed before drying is a variable that could be important to evaluate in order to improve mechanical characteristics and is a part of the fabrication method that previous works had not mentioned explicitly. In this work, mycelial biocomposites made with barley straw and a L. crinitus strain were fabricated and mechanically tested following the guidelines established in ASTM D 143 specifications for Small Clear Specimens of Timber. Two different loads were used to compress the composites before drying them. Obtained materials were tested accordingly to the followed standards.
Objectives
To determine if a pressing protocol of the composites in the fabrication process modifies the results of compressive and flexural strength obtained in mechanical tests.
Materials And Methods
Strain and spawn preparation
The L. crinitus strain ECC16 was used to develop the mycelial matrix. The strain used was conserved in PDA medium covered with sterilized mineral oil at room temperature, and it was taxonomically identified by molecular methods (César, 2018) and kept in Laboratorio de Biodiversidad y Sistemática, Instituto de Ecología A.C. To develop a new culture from the conserved one, little portions of mycelia were taken and washed carefully in sterilized distilled water and put on PDA Petri dishes. Once the mycelium covered approx. 70% of the plate, it was transferred to liquid media. The liquid media used (2.5 g saccharose, 2.5 g potato extract, 250 ml distilled water) was sterilized and cooled following the protocol of Chang and Miles (1989). Four pieces of 0.5 cm2 of colonized agar were introduced in Erlenmeyer flasks with 250 ml of liquid media and shook at 120 rpm for 72 hrs. Spawn was made with sorghum grains, washed, and sterilized at 121 °C for 1.5 h and inoculated with liquid media (7 days of development). Once cooled, plastic bags with 250 g of grain were inoculated with 15 ml of liquid media and placed in darkness seven days at 25 °C for incubation.
Substratum preparation
Barley straw was used as substrata. Packs without fungal contamination were selected and chopped with an animal feed shredder to obtain straw pieces between 10 mm to 80mm.Straw was moistened for 12 h, then pasteurized at 60 °C for 1 h. Later it was cooled and conditioned to 70% moisture. The inoculated straw with 10% spawn was placed in transparent plastic bags, lightly compacted with the hands and incubated for seven days at 25 °C. At day eight, casts were filled with the colonized straw, cover with a plastic mat and incubated for seven days more.
Composites fabrication
After the seven incubation days, the resulting composites without fungal contamination were pressed with two different loads, group G1 (n=6) was loaded with 3922.66 Pa for two hours and group G2 (n=6) was pressed with 17651.97 Pa for two hours. The loads were selected observing the behavior of the straw to the load. The G1 represent the minimum compression in which the fibers maintain the form after unload. G2 was selected when the compression fills the inner gaps of the composites. These pressed materials were dried at 45 °C for 72 h. After drying, specimens were cut to approx. 3 cm width and 30 cm length avoiding empty spaces or hypoxic sites with poor mycelial growth (Fig.1). Posterior to cutting specimens, dimensions and weight were recorded. Specimens of each composite were submerged in water to evaluate water absorption after two periods, 2 h and 24 h
Mechanical testing
A three-point bending test with a 288 mm span was performed using a Universal Test Machine INSTRON 3385H with the Bluehill II software, with a 5 kN load cell (1 N resolution), a loading rate of 1.3 mm/min ± 25% and load control following ASTM D-143 standard test methods for small clear specimens, section 7, for flexural strength and section 8 for parallel compression (Fig. 1). Stress-strain curves are showed on figure 2. Tests for expanded polystyrene specimens were also made following the ASTM C 578-04 standards in order to compare results. A Tukey test was carried out to compare results between groups and computed with SigmaPlot version 10.0.
Results And Discussion
The results show differences between values obtained from G1 and G2 composites (Table 1). Mean values of Modulus of rupture (MOR) for the G1 composites are 0.11 MPa ± 0.04 MPa and for G2 of 0.48 MPa ± 0.10 MPa (Fig. 3c). The same trend was observed for maxim compressive strength (fcmax) in which G2 composites presented 0.235 MPa ± 0.068 MPa in comparison with 0.097 MPa ± 0.043 MPa of G1. Regarding the modulus of elasticity (MOE), in G1 was 9 MPa ± 5.42 MPa, while in G2 was 26 MPa ± 5.19 MPa (Fig. 2, 3). Statistical difference was found between G1 and G2 in all tests performed. Data obtained for expanded polystyrene was not statistically different for flexural strength and elastic modulus of G1 composites, showing that mechanical properties were very similar.
Table 1 Results of the different tests for the G1 composites (pressed with 3.92 kPa), G2 composites (pressed 17.65 kPa), expanded polystyrene and balsa wood.
Marerial | Flexural strength (MOR) (MPa) | Compressive strength parallel to grain (fcmax) (MPa) | Modulus of Elasticity (E) (MPa) | Density (kg /m3) | Water absorption (% 2 h / 24 h) |
G1 composites | 0.11 ± 0.04 a | 0.097± 0.043 a | 9± 5.42 a | 102± 4a | 426.16±22.08 / 526.66±30.29ª |
G2 composites | 0.48 ± 0.10 b | 0.235 ± 0.068 b | 26± 5.19 b | 141 ± 11b | 592.5±46.66 / 710.5±59.30b |
Expanded Polystyrene | 0.084± 0.014 a | 0.035(ASTM C 578-04) | 3.952± 0.341 a | 10.83±0.55c | 4 (ASTM C 578-04) |
Balsa Wood-light density (Kotlarewski et al. 2016) | 9.83 ± 1.72 | 9.24 ± 0.61 | 1222.14 ± 246.93 | 80-120 | 31.16±7.67 / 74.66±17.99 |
According to its density, EPS type XI is compared. Letters indicate significant differences between groups.
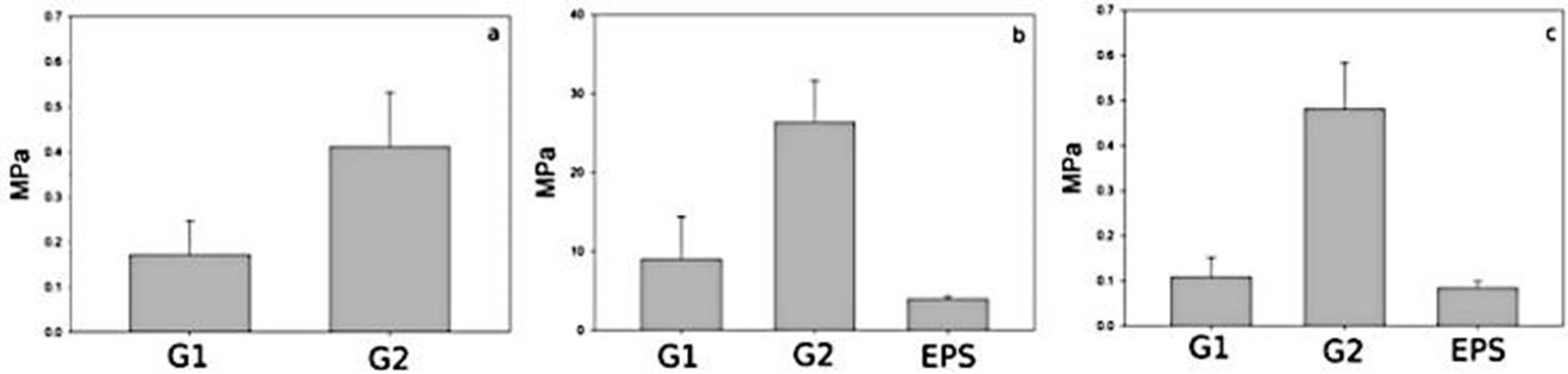
Figure 3 Values of performance obtained in this work. a) Compressive strength parallel to grain (fcmax) of mycelial composites. b) Modulus of elasticity values for mycelium composites and expanded polystyrene. c) Flexural strength (MOR) for mycelium composites and expanded polystyrene.
Balsa wood is a natural product that could be similar to mycelium composites. Data from Kotlarewski, Belleville, Gusamo, & Ozarska, (2016) for balsa wood show that the differences in mechanical properties obtained for balsa wood are important and difficult to compare with the materials here developed. Only density values are similar to the obtained here, but the water absorption differs markedly.
Comparing the results with those from other works, groups 1 and 2 evidence similarities in MOR values with Tudryn et al. (2017) and fcmax values with Roman-Ramos et al. (2014). Data for MOE in previous works (Table 2) differs in all cases. Density of the products is also diverse, showing that different fabrication protocols influence greatly the final properties. The composites of the G2 group exhibit higher properties in comparison with composites obtained by Holt et al. (2012) and López et al. (2016), the first developed with a mycelial matrix of Ganoderma sp., cotton gin waste as reinforcement and following different protocols of inoculation, that did not differ greatly between them. The studied composites show characteristics comparable with materials used for fabrication of insulation panels. Previous works have showed the feasibility to substitute EPS in some applications. In this study, the mechanical performance of G2 composites showed higher characteristics than EPS, G1 composites are very similar. The water absorption by mycelial composites developed in this study like others is very high in comparison with synthetic materials, which could be an impediment for applications especially in food packaging. For ephemeral uses in a wide range of applications, mycelium composites could be used, distinctly in industrial sectors that uses foams that must have low density, low price and adequate mechanical performance like the automotive industry for some insulation parts. This kind of composites could be a good alternative for replacement EPS used in construction due to low fabrication price and green ecological footprint. In comparison to others works, data from Yang et al. (2017) shows excessive higher compressive strength in comparison with the values obtained here and others included in Table 2. The use in that work of a specific fungal species could be the reason and the combination of Irpex lacteus with other substrata would be interesting to assess.
Table 2 Mechanical properties of fungus-based materials published in literature.
Flexural strength | Compressive strength | Modulus of | Density | Water | |
Reference | (MOR) | parallel to grain (fcmax) | Elasticity (E) | absorption | |
(kg/m 3) | |||||
(MPa) | (MPa) | (MPa) | (%) | ||
López et al. (2016) | 0.01091 ± 0.00441 | 0.04172 ± 0.01349 | No data | 183.8 ± 15.1 | 268.4 ± 80.4 |
Holt et al. (2012) | 0.007 - 0.0261 | 0.0011 - 0.0722 | 0.1228 - 0.6745 | 66.5 - 224 | 10.8 - 48.0 |
Yang et al. (2017) | No data | 0.35 - 0.57 | 15-60 | 240 - 265 | No data |
Tudryn et al. (2017) | 0.3 - 1.3 | No data | No data | No data | No data |
Ziegler et al. (2016) | No data | No data | 66.14 - 71.773 | No data | 300 |
Román-Ramos et al. (2014) | No data | 0.054 - 0.139 | No data | 450 - 550 | No data |
Appels et al. (2019) | 0.05 - 0.86 | No data | 2-97 | 100 - 240 | 43 - 508 |
Silvermann, 2018 | No data | 0.124 - 0.34 | No data | 285.5 - | No data |
353.9 |
Conclusions
As part of the fabrication protocols of mycelium composites, compression of materials after fungal colonization has shown to be a variable of importance as an indicator of more resistant composites. Higher loads could be tested but reaching a certain point a decrease in resistance is expected. Other characteristics that must be tested individually are the direction of fibers used as reinforcements and the size of these particles to generate better results. In this work, only the parallel to grain compressive strength was measured with a perpendicular load due to the heterogeneity of the obtained mycelium boards, a perpendicular to grain test would be suitable for more homogeneous samples with less than 25 mm width. Besides that, and taking into consideration the results of other works, the use of a specific fungal species must be carefully studied because a considerable percentage of the composite is represented by hyphal nets and this simple change modifies the entire structure.