Introduction
Phytophthora species (> 60) are a threat to different agronomically and ecologically important plants, causing diseases such as wilting or rotting of root, crown, stems, leaves and fruits (Drenth & Guest, 2004; Jung et al., 2018). Among these species, Phytophthora capsici (PC) is considered a destructive pathogen that affects agricultural crops such as cucurbits, eggplant (Solanum melongena L.), tomato (Solanum lycopersicum L.) and pepper (Capsicum annuum L.) (Lamour, Stam, Jupe, & Huitema, 2012). In Mexico, pepper is one of the main horticultural crops of economic importance. In 2018, Mexico ranked as the world’s second largest pepper producer and exporter, producing nearly 3.4 million tons with a value of US $844 million (Servicio de Información Agroalimentaria y Pesquera [SIAP], 2019). Despite the above, diseases due to phytopathogens, such as wilt caused by PC, have affected the productivity of the pepper crop.
Phytophthora spp. zoospores are the organism’s main propagule for survival, dispersal and infection; moreover, due to their motility, they can actively locate their hosts (Judelson & Blanco, 2005; Stanghellini, Kim, Rasmussen, & Rorabaugh, 1996). The use of chemical fungicides has been the main method of PC control; however, their constant use increases the risk of pathogen resistance (Wu et al., 2020). Therefore, other alternatives are required that, as a whole, allow for an integrated pest and disease management strategy focused on the development of sustainable agriculture (Lamichhane et al., 2017). In recent decades, the use of antagonistic microorganisms for disease control in plants has proven to be an alternative because microorganisms are specific to a phytopathogen, harmless to both non-target species and humans, and environmentally friendly (O’Brien, 2017).
Actinobacteria (commonly called actinomycetes) have potential as biological control agents, as they have the ability to inhibit or reduce the growth of plant pathogens through direct and indirect mechanisms such as the production of antibiotics, lytic enzymes, hyperparasitism, competition and induction of systemic resistance in plants (Kurth et al., 2014; Sharma & Salwan, 2018). Actinobacteria have demonstrated their in vitro potential as antagonists for different Phytophthora species.
Sadeghi, Koobaz, Azimi, Karimi, and Akbari (2017) demonstrated that Streptomyces spp. strains inhibit mycelial growth of P. drechsleri by 30 to 78 %. Fonseca-Ardila, Castellanos-Suárez, and León-Sicard (2011) report that actinobacterial isolates from fermented extracts of chipaca (Bidens pilosa L.) inhibit mycelial growth of P. infestans by 33 to 77 %. Arfaoui, Adam, Bezzahou, and Daayf (2018) isolated bacteria (100 isolates) from the rhizoplane of soybean (Glycine max [L.] Merr.) plants and found that nine isolates, belonging to the genera Streptomyces spp. (3), Bacillus spp. (4), Paenibacillus sp. (1) and Lysobacter sp. (1) reduced the percentage of mycelial growth of P. sojae by 14 to 85 %. Chen, Chen, and Tsay (2016) reported the antagonistic activity of S. plicatus B4-7 against several Phytophthora species, and Evangelista-Martínez (2014) demonstrated the antagonistic potential of Streptomyces sp. CA-CIS-1.16CA (isolated from the soil of the "Los Petenes" biosphere reserve) against several phytopathogenic fungi, among them PC.
Actinobacteria are a prominent source of natural products. According to Bérdy (2012), actinobacteria account for 40.8 % of all bioactive metabolites of microbial origin. Nguyen et al. (2015) demonstrated that pyrrolidone carboxylic acid, isolated from Streptomyces griseus H7602, reduced PC mycelial growth from concentrations of 8 to 128 µg∙mL-1. In similar studies, Joo (2005) and Chen et al. (2016) evaluated the activity of the cell-free culture broth of S. plicatus B4-7 and S. halstedii AJ-7, respectively; in in vitro tests, both cultures inhibited the germination of PC zoospores. Lee, Moon, Yun, Yoo, and Hwang (2004) isolated the natural product thiobutacin from Lechevalieria aerocolonigenes VK-A9, a compound that inhibited the in vitro germination of PC zoospores at a concentration of 10 µg·mL-1. Therefore, the objective of this work was to evaluate the effect of Streptomyces spp. in the biological control of Phytophthora capsici under in vitro and in vivo conditions.
Materials and methods
Plant material
Serrano pepper var. Camino Real F1 seeds (Harris Moran®, Seed Company) were used. The seeds were washed with running water to remove the fungicide coating and then sown in three plastic germination trays with 72 cavities (6×12, one seed per cavity); for this, a sand-peat mixture (Mix No. 3, Sunshine®) was used as substrate at a 1:1 (v/v) ratio and sterilized at 120 °C for 6 h at a pressure of 1.05 kg·cm-2. Each tray was placed inside a base and covered with a transparent plastic dome, then placed in an incubation room at 21 ± 1 °C with a photoperiod of 16/8 h (light / dark). Irrigation was carried out with running water once a week.
Microbiological material
Actinobacteria from the microorganism collection of the Phytopathology Laboratory of the CIATEJ Plant Biotechnology Unit were used. The actinobacteria used were ABV38, ABV39 and ABV45, previously isolated from the rhizosphere of Agave spp. (Rincón-Enríquez, López-Pérez, & Quiñones-Aguilar, 2014). In previous tests, these actinobacteria inhibited the in vitro growth of Phytophthora capsici CH11 (PC CH11) (Reyes-Tena, Fernández-Pavia, Rincón-Enríquez, López-Pérez, & Quiñones-Aguilar, 2014). Actinobacterial isolates were grown in potato-dextrose agar (PDA) culture medium with pH 7 (3 M NaOH) and were incubated at 21 ± 1 °C in the dark for 15 days. The PC CH11 strain was cultured in clarified V8 agar (50 mL·L-1 of V8 juice, 0.5 g·L-1 of CaCO3 and 15 g·L-1 of agar) and incubated at 21 ± 1 °C in the dark for 10 days.
Dual antagonistic activity bioassay
Actinobacteria ABV38, ABV39 and ABV45 were tested to determine their activity in inhibiting in vitro growth of PC CH11 in dual confrontation assays. A disk containing a 6 mm diameter actinobacterial isolate with 15 days of growth was deposited 1 cm from the edge of the Petri dish with PDA culture medium. The Petri dishes were pre-incubated before inoculating the phytopathogenic oomycete for 0, 3 and 5 days at 21 ± 1 °C in the dark (Evangelista-Martinez, 2014). After the pre-incubation time, a 6 mm diameter PC CH11 disk (10 days of growth) was deposited 1 cm from the edge of the Petri dish and opposite the disk with actinobacteria. Dishes inoculated only with PC CH11 at 1 cm from the edge served as the control. Antagonistic activity was determined 24 days after inoculation from the area of PC CH11 growth inhibition. PC CH11 growth in the control dishes was over the entire surface of the culture medium. For the evaluation, a completely randomized experimental design with three replicates was established, where three treatments were evaluated per actinobacterium, corresponding to the pre-incubation times (0, 3 and 5 days).
Production of broth culture supernatant
One disk of each actinobacterium on 6 mm diameter PDA was independently inoculated in 250 mL Erlenmeyer flasks with 125 mL of culture medium (potato-dextrose broth, pH 7) that had been previously sterilized (121 °C at 1.05 kg·cm-2 for 20 min). The flasks were placed in an orbital incubator at 30 °C and 200 rpm for 20 days. Four flasks were inoculated for each actinobacterium. After incubation, the broth culture was centrifuged at 13,000 rpm for 15 min. The broth culture supernatant (BCS) of each actinobacterium (unfiltered) was recovered and stored at 4 °C until use.
Production of Phytophthora capsici CH11 zoospores
Zoospore production was performed using the Ristaino (1990) technique, with slight modifications. PC CH11 cultures with 10 days of growth in clarified V8 agar (50 mL·L-1 of V8 juice, 0.5 g·L-1 of CaCO3 and 15 g·L-1 of agar) were used. The culture medium of each Petri dish was cut in half, and one of the halves was placed in another sterile Petri dish. Using a scalpel, the clarified V8 agar halves were cut into ~1 cm2 squares and sterile distilled water was added to cover the agar squares. The Petri dishes were placed in an incubation room at 21 ± 1 °C in the dark. The distilled water was renewed daily for five days. Finally, the Petri dishes were brought to 4 °C for 1 h to induce zoospore release. The suspension was adjusted to 500 zoospores·mL-1 using a hemocytometer.
Evaluation of biorational control of Phytophthora capsici CH11 in vivo
Three days before applying the treatments, the serrano pepper plants were irrigated with plenty of running water, and excess water from the base container was removed to avoid cross contamination. Ten mL of the BCS of actinobacteria ABV38, ABV39, and ABV45 were applied independently to each 25-day-old pepper plant. As a negative control, 10 mL of sterile distilled water were applied per plant, and the commercial product Infinito® (Bayer®, Mexico), recommended for the control of oomycetes, which contains fluopicolide (62.5 g·L-1) and propamocarb hydrochloride (625 g·L-1), was used as a chemical control. For the chemical control, a suspension was prepared at 3 mL∙L-1 of the concentrated product and 10 mL were added per plant. After applying the treatments, each plant was inoculated with 3 mL of the zoospore suspension (500 zoospores·mL-1) of PC CH11, at a final concentration in the substrate of 50 zoospores·g-1. The control plants were inoculated with 3 mL of sterile distilled water. After inoculation of the phytopathogenic oomycete, the plants were incubated at 21 ± 1 °C with a photoperiod of 16/8 h (light/dark) for 10 days. No further irrigation was applied to the plants.
For this test, a completely randomized experimental design with 10 treatments was used: T1) ABV38, T2) ABV38 + PC CH11, T3) ABV39, T4) ABV39 + PC CH11, T5) ABV45, T6) ABV45 + PC CH11, T7) control (healthy plants), T8) control + PC CH11 (diseased plants), T9) chemical control and T10) chemical control + PC CH11. The treatments were applied in trays with the pepper plants. One tray had 12 columns, each with six pepper plants. The end columns of the tray were excluded. The 10 internal columns were randomized to apply the treatments. The experimental unit consisted of six pepper plants, and the treatments were carried out in triplicate. The survival percentage of the plants was determined with the following formula:
Molecular identification of actinobacterium strains
Actinobacterial isolates ABV38, ABV39 and ABV45 were identified by partial amplification of the 16S rRNA gene by means of polymerase chain reaction (PCR), for which the oligonucleotides fD1 (5’-CCGAATTCGTCGACAACAGAGTTTGATCCTGGCTCAG-3’) and rD1 (5’-CCCGGGATCCAAGCTTAAGGAGGTGATCCAGCC-3’) (Weisburg, Barns, Pelletier, & Lane, 1991) were used. DNA extraction, PCR and sequencing of the amplified fragments were performed at the Biotechnology and Seed Pathology Laboratory of the Colegio de Postgraduados. The amplified fragments were sequenced in both directions using primers 8F, 1492R, U514, 800R, fD1 and rD1 (Edwards, Rogall, Blockerl, Emde, & Bottger, 1989; Stackebrandt & Liesack, 1993).
Consensus sequences of strains ABV38, ABV39 and ABV45 were compared in the GenBank/EMBL/DDBJ database through the NCBI (http://www.ncbi.nlm.nih.gov/) using the BLAST (Basic Local Alignment Search Tool) program (McGinnis & Madden, 2004). Reference sequences and those of phylogenetically-related strains were used with each actinobacterium. The sequences were aligned with CLUSTAL W (Thompson, Higgins, & Gibson, 1994) and the phylogenetic tree construction was performed by means of the Neighbor-Joining (NJ) method (Saitou & Nei, 1987) with 1,000 Bootstrap replicates using the MEGA-X program (Kumar, Stecher, Li, Knyaz, & Tamura, 2018). The nucleotide substitution model selected for NJ was Kimura-2 parameters (Kimura, 1980). The partial 16S rRNA gene sequences of strains ABV38, ABV39 and ABV45 were deposited in the GenBank database under accession numbers MT435538, MT435539 and MT435540, respectively.
Statistical analysis
The response variables (inhibition and survival percentage) were subjected to analysis of variance and Tukey's mean comparison test (P ≤ 0.05), for which the StatGraphics Centurion XV (StatPoint Inc., 2005) statistical package was used. The survival percentage data were transformed with ArcSen√x prior to analysis.
Results
In vitro antagonistic activity
Strains ABV38, ABV39 and ABV45 had different degrees of in vitro growth inhibition of PC CH11 (Figure 1). Pre-incubation (5 days) of strains ABV38, ABV39 and ABV45 showed significant differences (P ≤ 0.05) in PC CH11 growth inhibition (with an increase of 14.9, 10.4 and 9 %, respectively) compared to treatments without pre-incubation (Figure 1). Strain ABV39 showed the lowest reduction (28 %) of PC CH11 mycelial growth at 5 days of pre-incubation, while strains ABV38 and ABV45 had an inhibition percentage of 51 and 50 %, respectively, at the same pre-incubation time (Figure 1 and 2). Results of the in vitro antagonistic activity clearly indicate the potential of strains ABV38 and ABV45 as biological control agents of PC CH11, by presenting significant differences in the inhibition of mycelial growth of this plant pathogen with respect to strain ABV39 at any pre-incubation time (Figure 1 and 2).
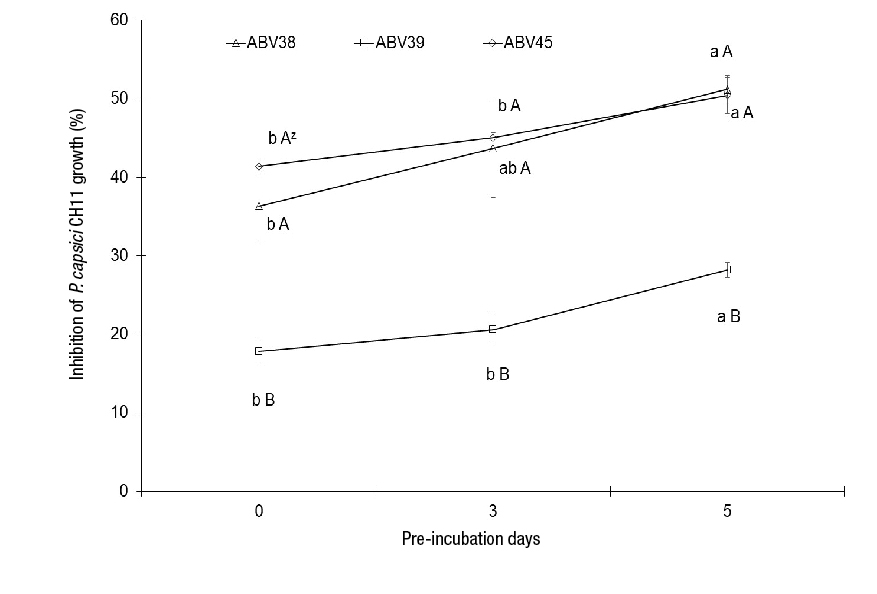
Figure 1 Behavior of the in vitro growth inhibition ability of Phytophthora capsici CH11 by different strains of Streptomyces spp. (mean ± standard deviation). zMeans with the same letter within pre-incubation time (lowercase) or by strains (uppercase) do not differ statistically (Tukey, P ≤ 0.05).
Biorational control of P. capsici CH11 in vivo
Table 1 presents the survival percentage of serrano pepper plants in the different treatments. Results indicate that the BCS of strains ABV45 (T6; 94 %), ABV38 (T2; 77 %) and the chemical control (T10; 100 %), applied to pepper plants inoculated with PC CH11, showed significant differences in the survival percentage compared to the control (T8; 0 %). Plants treated with the BCS of strain ABV39 (T4; 0 %) did not show a control effect of PC CH11.
Table 1 Effect of the application of the broth culture supernatant of actinobacterias on the survival percentage of serrano pepper var. Camino Real plants 10 days after inoculation with Phytophthora capsici CH11.
Treatment | Survival (%) |
---|---|
T1) Streptomyces sp. ABV38 | 100 az |
T2) Streptomyces sp. ABV38 + P. capsici CH11 | 77 b |
T3) Streptomyces sp. ABV39 | 100 a |
T4) Streptomyces sp. ABV39 + P. capsici CH11 | 0 c |
T5) Streptomyces sp. ABV45 | 100 a |
T6) Streptomyces sp. ABV45 + P. capsici CH11 | 94 a |
T7) Control (healhty plants) | 100 a |
T8) Control + P. capsici CH11 (diseased plants) | 0 c |
T9) Chemical control | 100 a |
T10) Chemical control + P. capsici CH11 | 100 a |
zMeans with the same letter do not differ statistically (Tukey, P ≤ 0.05).
The in vivo experiment showed that applying the BCS of strains ABV38 and ABV45 had a suppressive effect on the wilting of pepper plants caused by PC CH11, while in the control treatment inoculated with PC CH11, severe defoliation and wilting was observed 10 days after inoculation with the phytopathogenic oomycete (Figure 3). The application of the BCS of the actinobacteria did not affect the survival of pepper seedlings not inoculated with PC CH11 (Table 1); however, a decrease in root length was observed due to the application of the broth culture (Figure 3).
Molecular identification
Comparative analysis of the 16S rRNA gene sequences of actinobacterial strains ABV38 (1,404 bp), ABV39 (1,403 bp) and ABV45 (1,231 bp) confirmed that all three strains belong to the genus Streptomyces (Figure 4). Analysis of these sequences indicated that strains ABV38, ABV39 and ABV45 are phylogenetically related to S. thioluteus NBRC 13341 (99.36 %), S. galbus DSM 40480 (99.29 %) and S. cinnamoneus NBRC 15926 (99.76 %), respectively. However, further studies are needed to establish whether strains ABV38, ABV39 and ABV45 represent new species for the genus Streptomyces.

Figure 4 Neighbor-Joining phylogenetic tree based on partial 16S rRNA gene sequences showing the phylogenetic relationships between strains ABV38, ABV39 and ABV45 with other species of the genus Streptomyces. The numbers beside the branches are Bootstrap values (%). The sequence of Streptosporangium fragile DSM 43847 was used as an outgroup. The accession number of each sequence is shown in parentheses. Bar = 0.01 substitutions per nucleotide position.
Discussion
The genus Streptomyces is a potential source of biocontrol agents against Phytophthora spp. (Arfaoui et al., 2018; Sadeghi et al., 2017; Thampi & Bhai, 2017). In the present study, the antagonistic activity of three Streptomyces strains against PC was examined under in vitro and in vivo conditions. Under in vitro conditions, strains ABV38 and ABV45 showed the ability to inhibit mycelial growth of PC CH11 in direct confrontation, whereas strain ABV39 exhibited weak activity. Secondary metabolites with antioomycete activity could be involved in mechanisms inhibiting PC mycelial growth.
Chen et al. (2016) report that the fermented broth of S. plicatus B4-7 (cell-free) cultured in oatmeal broth inhibited mycelial growth of P. capsici, P. cinnamomi, P. palmivora, and P. parasitica by 55, 65, 43.8, and 35 %, respectively. Yang, Zhang, and Li (2019) note that metabolites from the ethanolic extract of the fermented broth of S. corchorusii AUH-1 inhibited the mycelial growth of P. capsici and P. parasitica var. nicotianae by 67.2 and 78.9 %, respectively. In this study, Streptomyces sp. ABV38 and ABV45 exhibited inhibition percentages of 51 and 50 %, respectively, on PC mycelial growth. However, further studies are required to determine which metabolite(s) are involved in the inhibition of P. capsici and their mode of action.
During in vivo evaluation of the BCS of strains ABV38 and ABV45, applied to pepper plants, the broth showed a suppressive effect on wilt caused by PC CH11. In studies with Streptomyces spp., Nguyen et al. (2012) showed that the culture supernatant of S. griseus H7602 reduced the wilt of pepper roots inoculated with PC, and in subsequent studies they demonstrated that the antioomycete activity was due to pyrrolidone carboxylic acid (Nguyen et al., 2015). Chen et al. (2016) demonstrated that the fermented broth obtained from the growth of S. plicatus B4-7 completely inhibited in vitro germination of PC zoospores and reduced crown rot disease by 75 % in bell pepper plants inoculated with PC zoospores. In this case, the compound purified from the culture broth with the highest antioomycete activity was borrelidin. Sellem et al. (2017) found that the supernatant from the lyophilized culture of Streptomyces sp. TN258 completely inhibited the germination of P. ultimum oospores at a concentration of 12.5 mg·mL-1.
Regarding secondary metabolites, Lee, Sherman, and Hwang (2008) found that thiobutacin, isolated from L. aerocolonigenes VK-A9, exhibited the lysis of PC zoospores. Islam, Laatsch, and von Tiedemann (2016) observed the zoosporicidal activity of macrotetrolide dinactin isolated from Streptomyces sp., as it halted motility and caused the subsequent lysis of zoospores of both P. capsici and the phytopathogenic oomycete Plasmopara viticola. Abdalla et al. (2011) isolated and purified compounds from the culture broth of Streptomyces sp. ANK313, a new one (khatmiamycin) and others already known, which inhibited motility and caused lysis of P. viticola zoospores.
In this study, the BCS of Streptomyces sp. strains ABV38 and ABV45 suppressed wilt of pepper plants when inoculated with PC CH11 zoospores. In vivo test results suggest that secondary metabolites of BCSs could be involved in controlling the disease by acting directly against zoospores. Further studies are needed to identify which BCS compound(s) are involved in suppressing wilt caused by PC.
On the other hand, the commercial product Infinito®, used as a chemical control, exhibited 100 % control over PC CH11 as the pepper plants showed no evidence of wilt 10 days after inoculation. In in vitro studies, fluopicolide and propamocarb hydrochloride have been shown to have an effect on the reduction of mycelial growth, sporangium formation, zoospore motility and germination at different stages of Phytophthora spp. development (Hu, Hong, Stromberg, & Moorman, 2007; Toquin, Latorse, & Beffa, 2019).
Although the BC of Streptomyces sp. ABV39 did not exhibit a wilt-suppressing effect on PC CH11, in previous studies, co-inoculation of poblano pepper plants with Streptomyces sp. ABV39 spores and arbuscular mycorrhizal fungi reduced wilt severity relative to the PC-inoculated control (Reyes-Tena, Rincón-Enríquez, López-Pérez, & Quiñones-Aguilar, 2017). The results suggest that Streptomyces sp. ABV39 is not useful for biorational control of PC; however, it could be used as a biocontrol agent.
Conclusions
Streptomyces sp. strains ABV38, ABV39 and ABV45 showed different degrees of control over in vitro growth of P. capsici CH11 depending on incubation time. The 5-day pre-incubation of the three strains significantly increased the growth inhibition of P. capsici CH11. In vivo evaluation showed biorational control of the culture supernatant of strains ABV38 and ABV45 in the suppression of wilt caused by P. capsici CH11 in pepper plants under controlled conditions. Molecular identification of strains ABV38, ABV39 and ABV45 indicated that they belong to the genus Streptomyces. Overall, strains ABV38 and ABV45 have potential for biorational control of pepper wilt.