Introduction
Most of the coastal lagoons situated in the tropics and other regions of the world are shallow (Solidoro et al. 2004, Medina-Gómez and Herrera-Silveira 2006, Abreu et al. 2010), with depths no greater than 3 m in their main channels; therefore, the atmosphere, through heat fluxes and air temperature, has an important effect on water temperature patterns inside the lagoons. Lagoons are also affected by river discharges mainly during the rainy season. An example is the Venetian Lagoon (Italy), for which an analysis of the most important water quality parameters confirmed the role of river discharges (which are enhanced during the rainy season) and exchanges promoted by tides with the sea in determining the water quality and the dynamics of the ecosystem (Solidoro et al. 2004). Unlike the Venetian Lagoon, Dzilam Lagoon (Yucatan Peninsula, Mexico) shows a decrease in primary production during the rainy season. The entry of large volumes of fresh water from direct precipitation and groundwater discharge into the lagoon is associated with short residence times of water during this season, causing reduced phytoplankton production despite the significant contribution of nutrients (Medina-Gómez and Herrera-Silveira 2006).
Moreover, variations in chlorophyll a (Chla) levels appear to be most affected by hydrology, which is primarily driven by meteorological factors such as wind, rainfall, and evaporation. However, hydrological driving forces play different roles on different time scales. Thus, meteorological phenomena and variations in river runoff (Elliott and Quintino 2007) can promote interannual, annual, and seasonal fluctuations in sea surface temperature (SST) and Chla concentrations in lagoons. Nevertheless, for the Venetian Lagoon, the temperature fields are rather uniform, even if the effect of the exchanges with the sea can be recognized (Solidoro et al. 2004). Conversely, in the Patos Lagoon estuary in southern Brazil, rainfall and wind direction fluctuations have been observed to control temperature, salinity, water transparency, and Chla concentrations associated with shorter cycles in the spring than in the summer (Fujita and Odebrecht 2007). These effects have also been observed on the El Niño/Southern Oscillation (ENSO) time scale (Abreu et al. 2010).
Materials and methods
Study area
Tamiahua Lagoon is located in the coastal zone of Veracruz State, in the southwestern Gulf of Mexico (Fig. 1). The lagoon is 110 km long, 25 km wide, and has a depth of 1-3 m. It is important because of fishing, shellfish farming, and tourism. The lagoon is connected to the western continental shelf through two inlets (Fig. 1), where the exchange of water is promoted by the diurnal tide of the gulf basin (Salas-Pérez et al. 2008). Additionally, the lagoon receives discharges from many rivers (Fig. 1); however, discharge data do not exist. We assume that there is an increase in river input to the lagoon during the rainy season and hence fluctuations in SST and Chla concentrations related to precipitation and runoff. Moreover, the use of satellite imagery and meteorological data over a period of 10 years allowed us to study interannual signals such as El Niño and La Niña and evaluate whether these phenomena affect meteorological and thus oceanographic parameters within the lagoon. In Veracruz, El Niño/La Niña warm/cold conditions induce extreme rainfall episodes in winter/summer-fall and moderate episodes in summer/winter (Torres-Alavez et al. 2010). Coastal lagoons are under-represented in the literature. For Tamiahua Lagoon, there are no studies on SST and Chla spatial and temporal fluctuations at seasonal, annual, and interannual scales forced by rainfall and heat fluxes, which are necessary to better understand its hydrological features.
Data set
Monthly SST images from the NOAA-16 infrared channel and MODIS Level-3 monthly standard mapped images of Chla were used to elucidate SST and Chla temporal and spatial variability in Tamiahua Lagoon from July 2002 to May 2012, and thereby evaluate the hypothesis that fluctuations of these oceanographic parameters are mainly influenced by precipitation and heat fluxes. The SST images employed in this study were obtained from the Giovanni Ocean Color Radiometry Online Visualization and Analysis node, and the Chla images were obtained from the ERDAPP node. The spatial resolution is 0.0360° x 0.0360° for the SST images and 0.0125° x 0.0125° for the Chla images; in both cases, the resolution is good enough to characterize processes in Tamiahua Lagoon. Satellite images were used in this study instead of in situ measurements in the lagoon, which are not in the public domain and are also under-represented over the study period. The study area is well covered by satellite imagery (98%). Therefore, this study was conducted using 119 monthly images of SST and Chla. The temporal fluctuations of SST and Chla were studied using time series built from the monthly satellite images. The spatial fluctuations in the SST and Chla images were then obtained from the means and standard deviations over the analyzed period, and seasonal averages from July 2002 to May 2012 were computed to obtain the climatologic fields of those parameters in Tamiahua Lagoon and thus describe their main patterns.
In situ atmospheric measurements were not used because there are no meteorological stations in the area of the coastal lagoon. Instead, the effect of air temperature, heat fluxes, and precipitation (river discharge) on the Tamiahua Lagoon waters was analyzed using the NARR/NCEP (North American Regional Reanalysis/National Center for Atmospheric Prediction) data set output model at 1000 mb (Mesinger et al. 2006). The analyzed data were built at a point located near the center of Tamiahua Lagoon (97.5549°N and 21.5506°W) (Fig. 1). It is important to stress that the observations from the NARR/NCEP meteorological model outputs only represent a point in the middle of the lagoon, and we assumed that the heat fluxes, air temperature, and rainfall were uniform throughout the lagoon. The cross-correlations and phase lags between the atmospheric variables (heat fluxes and precipitation), oceanographic parameters (SST and Chla) and normalized precipitation anomaly (SPA = , where SPA represents the seasonal precipitation anomaly, SP are individual values of seasonal precipitation,
is the average of the complete seasonal precipitation time series, and σSP is the standard deviation of the total seasonal precipitation time series), in conjunction with the Oceanic Niño Index (ONI) (Reynolds et al. 2008) were calculated as follows. The cross-correlation was computed with the Matlab crosscorr function. This function was used to analyze the effect of the atmospheric parameters on Chla and precipitation, the SST and Chla time series, and the SST and heat flux time series. The Tamiahua Lagoon heat fluxes (W m-2) were computed with the bulk heat formula:
where Qnet is the net heat flux, SW the shortwave radiation, LW the longwave radiation, LH the latent heat flux, and SH the sensible heat flux.
Results
Temporal fluctuations of SST, air temperature, precipitation, and Chl a concentrations
Seasonal averages of the SST and air temperature time series built from monthly images and NARR/NCEP outputs over the period analyzed in this study showed well-defined annual signals (Fig. 2a). The mean difference between SST and air temperature in Tamiahua Lagoon was approximately 2 °C. Thus, the differences in temperature between both curves were positive due to the heat transferred from the atmosphere to the lagoon throughout the year. Over the 10 years analyzed in this study, the mean SST and air temperature showed marked interannual fluctuations in the cold seasons (differences of approximately 1-3 °C) and in the warm seasons, which presented nearly the same temperature differences (approximately 2-3 °C) as observed in the cold seasons (Fig. 2a).

Figure 2: (a) Interannual time series of sea surface temperature (SST) and air temperature (dashed line). (b) Interannual precipitation (PP) and interannual time series of chlorophyll a (Chla) computed by season. (c) Normalized precipitation anomaly (SPA) vs the Oceanic Niño Index (ONI), in the ENSO warm and cold phases and in the neutral phase.
The seasonal precipitation time series revealed a clear annual signal during the analyzed period (Fig. 2b). The average precipitation was 3.0 ± 2.90 mm month-1. The minimum and maximum values in the period analyzed were ~0.002 mm month-1 (spring of 2011) and ~23 mm month-1 (summer of 2010), respectively. Seasonal precipitation time series displayed maximum values (~5 to 23 mm month-1) in summer and minimum values (approximately 0.008 to 0.43 mm month-1) in winter.
A clear Chla annual signal was observed (Fig. 2b) similarly to the other time series previously described, with maximum peaks between 13 and 40 mg m-3 throughout the summer and fall, in contrast to winter, during which minimum values of approximately 6 to 14 mg m-3 were observed. Spring showed minimum and maximum concentrations of 6 and 22 mg m-3, respectively. However, in general, as precipitation increased, the Chla concentration also increased.
Warmer temperatures, higher rainfall, and increased Chla were generally common features during the summer and fall (Fig. 2b). The cross-correlations between the seasonal precipitation and seasonal Chla time series showed values greater than 0.40 in all seasons (Table 1). The negative phase lags between those time series (Table 1) showed that seasonal precipitation occurred first, followed by the seasonal increase in Chla concentration. In general, high cross-correlations (about 1) were observed in the summer and fall, which agree with the seasonal periods of maximum precipitation in the study area, suggesting the influence of maximum river discharge into Tamiahua Lagoon. However, the cross-correlation values were also higher than 0.6 in winter and spring (Table 1). The cross-correlation analysis showed a P < 0.00001, indicating significant values. In general, the SPA in the last 4 years was highly positive compared with that in the first 6 years, during which the SPA showed low positive normalized precipitation anomalies (Fig. 2b, c). This behavior may have been due to an interannual forcing mechanism, such as ENSO (warm and cold phases of the phenomenon), which qualitatively manifested its effect on the normalized precipitation anomaly time series of the study area (Fig. 2c). First, the SPA decreased when a warm phase (El Niño) occurred from July 2002 to February 2003 (9 months), from July 2004 to February 2005 (8 months), and from September 2006 to January 2007 (5 months). In contrast, a positive SPA occurred for the El Niño period in the resulting interval: from June 2009 to April 2010 (11 months). Meanwhile, during the La Niña phase, the SPA decreased from November 2005 to March 2006 (5 months), whereas from April to November 2007 (6 months) it was mainly positive, with a short period of negative SPA between December 2007 and February 2008. During the last period, February to November 2008 (10 months), and during the periods June 2009 to April 2011 (11 months), August to October 2010 (3 months), and April to November 2011, the SPA was also positive.
Heat fluxes from the NARR/NCEP model
In general, most of the terms of the bulk heat equation (1) computed with the NARR/NCEP model showed a marked annual signal (Fig. 3, Table 2) except LH (Fig. 3c). The LW flux (Fig. 3a), which represents the radiation emitted by the ocean surface minus the LW emitted by the atmosphere in the direction of the ocean, had a mean net gain value of 460.84 ± 25.16 W m-2, and the flux of sunlight into the sea (SW) had a mean value of 248.02 ± 56.29 W m-2 (Fig. 3b). As expected, the LH (the flux of heat carried by evaporated water) and SH (the flux of heat out of the sea due to heat conduction) had mean negative values of 71.91 ± 37.20 and 81.90 ± 48.99 W m-2, respectively (Fig. 3c, d). Tamiahua Lagoon had a net heat gain of 555.05 ± 42.31 W m-2 throughout the year (Fig. 3e). The correlation of each term of the bulk heat equation with the SST time series showed a maximum value for the LW term (0.60-0.91), followed by the SW term (0.38-0.54) (Table 2). The other two fluxes (LH and SH) generally showed negative correlations (Table 2). The low correlations computed for the LH and SH fluxes affected the computations of the correlation values (0.26-0.70) estimated between the total heat fluxes and SST curve (Table 2).
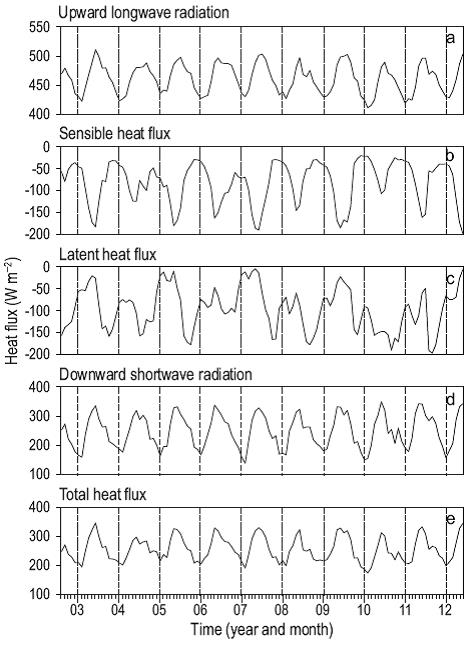
Figure 3: Temporal fluctuations of the time series of heat fluxes, from July 2002 to May 2012: (a) longwave radiation flux, (b) sensible heat flux, (c) latent heat flux, (d) shortwave radiation flux, and (e) net heat flux. Heat fluxes >0 indicate heat gain to the lagoon and <0 indicate heat loss to the atmosphere.
Mean and standard deviation fields of SST
The spatial distributions of the SST field averaged over the study period and their standard deviation are shown in Figure 4(a, b). Mean SST increased from the Boca Corazones Inlet (26 °C) to the mid-western corridor of Ídolo Island (28 °C). From there to the central part of the lagoon, mean SST decreased by approximately 1 °C, whereas at the western boundaries the temperature was higher than 27 °C, likely due to river discharge. A patch of water with a temperature of 28 °C near Juan A. Ramírez Island decreased the temperature of the water extending along the eastern portion of Tamiahua Lagoon to Tampachichi Inlet to 26 °C. The mean SST field temperature computed over the study period was 26.59 ± 3.29 °C. Because of its standard deviation and unlike the mean SST field, there was remarkably high variability in front of the river mouths located to the west of the lagoon. The variability occurred in the southern and northern portions of the lagoon. In contrast, the center of the lagoon retained a homogenous standard deviation.
Mean and standard deviation fields of Chl a
The mean field of the Chla concentration was >11mgm-3, primarily in the southern region where Boca Corazones Inlet is located and in the western corridor of Ídolo Island (Fig. 5a). In front of the Milpas estuarine system, there was a patch of Chla with concentrations >16 mg m-3. Higher values (>16 mg m-3) were found in the western area of the lagoon, in agreement with the river discharge locations (Fig. 1). The eutrophic water with high concentrations of Chla located in the southern area of the lagoon was spread around the eastern side of the lagoon due to local circulation. Moreover, the maximum values of the mean field were found in the corridor along Cabo Rojo and Juan A. Ramírez Island (eastern side of the lagoon). The values increased from 16 mg m-3 in the southwestern region of the Cabo Rojo sand barrier to more than 20 mg m-3 at Tampachichi Inlet. From both sides of Tamiahua Lagoon toward the center, the concentration of Chla decreased, with minimum concentrations reaching <11 mg m-3. In general, the variability of Chla was homogenous in the center of the lagoon, with values <2 mg m-3. The variability of the Chla field showed high values (which increased from 6 to 10 mg m-3) from Tampachichi Inlet toward the southeastern corridor (Juan A. Ramírez Island) and toward the western corridor formed by the island. The southern portion of the lagoon, the corridor formed by the western boundary of the area, and Ídolo Island showed high variability (>11 mg m-3), which was similar to that observed in the northern part of the lagoon (Fig. 5b).
Temporal climatology of SST by season
The SST seasonal time series built from the monthly images showed a strong annual pattern with a Gaussian shape during the year. The maximum temperature was found during summer (30 ± 0.15 °C) and the minimum (23 ± 0.46 °C) in winter. A slightly higher value of SST was determined during fall (26 ± 0.44 °C) than that observed in winter. During spring, we observed an SST value of 2 °C (28 ± 0.41 °C) that was lower than that observed in summer.
Spatial climatology of SST by season
In winter (Fig. 4c), SST was less than 20 °C in the south-southwestern corridor of Ídolo Island and around Juan A. Ramírez Island; this water entered the lagoon through the Boca Corazones and Tampachichi inlets because of the exchange of water caused by the diurnal tides. Parts of the coastline in the western area, where the Laja and Saladero rivers are located, had cooler waters, similar to those that penetrated through Boca Corazones and spread to the northwestern area of Tamiahua Lagoon. Outside this area, in the central part of the lagoon, water temperature increased from 20 to 22 °C. In the north, surrounding the eastern portion of Juan A. Ramírez Island and Tampachichi Inlet, the water temperature was warmer than the water in Boca Corazones Inlet.
During spring (Fig. 4d), the spatial distribution of SST was almost similar to that in winter. In spring and winter, three water types were identified in three areas of the lagoon. The first, with SST ranging from 15 to 20 °C, was observed from Boca Corazones to the southwestern corridor of Ídolo Island; this area showed a significant increase in water temperature in spring (~5 °C) relative to the winter values. The second, with SST above 25 °C, was observed from the southern boundary of Ídolo Island to the northern part of Juan A. Ramírez Island, in the central portion of the lagoon. The third water type spread from the western part of the lagoon; it had a patch of water near the mouth of the Laja and Saladero rivers, with temperatures of 20 to 25 °C, similar to those observed in winter. To the north, near Juan A. Ramírez Island and Tampachichi Inlet, the water temperature was 25 °C, decreasing to 15 °C in the southern portion of Juan A. Ramirez Island; this water was warmer than that observed during winter.
In summer (Fig. 4e), the SST values in the lagoon increased by 10 °C relative to the winter values and by 3 °C relative to the spring values (Fig. 4c, d). In addition, in the western part of the lagoon, the water discharged by Arroyo Carbajal (SST of approximately 29 to 30 °C) extended to the central portion of the lagoon and almost to the western flank of Toro Island (Fig. 1). The water discharged by the Saladero River and the Milpas estuarine system affected the distribution of SST along the western corridor between Boca Corazones and the northern portion of Ídolo Island, where the SST fluctuated from 28 to 29 °C (Fig. 4e).
In fall (Fig. 4f), the spatial distribution of SST was similar to that of summer. In the region close to Boca Corazones and the southern area of Ídolo Island, SST was below 25 °C. Consequently, along the corridor structured by the western boundary of the lagoon, SST was 25 °C; this water spread to the central portion and southern part of Juan A. Ramírez Island. In front of the Arroyo Carbajal discharge, there was a patch of water with a temperature of 25 °C; this water penetrated into the central part of the lagoon near the western flank of Toro Island. At the Cabo Rojo sand barrier, it had a homogeneous temperature below 28 °C. To the north, where the western corridor is structured by Juan A. Ramírez Island and the land, SST varied from 23 to 25 °C. In the eastern corridor located in front of Tampachichi Inlet, SST was approximately 28 °C and surrounded a patch of water with a temperature of approximately 24 °C.
Temporal climatology of Chl a levels by season
The monthly time series of Chla levels for the time interval analyzed showed a mean value of 18.58 ± 2.59 mg m-3 during summer. During winter and spring, the mean Chla values were similar throughout the entire lagoon: 9.48 ± 1.03 and 9.58 ± 1.34 mg m-3, respectively. In the fall, the mean concentration was 17.10 ± 2.02 mg m-3, which was higher than that observed in winter and spring. In general, the temporal fluctuations of the Chla climatology showed the same annual signal observed for SST.
Spatial climatology of Chl a levels by season
During winter, the spatial distribution of Chla levels (Fig. 5c) between Boca Corazones and the southwestern area of Ídolo Island varied from 6 to 10 mg m-3. In the northern area of Ídolo Island, the Chla values fluctuated from 9 to 11 mg m-3. Around Toro Island, there was a patch of water with a Chla concentration of 8 mg m-3. In the northern part of both corridors structured by Juan A. Ramírez Island, the Chla concentration tended to increase from 9 to 12 mg m-3 at Tampachichi Inlet. The average value for the spatial field of Chla during winter was 9.47 ± 1.03 mg m-3.
During spring (Fig. 5d), the average concentration of the Chla spatial field was 9.58 ± 1.33 mg m-3. This value was approximately equal to that computed for winter. From Boca Corazones to Ídolo Island the average Chla concentrations were less than 5 mg m-3. At the northern end of Ídolo Island and in the area of the Saladero River, the concentrations of Chla fluctuated from 10 to 14 mg m-3. At Toro Island and in the central part of the lagoon, there was a patch of water with lower concentrations (<8 mg m-3), similar to that observed in the winter field. In contrast, along the western boundary of the lagoon where the rivers discharge, higher levels were observed (>9 mg m-3). At Tampachichi Inlet, the concentrations of Chla varied from 10 to 11 mg m-3.
During summer (Fig. 5e), there was an increase in the concentrations of Chla relative to those computed for winter and spring. The mean Chla concentration was 18.58 ± 2.59 mg m-3. Thus, summer had the maximum Chla values of all the seasons. Along the western boundary of the lagoon, a higher concentration was observed (8 to 20 mg m-3) due to water flowing from the Boca Corazones Inlet to the northwestern side of the lagoon and the northeastern portion of Ídolo Island. This water surrounded waters from the center of the lagoon, where concentrations were approximately 12 to 14 mg m-3, decreasing (<12 mg m-3) around Toro Island. In the area around Tampachichi Inlet and the eastern end of Juan A. Ramírez Island, the Chla concentrations are >25 mg m-3.
During the fall (Fig. 5f), the Chla values ranged from 13 to 16 mg m-3 near the mouth of the Milpas estuary. On the western boundary of the lagoon, north of Saladero River and north of Ídolo Island, there were patches of water with a Chla concentration of 13 mg m-3. Surrounding Toro Island, there was a patch of water with a concentration of 10 mg m-3, which was surrounded by a water mass that showed a homogeneous concentration of 12 mg m-3. In the area west of Juan A. Ramírez Island and near the mouth of the Cucharas River, there was a patch of Chla with a concentration higher than 13 mg m-3. Between Tampachichi Inlet and the eastern corridor of Juan A. Ramírez Island, the Chla values were higher than 25 mg m-3, which were similar to the Chla concentrations found during summer. The average value for the spatial field of Chla for this season was 17.10 ±2.01 mg m-3.
Discussion
Satellite images of SST and Chla collected from July 2002 to May 2012 in Tamiahua Lagoon, Mexico's third largest tropical coastal lagoon located in the western Gulf of Mexico, were used to explain their temporal and spatial fluctuations. The maximum value of the Chla curve during the summer of 2005 (Fig. 2b) could be related to episodes of heavy rainfall in the western Gulf of Mexico caused by atmospheric phenomena, including cold fronts, tropical storms and waves, and hurricanes in the study area (Tejeda-Martínez and Welsh-Rodríguez 2006). Like other coastal lagoons around the world, Tamiahua Lagoon is eutrophic (Chla > 5 mg m-3) because it receives nutrient loads from the rivers located in its western part (Nixon 1995); however, eutrophic conditions can also be caused by internal recycling processes between the sediment and water column circulation due to the shallowness of lagoons (Nixon 1995).
Contreras and Castañeda (2004) measured (in situ) average Chla concentrations around Tamiahua Lagoon ranging from 0.01 to 20.7 mg m-3, and they recorded the lowest Chla values (<3 mg m-3) in winter and the highest (>15 mg m-3) in summer. The average Chla value computed from the time series constructed with satellite images was 18.58 ± 2.59 mg m-3, which is a lower Chla value than the average in-situ concentrations registered around Tamiahua Lagoon. Also, Contreras and Castañeda (2004) reported a maximum Chla value of 64.1 mg m-3 and in the present study the maximum value was 40 mg m-3. Thus, the Chla values derived from satellite images fall in the range of the in-situ observations reported by Contreras and Castañeda (2004). On the other hand, Ramírez-León et al. (2015) mentioned that the Chla values of shallow waters measured with satellite sensors tend to increase due to high concentrations of organic matter, detritus, or dissolved organic matter, but at least in the present study, the Chla values measured derived from satellite images and in-situ observations agree.
According to Trenberth's (1997) definition, warm (El Niño) and cold (La Niña) phases occur when SST anomalies are greater than ±0.4 °C and have an interval of more than six months. The quantitative cross-correlation was computed between the SPA and ONI (Reynolds et al. 2008) during the periods that fit the ENSO definition, to establish a quantitative remote correlation between the ENSO phases and the rainfall variability in the study area and thereby establish the interannual effects of rain (river runoff) on fluctuations in Chla concentration. For the warm phase (El Niño) (Fig. 2c), only one interval fit the ENSO definition and had a cross-correlation value of 0.60 (3 lag). For the ENSO cold phase (Fig. 2c), two periods fit Trenberth's (1997) definition and had cross-correlation values of 0.86 (-1 lag) and 0.68 (-1 lag). The analysis demonstrated the effect of the ENSO signal on the two phases, which affected the interannual variability of precipitation in the study area and thus of river runoff during the study period.
Concentrations of Chla and SST values can vary in coastal lagoons interannually. For example, in the Patos Lagoon estuary (southeast Brazil), where the concentration of Chla was studied over 25 years (1994-2008), the concentration ranged from 0.30 to 76.50 mg m-3 and the SST fluctuated from 8 to 30 °C with a mean of 19.5 °C (Fujita and Odebrecht 2007), which is 7 °C less than the mean temperature computed for Tamiahua Lagoon. The fluctuations of both parameters in Patos Lagoon were caused by precipitation in 1998 and 2002, when the area was strongly affected by El Niño. River runoff resulted in a high nutrient supply and a corresponding increase in Chla levels. In Tamiahua Lagoon, the quantitative correlation between seasonal precipitation and seasonal Chla concentration increased in the summer and fall seasons. In general, the cross-correlations were greater than 0.7, primarily with a phase lag of 0 (Table 1). In the Patos Lagoon estuary, during strong El Niño years precipitation was extremely high (1,500 mm month-1) and river discharges introduced a large amount of sediment and increased turbidity; therefore, primary biomass decreased. According to the ONI (Reynolds et al. 2008), in Tamiahua Lagoon the SPA is negative during El Niño periods and generally positive during La Niña. Thus, according to the qualitative correlation between SPA fluctuation and the ONI (Fig. 2c), the summer precipitation peaks in 2007 and 2011 developed during the ENSO cold phase and caused an increase in rainfall in the study area (Torres-Alavez et al. 2010); however, it has been well documented by Torres-Alavez et al. (2010) that other atmospheric phenomena also caused an increase in rainfall in the study area, particularly in 2010. Hence, the La Niña signal had an effect on the increase in precipitation in Tamiahua Lagoon and, thus, on river runoff, which affected Chla concentrations. In other lagoons of the world, the La Niña phenomenon tends to decrease rainfall, river discharges, and nutrient inputs and increase evaporation, resulting in decreased SST and Chla levels (Abreu et al. 2010, Coutinho-Hennemann and Mello-Pretucio 2011). Moreover, the cross-correlation between SST and Chla values was generally higher in summer and fall than in winter and spring (Table 3). Thus, the warmer river waters transport waters with high Chla concentrations to the lagoon (Kjerfve 1994).
Table 3: Cross-correlation between sea surface temperature and chlorophyll a time series at zero lag, from July 2002 to May 2012 at Tamiahua Lagoon. The significance levels of the computations were P < 0.0001.

The SST and Chla levels of the Tamiahua Lagoon waters showed strong variability near the Boca Corazones and Tampachichi inlets. This variability can be explained by the diurnal exchange of Gulf of Mexico waters with Tamiahua Lagoon (Salas-Pérez et al. 2008). Moreover, Tamiahua Lagoon has been described as a constant reservoir containing estuarine-type warm water that is temporarily affected by discharges of continental water and continental shelf water entering through the Boca Corazones and Tampachichi inlets. These water types that were introduced into the lagoon by diurnal tide forcing and river discharges in conjunction generated three water types previously mentioned in the results section. In the Venetian Lagoon (Italy), the temporal evolution of the spatial distribution revealed the role of river discharges and tide exchanges with the sea to determine water quality parameters and ecosystem dynamics (Solidoro et al. 2004). The LW heat flux showed the largest correlation values (between 0.60 and 0.91) among all terms of the net flux, implying that it was most important in the modulation of the SST fields of Tamiahua Lagoon. Although this was the poorest heat flux quantified from the reanalysis output models, over sea surface, the differences in LW were substantially smaller than those over land sites, likely because oceans are spatially homogeneous (Wang and Dickinson 2013). Nonetheless, these results explain the high temperatures (about 27 °C) observed throughout the year within Tamiahua Lagoon.