The tomato (Solanum lycopersicum) is considered one of the most important vegetables in many countries in the world (González et al., 2012) due to its wide consumption, area planted, the economic value of its production, the importance of incomes it provides for small -and middle- scale farmers (Burbano and Vallejo, 2017) and due to its high nutritional contribution, since it is a source of antioxidant substances such as lycopene, beta-carotene, flavonoids and vitamins (Rodríguez-Cabello et al., 2020). The most representative of the varieties planted are chonto, cherry and milano (Miranda et al., 2009).
The crop is implemented in the greenhouse or in the open, in ventilated soils, with loamy textures and high contents of organic matters. In addition, this is a warm -and moderate- weather crop, susceptible to frost and low temperatures, therefore it grows in temperatures between 21 to 24 °C (Florido and Álvarez, 2015), which enhances the normal development of the biochemical processes, vegetative growth and fruition. Currently, the quality, yield and production of this crop is affected by numerous phytosanitary problems, some of which are produced by diverse microorganisms, leading to important economic losses (Henao-Henao et al., 2018). Worth highlighting among these microorganisms is the fungus Fusarium oxysporum f. sp. lycopersici (Fol), a frequent inhabitant of the soil, which enters the plant via its roots. The production of proteins, mycotoxins and other components inactivate the defenses of the hosts, allowing the fungal mycelia to accumulate in the xylem, preventing water and nutrients to flow to the leaves and fruits, causing the disease known as vascular wilt. This is one of the most harmful diseases for this crop, due to the easy spread of the phytopathogen, the resistance to adverse conditions and the high cost of the control measures used for their management (Eraso et al., 2014).
Different models have been implemented worldwide for the control of infections by fungi in crops, such as the use of systemic fungicides and diverse agrochemicals, which, in addition to their high cost, are not completely effective or may even lose their effectiveness, leading to multi-resistant microorganisms (Sood et al., 2020), which, in turn, affect both human health and the quality of the environment. Some commonly used fungicides are benzimidazoles and these compounds have been proven to cause mutagenesis of different kinds in plants that come in contact with them (Villa-Martínez et al., 2014). For these reasons, it is currently necessary to reduce the use of fungicides for the control of fungal diseases, and biological control is considered as a healthy, efficient and cheap alternative to solve this problem, with favorable results, not only in the treatment of the disease, but also in the development of the plant.
In recent years, products have been created for the care of agricultural produce, including the use of the genus Trichoderma spp., which is a widely studied antagonist for disease control due to the ease with which it is isolated, cultivated, and to its quick growth in a number of substrates (Ronnie-Gakegne and Martínez-Coca, 2018). This fungus, included in the biological control agents of microbial origin (ACB-M), has the ability to suppress the development of pathogens in plants, colonize its internal tissues and stimulate its growth (Córdova-Albores et al., 2021). Trichoderma spp. can be used as a bio-controller against Fol, due to its chemotaxis, antibiosis and mycoparasitic capacity (Cubillos et al., 2011). The activation of these mechanisms implies the production of metabolites and specific compounds such as hydrolytic enzymes, siderophores, antibiotics, plant growth factors, and others (Poveda et al., 2020).
Vargas-Hoyos and Gilchrist-Ramelli (2015) and Herrera-Téllez et al. (2019) have experimentally determined, both in vivo and in vitro, that Trichoderma spp. displays an evident antagonistic effect against Fusarium spp., the species T. harzianum, T. viride and T. asperellum being the most effective in their antifungal activity. The reduction of the presence of the pathogen, and therefore the symptoms of wilting in tomato plants, is due to the production of different hydrolytic enzymes and secondary metabolites, providing the ability of inhibition and cell degradation. Likewise, they observed that these species are able to stimulate plant growth, including absolute growth rate, and to induce the systemic resistance in plants (Vargas-Hoyos y Gilchrist-Ramelli, 2015; Herrera-Téllez et al., 2019).
One of the main problems with carrying out an adequate management of this disease in tomato crops is the scarce application of information related to the plant-pathogen-antagonist interaction that helps clarify the machinery of resistance of the plant, the mechanisms used by the fungus to evade this response and how the antagonist may modulate those mechanisms in plants and pathogens, as factors to take into consideration when designing efficient control strategies against this disease (González et al., 2012).
According to the above, this article has the aim of carrying out a revision on the genic and metabolic interactions between the tomato plant (Solanum lycopersicum), Fusarium oxysporum f. sp. lycopersici and Trichoderma spp., which give rise to an antagonistic effect, which is used as an alternative for the prevention, control and treatment of vascular wilt. This information essentially contributes to understanding the pattern of virulence of Fol and helps in the development of molecular markers for the strategies to manage the disease.
Process of infection and reactions involved
Fol is a pathogenic fungus, commonly found in the soil and it causes wilting in over 150 hosts. Its ability of survival helps it overcome harsh conditions, due to its resistance structures, known as chlamydospores, with the ability to live indefinitely, even without the presence of a host; but once they come in contact with it, the vascular infection progresses (Srinivas et al., 2019). The plant-pathogen interaction includes the secretion of proteins, metabolites and specific virulence factors, which can be identified with genomics, metabolomics and proteomics (Manikandan et al., 2018; Padilla-Ramos et al., 2021). These studies have provided new knowledge on the molecular crosstalk produced between Fol and its host (González-López et al., 2021).
The life cycle of the Fusarium spp. species is divided into the latent, parasitic and saprophytic stages (Villa-Martínez et al., 2014). In the latent phase, due to the microbiostasis, the growth of the mycelia, chlamydospores and conidia are inhibited, until they come in contact with the exudates, which serve as sources of carbon, nitrogen and organic acids, recognizing specific signals and stimulating their germination. Later, in the parasitic phase, the hyphae of the fungus attach to the roots of the plant, enter them, and once inside, the mycelium moves through the endodermis until it reaches the vascular system of the plant (Cruz et al., 2012) or xylem, the tissue in which the water and nutrients flow, and that is where the mitochondria are produced, which germinate into new hyphae. During this phase, effectors such as enzymes and small secreted cysteine-rich proteins are identified, which make the parasitic lifestyle of Fol possible and act by suppressing the defenses of the host or interfering in the recognition by the host. Then, the fungus uses the vascular system of the plant to spread quickly in an upward direction inside the cell apoplast and finally produce virulence factors, causing the collapse of the tissue when the absorption and the translocation of nutrients in the plant stop (Okungbowa and Shittu, 2012; González et al., 2012).
The initial symptoms presented by the infected plants include a pale color in the leaves, a premature loss of leaves, a delayed growth and a dark brown color in the xylem of the plant, a characteristic sign of the plant. The wilting of leaves, the rotting of the root, and finally death, are a clear sign of the disease in its most advanced stage. Likewise, the fungus may spread to the surface and the outside of the plant when its host dies, returning to the soil and sporulating abundantly to continue its pathogenesis in adjacent plants, or remaining in a latent state until it finds a new stimulus (Cardona-Piedrahita and Castaño-Zapata, 2019).
The development of the disease at a molecular level begins once the Fol identifies the host, and that is when two ways of signaling, known as MAPK and HOG, (response of high-osmolarity glycerol), are activated by the genes Fmk1, Mpk1 and Hog1, respectively. Each pathway has a specific role during the infection. Gene Fmk1 has functions related to the virulence and the fusion of hyphae. Mpk1 is related to characteristics of the cell wall such as its integrity and remodeling, and the growth and fusion of vegetative hyphae. In turn, Hog1 is related to the stress and osmoregulation responses (García-Enciso et al., 2017; Sharma and Marques, 2018). Other genes needed for the disease to develop are Fgb1, Fga1, Fga2, Fpd1 y FOW2. The first two codify subunits β and α of protein G, respectively, controlling the growth, development and virulence of the hyphae, as well as their morphogenesis and conidiation. Fga2 and Fpd1 play a crucial part in pathogenicity (García-Enciso et al., 2017), and FOW2 is important in the initial invasion of the root tissue, in the invasive growth and colonization of plant tissues by this pathogen (Hu et al., 2015).
Aside from the factors mentioned, Fol has a series of secreted proteins in the xylem (Six), which are important for the colonization of the vascular tissue and the development of symptoms of wilting. They are codified by avirulence genes (Avr) in the fungus, the expression of which is regulated by the SGE1 transcription factor. The Avr genes are identified by receptor genes of the tomato plant (R genes), also known as immunity genes (I genes) (Gawehns et al., 2015). Likewise, the presence of the Avr and R genes reflects what was established in the hypothesis called “gene for gene”, which emphasizes that for every gene involved in the resistance of the host, there is a corresponding gene in the pathogen with which it interacts, that is, the interaction and compatibility between Avr and R genes will result in the success or failure of the infection in tomato plants (Thrall et al., 2016).
Six1, codified by gene Avr3, requires the presence of living plant cells and its secretion is carried out immediately after the penetration of the root cortex (García-Enciso et al., 2017). It accumulates in the sap of the xylem, like Six3, Six5 and Six6, and jointly, Six1, Six3 and Six4, along with their corresponding Avr genes, activate the resistance of the host, mediated by the I genes of the tomato plant. In this way, Six4 (Avr1), Six3 (Avr2) and Six1 (Avr3) are recognized by I genes e I-1, I-2 e I-3 of the plant, respectively (Petit-Houdenot and Fudal, 2017; Carmona et al., 2020).
Fol is also able to secrete other enzymes that increase its virulence and intervene in its pathogenicity. Polygalacturonases, pectinases, xylanases and proteases are important in the degradation of the plant cell wall, like tomatinase, codified by gene TOM1, responsible for inhibiting the plant defense response, allowing the infection to develop (De Sain and Rep, 2015). Regarding toxins, fumonisin, fusaric acid, trichothecenes A and B, enniatins, fusaproliferin and moniliformines are one of the most relevant tones produced by Fol, and they have the ability to break the permeability of the cell membrane in the plant and block their ability to control the loss of water and nutrients. They also suppress the absorption of mitochondrial oxygen and are able to conjugate with elements such as Cu, Zn, Co and Fe, forming complexes that make these minerals unavailable for plants (Figure 1) (Li et al., 2013).
Immune response of the plant and capacity of resistance
Biologically, the most superficial defense barrier of the plant is cutin, which protects it from microorganisms and other factors in the environment; however, when the plant is infected internally by a pathogen such as Fusarium spp., the pathogen quickly penetrates the intercellular spaces and, upon being recognized as a foreign agent, calose, lignin and suberin thicken the cell wall so it prevents the mycelium from spreading. Biochemically, toxins are synthesized and a large variety of antimicrobial compounds are released, such as glycosidics, phenolic, sulphurated compounds, unsaturated lactones, and others. Finally, the well-known necrotic aspect of the disease is the product of a hypersensitive response, in which cell death is programmed around the source of infection due to a metabolic and transcriptional change that allows phytoalexins to accumulate in the stem and achieves a later acquired systemic resistance in case the same or another infection agent with a similar pathogenesis presents itself (De Miguel-Rojas, 2014).

Figure 1 Genomic and proteomic activation and expression of Fol. A) Activation of specific signaling pathways, B) Expression of avirulence genes, C-D) Secretion of enzymes and toxins that promote virulence (Source: Authors).
To face the effects of Fol, the tomato plant carries out two basic responses. In the first place, the detection and identification of many, pathogenic and non-pathogenic microorganisms become possible and, in addition, at a cellular level, calcium mobilizes towards cytosol to produce chinase proteins, reactive oxygen species (ROS) are generated and immunity genes (R genes) are activated, thanks to Pathogen Associated Molecular Patterns (PAMP). Secondly, effector-triggered immunity takes place, which responds to the presence of pathogens and virulence factors, developing diverse mechanisms, such as the production of tyloses and the retention of H2O2, which act as barriers to avoid infections from progressing (García-Enciso et al., 2017; Andrade-Hoyos et al., 2015). This second type of immunity, also known as the acquired systemic resistance, is mediated by the hormonal signaling of salicylic acid (SA) (Aamir et al., 2018).
As a part of the PAMPs activated in the disease by wilting, molecules such as membrane-related chitin, glucans (particularly β-1,6-glucan) and some glycoproteins, codified by gene Fem1 are considered. Each one of these elements can be recognized by the defense system of the plant, since it has pattern recognition receptors (PRR) (Figure 2). The chitin synthesis is codified by the gene chsV, and its expression depends on the Fmk1-MAPK signaling pathway, its union to receptors is direct and it can allow the induction of defense genes against pathogenic fungi. On the other hand, the presence of glucans in tomato has not been widely studied, but it is known to be useful to detect fragments of the pathogen that may be causing harm (García-Enciso et al., 2017).
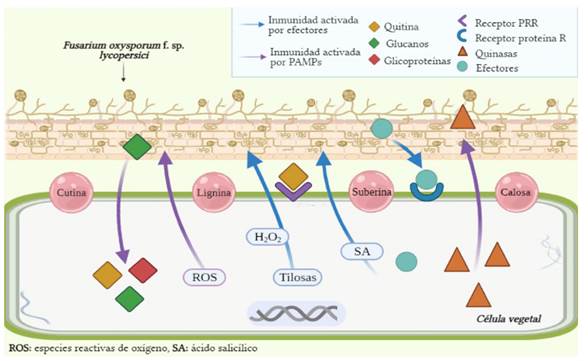
Figure 2 Genic and metabolic interaction between the tomato plant and Fusarium oxysporum f. sp. lycopersici (Source: Authors).
Regarding the resistance genetics of the host, the I genes, located in chromosomes 11 and 7 of the plant, are essential, depending on the actions of the different races of Fol; thus, the I and I-1 genes provide race 1 with resistance, gene I-2 controls resistance against races 1 and 2 and gene I-3 controls resistance against race 3 of Fol. In tomato, the partial resistance to Fol is also mediated by the Frl gene, located in the long arm of chromosome 9 (Gonzalez-Cendales et al., 2015; Pérez-Almeida et al., 2016).
On the other hand, the capacity of transcription factors has also been investigated, as in the case of WRKY proteins, which, in tomato, make up a total of 83 genes. These function as positive or negative regulators (Bai et al., 2018) and recognize a non-codifying DNA sequence known as W-box (TTGACC/T) which regulates expression directly or indirectly and activates or inactivates other genes, being able to control biological processes such as plant growth and their response to biotic and abiotic stress (Aamir et al., 2019; Mohanta et al., 2016).
Relevant aspects in the Trichoderma spp. - Fusarium oxysporum f. sp. lycopersici interaction
Fungi of the genus Trichoderma spp. are distributed ubiquitously around the world and include different species with the ability to colonize the roots of a wide variety of plants, as well as to antagonize and eliminate other fungi (Domínguez et al., 2016). These antagonistic properties are based on action mechanisms that control the development of phytopathogens. Particularly, competition for space and nutrients, mycoparasitism, antibiosis and the production of secondary metabolites, enzymes, volatile and non-volatile compounds, and others (Hernández-Melchor et al., 2019; Martínez et al., 2013).
During the infection process, Trichoderma spp. activates these mechanisms in order to regulate the development of Fol. The competition for nutrients and space is a very important antagonistic mechanism. This takes place only when resources such as soil nutrients and space are limited (Sood et al., 2020). Trichoderma spp. produces secondary metabolites able to inhibit or reduce growth and other activities in Fol, giving it ecological advantages over its competitors, since it uses the resources available in the soil for its growth, leaving the phytopathogen with insufficient nutrients to grow. The competition for nitrogen, carbon and iron has been proven to be a mechanism related to biological control or the suppression of wilting by Fusarium spp. (Sánchez-Espinosa et al., 2020). The low concentrations of these nutrients lead to a reduced germination of conidia, a slower growth of the germinative tube of Fol and a reduced number of infection sites, making it inefficient to produce diseases (Vos et al., 2015; Nusaibah and Musa, 2019).
On the other hand, mycoparasitism is a direct biological control mechanism in which Trichoderma spp. acts by detecting and parasitizing phytopathogens (Nusaibah and Musa, 2019). In this mechanism, Trichoderma spp. grows chemotropically towards Fol and sticks to its hyphae through carbohydrates, which attach to the lectins of the pathogen. This adhesion takes place alongside the formation of structures known as appresoria, which wrap around the hyphae of the pathogen (Sharma and Sharma, 2020). Once it recognizes and sticks to it, the Trichoderma spp. mycelium wraps around the mycelium of the phytopathogen and secretes cell wall degrading enzymes (CWDE), such as chitinases, glucanases (β-1,3-glucanase, β-1,6-glucanase), cellulases, laccases and proteases (Silva et al., 2019). Microbial strains that synthesize such enzymes can lead to the formation of exoelicitors (inducers of resistance of the cell wall) and endoelicitors (inducers of resistance of the cell wall of the plant) (Jaroszuk-Ściseł et al., 2019). In addition, heterotrimeric G proteins are crucial for intracellular signalling and they participate in the perception of signals from the host, thus activating the mycoparasitic attack in Trichoderma spp. (Alfiky and Weisskopf, 2021).
Antibiosis is a strategy used by Trichoderma spp. for its defense and to inhibit the growth of phytopathogens. This fungus can secrete antibiotics such as terpenes, trichodermine, trichozianine, gliovirine, gliotoxin, viridine, pyrones and peptaibols (Khan et al., 2020; Mukherjee et al., 2012). Other metabolites include tricholine, harcyanic acid, heptelidic acid, 6-pentyl-α-pyrone, terpenoids, polyketides and sesquiterpenes, which penetrate the mycelium of the pathogen and act synergically in the cell wall degradation, digesting its intracellular content (Sharma and Sharma, 2020; Wu et al., 2017; Matarese et al., 2012; Ojha y Chatterjee, 2011). The secondary metabolites and antibiotics are the most important chemical factors produced by Trichoderma spp. to eliminate phytopathogenic fungi. It is therefore important to point out that, the more metabolites are produced, the more antagonistic action there will be. This turns Trichoderma spp. in the most widely used antagonist as a biological control agent (BCA) (Sonkar, 2019).
Role of Trichoderma spp. in the biocontrol of wilting
Some species of Trichoderma spp. interact in the benefit of the tomato plant (Solanum lycopersicum), favoring its development and activating its defense system against diverse phytopathogens. Each interaction is mediated by metabolites and genes expressed differentially and it is possible to deduce that the proteins codified by them, which are also known as effectors, help establish these relations (Ramirez-Valdespino et al., 2019).
The effector molecules have been studied in detail, since they play an important part in harmful interactions and in symbiotic interactions. As a part of an action of mechanism, Trichoderma spp. expresses effectors involved in the interaction with plants, inducing their defense system and promoting their growth (Guzmán-Guzmán et al., 2017). One of the most abundant group of effectors in this fungus correspond to small secreted cysteine-rich proteins (SSCP), which group into different protein families, such as: a) cerato-platanins, which are able to induce defense responses in the plant; b) thioredoxin proteins, identified as possible effectors during mycoparasitic interactions; c) hydrophobins, which mediate the interaction between the fungus and hydrophobic surfaces, promoting the growth and creation of defenses in plants; d) glycoside-hydrolases, which participate in the induction of genes involved in the defense and unleash the biosynthesis of ethylene and the hypersensitive response; and e) proteins with CFEM (Commonly Found in Extracellular Membranes) domains, described as cell receptors and signal transductors in host-pathogen interaction signals (Figure 3) (Ramírez-Valdespino et al., 2019; Kubicek et al., 2019; Guzmán-Guzmán et al., 2017).

Figure 3 Action of Trichoderma spp. as a biocontroller of wilting (Fusarium oxysporum f. sp. lycopersici) in the tomato crop (Source: Authors).
Nevertheless, the genetic biocontrol mechanism induced by Trichoderma spp. is mediated by the transcriptional regulation of genes inducible by stress and by the activation of an adaptive systemic response, which is modulated through an immediate expression of the WRKY genes in the plant. This response consists of a complex cascade that may involve phytohormones or auxins, abscisic acid (ABA), cytokinins (CK) and brassinosteroids to for signaling or cross-communication pathways, such as the one induced by jasmonic acid and ethylene (JA/ET) or the one dependent on salicylic acid (SA). Thus, transcription factor WRKY acts as a convergence between the SA (resistance activated by the PAMPs) and JA signaling pathways (resistance activated by WRKY proteins contained in both Trichoderma spp. and the plant) (Aamir et al., 2019). All these processes jointly lead to a facultative symbiosis between the pathogen’s host plant and Trichoderma spp., where these reciprocal advantages take place (Manganiello et al., 2018).
Both the processes that make the infection of tomato possible and those which activate the response to the attack of the pathogen and those which unleash the regulating cascade of the antagonist may display a broad and complex molecular activity, and since all interactions carried out require a series of simultaneous stimuli and reactions, it is complicated to understand the relationship in an isolated manner; therefore, the success of the interaction must not depend only on the molecules carrying out each function. The physiological state of the three participants, as well as their threshold of perception of the molecular signals exchanged are also important factors. This regulation does not depend only on the genic background of the fungus; the signals generated by the plant and by the antagonist are also important (Ramirez-Valdespino et al., 2019).
Conclusion
The vascular wilt disease in tomato plants, caused by Fusarium oxysporum f. sp. lycopersici (Fol), is becoming increasingly relevant due to its important losses in production, which lead to a negative economic impact. To counteract this problem, the implementation of diverse microorganisms is becoming increasingly frequent and studied, including Trichoderma spp., which can face this type of effects in agriculture, and particularly in commercially important crops such as tomato, and its beneficial potential for interaction with plant species and antagonistic interaction with pathogenic microorganisms have been used as a feasible option to improve its ability of resistance and ideal growth and development.
It is crucial to highlight the importance of genomics, metabolomics, proteomics and transcriptomics, among other molecular tools, which currently help unveil implied pathways, both in pathogenesis and in an immune response in plants and in the production of metabolites in antagonistic organisms, making it easier to understand the plant-pathogen-biocontroller relations more easily, and in the long term, to contemplate useful options to inhibit the effect of phytopathogens. From this analysis, and as a possible strategy for a better use of the interactions between these microorganisms, investigations focused on genetic improvement are suggested, as well as the development of molecular markers from biolistics and other current systems for gene transfer and sequencing such as those involved in the symbiosis between Trichoderma spp. and the tomato plant, facilitating the production of crops that are more resistant, sustainable, profitable and less harmful to both the environment and consumers, due to the reduced habitual use of agricultural chemicals.