Citrus fruits, especially orange (Citrus sinensis), Persian lime (C. latifolia), Mexican lemon (C. aurantifolia), mandarin (C. reticulata), and grapefruit (C. paradisi), are the most cultivated and consumed fruits throughout the world (Iqbal et al., 2018). They are considered universal fruit trees because they are present in more than 100 countries and are the most economically important group of fruits, representing 20% of the world fruit market (Franco et al., 2015). Mexico is the fourth largest citrus producer worldwide (FAOSTAT, 2019). Its citrus-producing areas are in tropical and subtropical areas, which causes phytosanitary problems. Among the most important diseases of citrus trees are those caused by bacteria, viruses, viroids, and fungi (Holguín et al., 2012).
In 2018, in Papantla, Veracruz, ‘Valencia’ orange trees (Citrus sinensis) were observed with symptoms of chlorosis, defoliation, and wilting of branches (Figure 1). This disease poses an alarming problem for citrus growers due to the economic losses it can cause and the fact that the causative agent is still unknown.
The main diseases inducing leaf drop and wilt in citrus trees are caused by the following genera: Lasiodiplodia spp., Phytophthora spp., and Fusarium spp. (Hannachi et al., 2014; Savita and Nagpal, 2012; Valle-De la Paz et al., 2019). The fungi of the genus Fusarium belong to the kingdom Fungi, phylum Ascomycota, order Hypocreales. They comprise a great diversity of fungi species that can be saprophytic or pathogenic to plants, animals, and humans (Moretti, 2009). They are filamentous, cosmopolitan, have well-developed septate mycelium and characteristic conidiophores (Villa-Martínez et al., 2014). The Fusarium species complex is among the 10 most important fungal pathogens of plants (Dean et al., 2012) due to its negative economic impact on world agriculture. These fungi are the causal agents of vascular wilting and basal rot in a wide variety of plants. Root rot caused by Fusarium spp. is a destructive disease of citrus crops (Yaseen and D’Onghia, 2012). More than 40 phylogenetic strains of Fusarium that affect citrus trees have been reported (Sandoval-Denis et al., 2018). In Mexico, Parra-Cota et al. (2018) made the first report of wilt in ‘Valencia’ orange trees, caused by seven isolates of the Fusarium solani species complex in the Yaqui Valley, Sonora. This fungus can associate with other microorganisms and act synergistically with them (Lamichhane and Venturi, 2015). This is why determining the pathogenicity is essential for the identification of microorganisms associated with diseased plants. When the association of an organism with a disease turns out to be constant, it is important to comply with Koch’s postulates to confirm its pathogenicity.
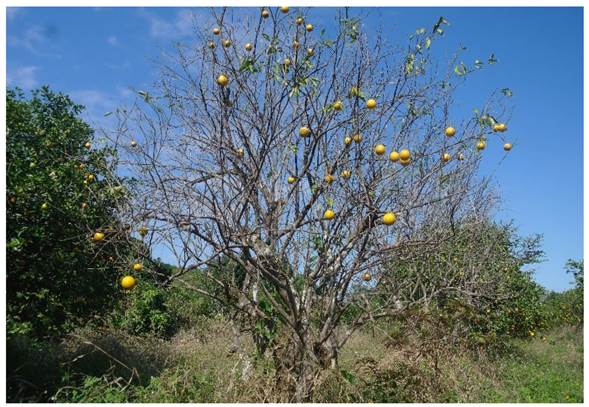
Figure 1 ‘Valencia’ orange trees (Citrus sinensis) with severe wilting and defoliation in Papantla, Veracruz.
Chemical control is the main strategy for the management of fungal diseases (Villa-Martínez et al., 2014). The disinfestation of substrates with hydrogen peroxide, sodium hypochlorite, quaternary ammonium salts, and formaldehyde is the first preventive barrier against Fusarium inoculum (Vásquez-Ramírez and Castaño-Zapata, 2017). The application of benzimidazole-based fungicides such as benomyl, carbendazim, thiabendazole, and thiophanate helps reduce rot caused by Fusarium avenaceum, Fusarium culmorum, Fusarium equiseti, and Fusarium solani (Voigt, 2002). It has also been reported that fungicides based on organomercury and formalin inhibit Fusarium spp. growth (Cook, 1981). The application of methyl bromide fumigant was the most common alternative for managing rot diseases until it was withdrawn from the market in 2005. Nowadays, the application of fumigants such as 1,3-dichloropropene, chloropicrin, sodium N-methyl dithiocarbamate, and methyl isothiocyanate, is mostly used in pre-planting (McGovern, 2015; Vásquez-Ramírez and Castaño-Zapata, 2017). The present study aimed to morphologically and molecularly identify the causal agent of citrus wilt in the municipality of Papantla, Veracruz, to check if Koch’s postulates were fulfilled in three citrus varieties under greenhouse conditions, and to evaluate the in vitro sensitivity of the pathogen to different fungicides.
Materials and methods
The experiment was carried out in the Laboratory of Agricultural Parasitology of the Universidad Autónoma de Chapingo, Mexico.
Isolation and purification. Roots and stems of eight diseased ‘Valencia’ orange trees were collected in four farms located in the San Pablo ejido, in the municipality of Papantla, in the state of Veracruz, Mexico. The site is located at 20° 27’ N and 97° 11’ W, at 45 meters above sea level. Pieces of 1 cm in length were cut in the laboratory and disinfected in sodium hypochlorite solution (1%) for one minute, rinsed twice with sterile water, and dried with sterile paper towels. Under aseptic conditions, six pieces of tissue from each sample were placed in each Petri dish containing Potato Dextrose Agar (PDA) culture medium (200 g potato, 15 g dextrose, 20 g agar in 1000 mL of distilled water), and were then incubated at room temperature for 24 h, under a 12 h light/12 h dark cycle. To obtain pure isolates, a mycelium fragment was transferred into water agar culture medium. After 24 h, small pieces of culture medium containing hyphal tips were transferred into individual Petri dishes with PDA medium.
Morphological characterization. Three standard culture media, Leslie and Summerell’s (2006), Carnation Leaf-piece Agar (CLA), Spezieller Nährstoffarmer Agar (SNA), and PDA were used to identify the species under study. Semi-permanent preparations were made of eight isolates of the fungus and glycerin (50%), with five replicates per isolate. The structures were examined using a compound microscope (Nikon SMZ800®, USA) with an integrated digital camera. The 40x objective was used to take photographs, which were analyzed with the program Motic Imagen v. 3.0 (Motic Group, 2018) to perform the qualitative (shape) and quantitative (length x width) characterization of 50 macroconidia, 50 microconidia, and 20 chlamydospores per preparation. The eight fungal isolates presented the same morphological characteristics. Consequently, it was decided to work with a single isolate for further analysis.
Pathogenicity test. To determine the infective potential of the fungal isolate in citrus trees, three 14-month-old plants of different varieties were inoculated: ‘Valencia’ orange ‘Volkameriana’ rootstock (T4), ‘Delicias’ mandarin ‘Sour’ rootstock (T5), and ‘Valencia’ orange ‘Sour’ rootstock (T6), with their respective negative controls T1, T2, T3, with three repetitions each, in a completely randomized experimental design. Once the greenhouse experiment was set up, the plant roots were inoculated by immersion (Güler and Güldür, 2018; Herman and Perl-Treves, 2007). All the plants were removed from the substrate trying to do the least damage possible. The roots were washed with common water before inducing slight wounds in them with the help of scissors to create entrance routes for the Fusarium isolate. Subsequently, the roots were placed in a suspension of conidia (1 x 106 spores mL-1) for 24 h. Negative controls were subjected to the same treatment but their roots were immersed in sterile water. The plants were placed individually in pots containing sterile Peat Moss (PRO-MIX FLEX) and kept in a greenhouse with a day and night temperature of 39 ± 2 °C and 15 ± 2 °C, respectively, and relative humidity of 40-83%. The growth of the vegetative shoots and the height of the plants were recorded on days 1, 15, 60, and 110 after inoculation. At 110 days after inoculation, the microorganisms were purified and described to check if they corresponded to the isolate of Fusarium sp. previously inoculated, and to confirm their pathogenicity. The isolation of the fungus in the plant tissue was carried out by direct seeding in PDA culture medium, according to the method proposed by Leslie and Summerell (2006), to comply with Koch’s postulates.
In vitro fungicide sensitivity test. Five fungicides were evaluated at different concentrations: Chlorothalonil (1, 5, 10, 100 and 300 mg L-1), Benomyl (1, 5, 10 and 100 mg L-1), Thiabendazole (0.1, 1, 5, 10 mg L-1), Bacillus subtilis (0.005, 0.05, 0.1 and 1 mg L-1), and Prochloraz (1, 5 and 10 mg L-1), plus a negative control that consisted of PDA medium without fungicide. Each treatment consisted of three replications in a completely randomized experimental design. To evaluate the efficacy of the fungicides, 5-day-old mycelium discs of 5 mm in diameter were seeded in the center of Petri dishes containing PDA culture medium mixed with the aqueous solution of the corresponding fungicide. All the seeded Petri dishes were labeled, sealed, and incubated at room temperature (26 ± 1 °C) under continuous darkness. The diameter of the mycelial growth was measured every 48 h until the negative control covered the Petri dish completely. The percentage of inhibition was calculated using Abbott’s formula: (Control-Treatment)/Control x 100 (Miranda-Granados et al., 2018).
DNA extraction, PCR, and sequencing. DNA extraction was performed using the Plant DNeasy Mini Kit (Qiagen) following the manufacturer’s instructions. The PCR was carried out using primers EF1-728F/EF1-986R to amplify a 320-base pair (bp) fragment (Carbone and Kohn, 1999). The reaction mixture was prepared in a final volume of 50 µL with PCR IX buffer, 2.5 mM MgCl2, 0.2 mM dNTP, 0.8 µM of each oligo, 0.04 U DNA polymerase (Promega, USA), and 4 ng of DNA. The PCR was performed in a C1000 thermal cycler (Bio-Rad, USA). An initial denaturation was carried out at 95 °C for 3 min, 35 cycles of 95 °C for 30 s, 54 °C for 30 s, 72 °C for 1 min, and a final extension of 72 °C for 10 min. The amplified products were verified by agarose gel (1%) electrophoresis. The gel was visualized under ultraviolet light using an M-26X transilluminator and a GelDoc-It™ 300 imaging system (UVP, USA). The amplified fragments were purified using the DNA Clean & concentrator-5TM protocol (Zymo Research, USA). The purified DNA fragments were sent to Macrogen® for sequencing.
Phylogenetic tree. To confirm the identity of the pathogen, the sense and antisense sequences were aligned using the software MEGA X 10.0.5 (Kumar et al., 2018). The consensus sequence obtained was compared with those registered in the Basic Local Alignment Search Tools (BLAST) of the National Center for Biotechnology Information (NCBI). To construct the phylogenetic tree and identify the clade where the consensus sequence is grouped (Fusarium sp. Isolated Veracruz), the sequences of the translation elongation factor 1 alpha (TEF-1ɑ) of the species complex comprising Fusarium solani, F. oxysporum, and F. citricola, which affect citrus plants, were downloaded from GenBank (Liu et al., 2020; Sandoval-Denis et al., 2018; Yaseen and D’Onghia, 2012), as well as the sequences that were most similar to those of the species under study (Table 1). The species Colletotrichum truncatum (KM818513) was used as an external group. Multiple sequence alignment was performed using MUSCLE. The phylogenetic tree was constructed by the Maximum Likelihood (ML) method with 1500 bootstrap repetitions, based on the two-parameter Kimura model and the gamma distribution. The model used was obtained using the model test function in the MEGA X software.
Table 1 Sequences of Fusarium isolates obtained from GenBank and used in the phylogenetic tree (Sandoval-Denis et al., 2018).
Species | Strain | Host | GenBank Access Number |
---|---|---|---|
TEF-1ɑ | |||
F. solani | CPC 28189 | Citrus sinensis | LT746226 |
F. solani | CPC 27193 | C. sinensis | LT746222 |
F. solani | CPC 27198 | C. sinensis | LT746223 |
F. solani | CPC 27192 | C. sinensis | LT746221 |
F. macrosporum | C. sinensis | LT746218 | |
F. croci | C. sinensis | LT746216 | |
F. oxysporum | CPC 28190 | C. sinensis | LT746206 |
F. oxysporum | CPC 27700 | C. sinensis | LT746203 |
F. oxysporum | CPC 27196 | C. sinensis | LT746202 |
F. oxysporum | CPC 27194 | C. sinensis | LT746201 |
F. citricola | CPC 27813 | C. reticulata | LT746198 |
F. citricola | CPC 27709 | C. sinensis | LT746196 |
F. citricola | CPC 27069 | C. sinensis | LT746195 |
F. citricola | CPC 27067 | C. limon | LT746194 |
F. polyphialidicum | Vanilla planifolia | GQ425229 | |
F. concolor | KY794889 |
Experimental design and statistical analysis. The data obtained was subjected to an analysis of variance for the variables of plant height and percentage of inhibition of mycelial growth. Tukey’s test for multiple comparisons of means (P≤0.05) was used for variables with significant effect. The statistical de analysis was carried out using the statistical package SAS 9.0 (SAS Institute, 2002).
Results and discussion
Morphological identification. Eight isolates were obtained. They presented a circular border, white and orange mycelium with a diameter of 2.6 cm after three days of growth in PDA medium. Macroconidia with two to three septa were observed in CLA medium, with the dorsal side more curved than the ventral side and measuring 16-30 x 3-6 μm. The microconidia presented zero or one septum, oval in shape, 9-17 x 3-5 μm in size. In CLA medium, chlamydospores measured 6-10 x 6-10 µm, and pale yellow sporodochia were formed (Figure 2). According to the molecular and morphological characteristics observed here, the eight isolates correspond to the genus Fusarium (Leslie and Summerell, 2006). The fungal isolates presented the same morphological characteristics. Consequently, it was decided to work with a single isolate in subsequent studies.
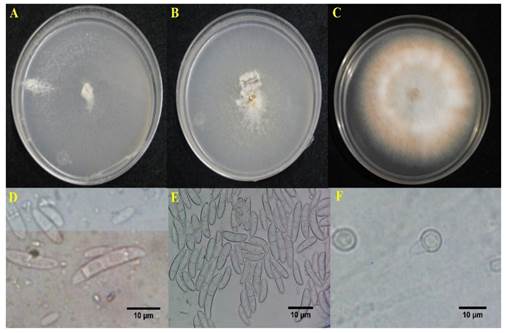
Figure 2 Fusarium sp. isolated from ‘Valencia’ orange tree (Citrus sinensis). A) Growth of Fusarium sp. in SNA medium; B) in CLA medium; C) in PDA; D) macroconidia; E) microconidia; F) chlamydospores.
Pathogenicity test. Ten days after inoculation, all plants that were in contact with the spore suspension exhibited wilt symptoms on vegetative shoots. Sandoval-Denis et al. (2018) reported that Fusarium sarcochroum causes regressive death in lemon and mandarin branches in Italy and Spain. In the present study, the inoculated plants showed little growth of the root system and a brown or dark reddish-brown coloration in the vascular bundle. This partially agrees with what was reported by Hannachi et al. (2015), who observed a reduction in growth and a limited number of roots in citrus plants inoculated with Fusarium oxysporum f. sp. citri, three weeks after inoculation. The fungus was isolated from root tissue with vascular necrosis, fulfilling Koch’s postulates (Figure 3).
The non-inoculated treatments of ‘Valencia’ orange ‘Volkameriana’ rootstock (T1), ‘Delicias’ mandarin ‘Sour’ rootstock (T2), and ‘Valencia’ orange ‘Sour’ rootstock (T3), had a height of 103 to 112 cm 110 days after inoculation, while the inoculated treatments (T4, T5, and T6) were between 85 and 93 cm in height. This meant a 16.9-17.4% reduction in height compared with the non-inoculated treatments (Figure 4). These results indicate that the pathogen can affect the growth of citrus plants, which agrees with the results reported by Baysal-Gurel and Cinar (2015) on grapefruit trees infected with F. oxysporum, which showed reduced vigor and foliage chlorosis.
Fungicide sensitivity test. The analysis of variance showed significant differences between the treatments. The treatments that inhibited mycelial growth were thiabendazole, B. subtilis, and prochloraz (Table 2). Thiabendazole inhibited 100% of mycelial growth starting at a concentration of 5 mg L-1. These results are similar to those reported by Yossen and Conles (2014), who reported that thiabendazole had 99.8% efficacy in the control of F. oxysporum at 4 mg L-1 under in vitro conditions. B. subtilis presented 100% inhibition from 1 mg L-1 and Romero-Velázquez et al. (2015) found that B. subtilis inhibited 100% of the growth of F. oxysporum starting at a concentration of 0.01 mg L-1. The difference in the sensitivity to B. subtilis between the present study and the study of Romero-Velázquez et al. (2015) may be due to the different strains of B. subtilis used in each study. It is possible that the fungi belong to different Fusarium species or that the isolates used in the present study are resistant to B. subtilis (Bardin et al., 2015). Further studies are required to corroborate these assumptions.
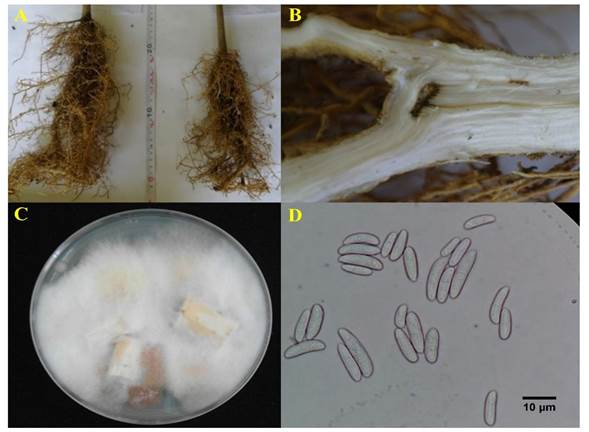
Figure 3 A) Root system of non-inoculated ‘Valencia’ ‘Volkameriana’ rootstock plants (left) vs ‘Valencia’ orange ‘Volkameriana’ rootstock inoculated with Fusarium sp. (right); B) necrosis in the vascular system of the inoculated plant; C) recovery of the inoculated isolate in citrus plants; D) microconidia of the recovered isolate.
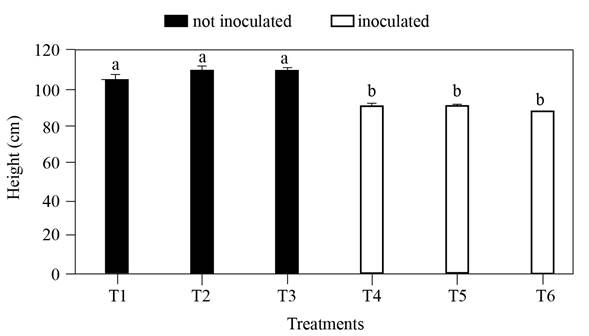
Figure 4 Height of citrus plants inoculated with Fusarium sp. 110 days after inoculation. T1, T4: ‘Valencia’ orange ‘Volkameriana’ rootstock; T2, T5: ‘Delicias’ mandarin ‘Sour’ rootstock; T3, T6: ‘Valencia’ orange ‘Sour’ rootstock. Means with different letters are statistically different (Tukey, P≤ 0.05).
Table 2 Percentage of inhibition of the mycelial growth of Fusarium sp. treated with fungicides and B. subtilis.
Concentration (mg L-1) | Control | Chlorothalonil | Benomyl | Thiabendazole | B. subtilis | Prochloraz |
---|---|---|---|---|---|---|
0.0 hx | ||||||
0.005 | 81.0 bc | |||||
0.05 | 82.3 bc | |||||
0.1 | 0.0 h | 80.6 bc | ||||
1 | 24.3 g | 10.0 h | 33.3 gf | 100.0 a | 75.3 dc | |
5 | 36.6 gf | 73.0 dce | 100.0 a | 84.6 bc | ||
10 | 40.6 f | 74.0 dce | 100.0 a | 92. 3 ba | ||
100 | 61.3 e | 85.3 bc | ||||
300 | 62.0 de | |||||
DMSHy | 13.6 | |||||
CVz (%) | 6.7 | |||||
Media | 64.8 |
Similar studies have found that B. subtilis isolates produce substances such as ammonia and indole acetic acid (IAA), which inhibit the mycelial growth of F. oxysporum (Mohammed et al., 2019; Sharifi and Ramezani, 2003). Prochloraz inhibited 92% of mycelial growth starting at a concentration of 10 mg L-1. These results agree with those reported by Song et al. (2004), who found that the most effective fungicides for inhibiting the mycelial growth of F. oxysporum were prochloraz and carbendazim. Similar results showed that prochloraz inhibited 100% of the growth of F. oxysporum under in vitro conditions at a concentration of 100-1000 mg L-1 (Romero-Velázquez et al., 2015). Amini and Sidovich (2010) demonstrated that under field conditions the application of 10 mg L-1 of bromuconazole and prochloraz completely reduced the severity of the disease caused by F. oxysporum f. sp. lycopersici, causative agent of vascular wilt in tomato.
Molecular identification and phylogenetic tree. BLASTN searches using partial TEF1 sequences from the pathogenic isolate showed 98.7% similarity to F. polyphialidicum (accession No. GQ425229). The phylogenetic tree yielded four highly contrasting lineages, three of which corresponded to the species complex formed by F. oxysporum, F. citricola, and F. solani, currently known as citrus pathogens (Liu et al., 2020; Sandoval-Denis et al., 2018; Yaseen and D’Onghia, 2012). The fourth lineage grouped Fusarium sp. isolated Veracruz, F. polyphialidicum, and Fusarium cf. concolor (Figure 5).
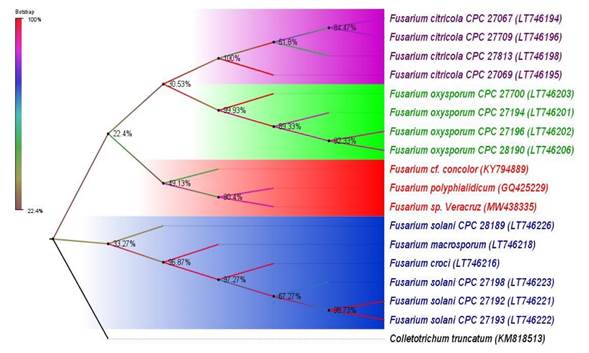
Figure 5 Phylogenetic tree based on the comparison of the sequences of the TEF-1ɑ of Fusarium species. Maximum Likelihood (ML) with 1500 bootstrap repeats, based on the two-parameter Kimura model and the gamma distribution. The isolate under study is Fusarium sp., isolated in Veracruz. The accession numbers of the sequences registered in the NCBI database are indicated in parentheses.
Conclusions
The morphological characterization, molecular identification, and pathogenicity tests confirm that Fusarium sp., and possibly F. polyphialidicum, is the causal agent of vascular wilt in citrus plants from Papantla, Veracruz. The symptoms presented by infected citrus trees were apical bud wilting, growth retardation, decreased number of roots, and necrosis of the vascular system. All three varieties of citrus plants were affected by the pathogen. The fungicides with the highest efficacy in inhibiting mycelial growth were thiabendazole (100%), B. subtilis (100%) and prochloraz (92%) under in vitro conditions.