Servicios Personalizados
Revista
Articulo
Indicadores
-
Citado por SciELO
-
Accesos
Links relacionados
-
Similares en SciELO
Compartir
Revista mexicana de fitopatología
versión On-line ISSN 2007-8080versión impresa ISSN 0185-3309
Rev. mex. fitopatol vol.36 no.2 Texcoco may./ago. 2018
https://doi.org/10.18781/r.mex.fit.1710-1
Scientific articles
Multilocus phylogenetic analysis of fungal complex associated with root rot watermelon in Sonora, Mexico
1Laboratorio de Biología Molecular, Departamento de Agricultura y Ganadería, Universidad de Sonora, Carretera Hermosillo-Bahía Kino Km. 21, Hermosillo, Sonora, CP. 83000, México
2Posgrado en Biociencias, Departamento de Investigaciones Científicas y Tecnológicas, Universidad de Sonora, Luis Donaldo Colosio S/N, Colonia Centro, Hermosillo, Sonora, CP. 83000, México
3Universidad Estatal de Sonora, Avenida Ley Federal del Trabajo S/N, Colonia Apolo, Hermosillo, Sonora, CP. 83000, México.
The state of Sonora is one of the main producers of watermelons in Mexico. Each year, agricultural producers deal with phytosanitary issues like soilborne pathogens. In this study the presence of phytopathogenic fungi associated to watermelon root rot was analyzed in the main production regions of Sonora. Morphological analysis revealed three genera: Fusarium (73%), Ceratobasidium (20%) and Rhizoctonia (6%). Through a multilocus phylogenetic analysis (ITS1, TEF and RPB2 for Fusarium; ITS1 and RPB2 for Rhizoctonia and Ceratobasidium), the following species were identified: Fusarium falciforme, F. brachygibbosum and F. oxysporum. In addition to this, two anastomosic groups for Ceratobasidium sp. (AG-F y AG-A) and two for Rhizoctonia spp. (AG-4 y AG-6) were identified. Pathogenicity assays showed that the representative isolates from these five different species caused root rot wounds and wilting in watermelon plantlets 21 days post inoculation. In this study, F. falciforme is reported for the first time and anastomosic groups for Rhizoctonia and Ceratobasidium are defined as causal agents of watermelon root rot in the region.
Key words: MLST; Fusarium; Ceratobasidium; Rhizoctonia
El estado de Sonora es uno de los principales productores de sandía en México. Cada año, los productores locales enfrentan problemas fitosanitarios, provocados principalmente por hongos del suelo. En el presente estudio se analizó la presencia de hongos patógenos asociados con pudrición de raíz en plantas de sandía en las dos zonas de mayor producción en Sonora. El análisis morfológico reveló la presencia de tres géneros de hongos: Fusarium (73%), Ceratobasidium (20%) y Rhizoctonia (6%). Mediante un análisis filogenético multilocus (ITS1, TEF y RPB2 para Fusarium; ITS1 y RPB2 para Rhizoctonia y Ceratobasidium), se identificó a: F. falciforme, F. brachygibbosum y F. oxysporum; además de dos grupos anastomósicos de Ceratobasidium sp. (AG-F y AG-A) y dos de Rhizoctonia sp. (AG-4 y AG-6). Aislados representativos de estas cinco especies causaron pudrición de raíz y marchitez de plántulas de sandía a los 21 días después de su inoculación. En este estudio se informa por primera vez de F. falciforme y se define a nivel de grupos anastomósicos las cepas de Rhizoctonia y Ceratobasidium como causantes de pudrición radicular en sandía en la región.
Palabras clave: análisis multilocus; Fusarium; Ceratobasidium; Rhizoctonia
Mexico is the world’s leading exporter of watermelon, with 23% of the total worldwide supply of the fruit (FAO, 2014), mainly to the United States, Canada and the Netherlands. In the last ten years, world exports have grown to an average rate of 8% a year (SAGARPA, 2014). Sonora is the main producer of watermelon (Citrullus lanatus) in Mexico. In 2016, over 9 thousand hectares of this crop were planted in this state (SIAP, 2017). However, one of the limiting factors in Sonora’s watermelon production are root diseases. In 2013, in certain watermelon plantations in the valleys of Guaymas and the Coast of Hermosillo in Sonora, areas that concentrate over 96% of the surface in the state with this crop, it was observed that before physiological maturity, certain plants presented yellowing and wilting of leaves; days later, the plants died. A visual analysis showed the presence of lesions and rotting in the cortex of the base of the stem and the top section of the main root, as well as rotting in the main and secondary roots, typical of diseases caused by fungi (Meza-Möller et al., 2014).
To date there is no knowledge on work published concerning the fungal complexes related to root rotting in watermelon plants grown in Sonora; diagnoses are generally based only on the symptoms of the crop and, in the best of cases, on the morphology of the colonies and the observation of reproductive structures. It is frequently mentioned that the root diseases in watermelon plants produced in the area are due to the attack of Fusarium oxysporum, Fusarium solani or Rhizoctonia solani.
The species belonging to the genus Fusarium (Nectriaceae, Hypocreales, Sordariomycetes, Ascomycota), are ubiquitous and economically very important in agriculture, since most of them are pathogenic for plants. Some of their species also produce toxins harmful to humans and animals. This monophyletic group is composed of 20 clades that include over 300 species. With some exceptions, the Fusarium species produce the multi-shafted, macroconidial features and spindle shapes; but there are also other morphological characteristics that help to tell species apart (O’Donnell et al., 2013; Geiser et al., 2013; O’Donnell et al., 2015).
In the Rhizoctonia complex, the hyphal morphology and configuration of the septum help differentiate the genera, whereas species can be told apart by the number of nuclei present in the somatic cells of young hyphae and the thickness of the guide hyphae, or by the morphometric characteristics of the sexual reproductive structures (Cedeño, 2008). The group of multinuclear Rhizoctonia includes R. solani, R. zeae andR. oryzae.
R. solani [teleomorph: Thanatephorus cucumeris] (Ceratobasidiaceae: Cantharellales: Agaricomycetes: Basidiomycota), groups a heterogenous mixture of strains that cause root rotting in many crops around the world (González, 2013). According to the hyphal fusion analysis (anastomosis), these strains are split into 14 anastomosic groups (AG), labelled between AG-1 and AG-13, plus AG-BI (Carling et al., 2002). The group of binuclear Rhizoctonia corresponds to the teleomorphs Ceratobasidium spp. and Tulasnella spp. According to Sharon et al. (2008), Ceratobasidium is composed of 21 anastomosic groups identified as AG-A to AG-U, some of which are highly pathogenic in different plant species.
In the past, fungus taxonomy was based on the morphology of their reproductive structures in the anamorphic state. The concept of morphological species still prevails as the most common diagnostic method to differentiate fungal species, since the morphological characteristics of individuals are easily traceable and comparable. However, this method is unable to find differences between nearby species, underestimating the true fungal diversity (Taylor et al., 2000).
The molecular techniques based on DNA analysis surpass the disadvantages of morphological identification, since they are quick, precise, objective and applicable to a large number of samples. They help differentiate between genotypes and to establish genetic variability indices within a population (Narayanasamy, 2011). In recent years, the concept of phylogenetic species between filamentous fungi has become increasingly popular, based on the consistency of multiple DNA gene sequences; this phylogenetic approach helps define species better (Taylor et al., 2000; Choi et al., 2013).
Since White et al. (1990) published the sequences of primers that allowed the amplification and sequencing of sections of the rDNA operon, an interest arose in phylogenetic research, which now dominates fungal taxonomy. The sequencing of the ITS fragments of rDNA continues to be the most widely accepted approach in molecular mycology to classify and identify specimens or cultures of unknown fungi. However, its resolution in taxonomic relations of a higher level is inferior to many other genes. Numerous studies have been carried out to identify loci with characteristics of adequate primary barcodes. The AFTOL (Assembling the fungal tree of life) project, completed in 2008, has established a phylogeny based on the amplification of genes RPB1, RPB2, nucLSU, nucSSU, mtSSU, TEF1α and mtATP6 (Stielow et al., 2015). Knowing the species that cause a disease is crucial for their adequate management and control.
Based on this, the aim of the present investigation was to identify the fungus species that cause rotting of the roots in watermelon plants in the Coast of Hermosillo and Valley of Guaymas in Sonora, based on morphological and multilocus phylogeny analyses.
MATERIALS AND METHODS
Sampling: The study was carried out during the spring-summer cycles of 2013 and 2014, in four commercial fields, two of which were located on the Coast of Hermosillo (CH1, CH2) and two in the Valley of Guaymas (VG1, VG2), in Sonora, Mexico, which account for 10% of the state’s surface used for the production of watermelon. The varieties planted in these fields were Sugar Red, SuperSeedless 7187HQ F1 and Precious Petit. In each cycle, 40 plants (10 plants per field) were collected at random in the areas with wilting and dryness of runners . The samples were placed in polyethylene bags, which were labelled and transported in containers with ice to the laboratory for processing.
Fungal isolation. The plant roots were washed using water, dried with paper towels, and cut into 1 cm pieces. Segments were taken from the crown, main root and secondary roots. They were disinfected by submerging them for 2 min in a solution prepared with sodium hypochlorite at 6%, ethyl alcohol at 96% and sterilized distilled water in a 1:1:8 ratio, respectivly. They were then placed in dishes with agar-water at 2%. The dishes were incubated at 25 ± 0.1 °C until the mycelium produced from the pieces of plant allowed for the extraction of a hypha tip. The hypha tips were planted successfully in Potato Dextrose Agar (PDA) supplemented with a solution of streptomycin/neomycin and incubated at 25 ± 0.1 °C, until a pure culture was obtained.
Morphological and cultural characterization. All isolates were grouped based on the characteristics of the cultures and the color developed on both sides of the PDA plate. A representative of each group was used for the morphological characterization. In the isolations with characteristics of Fusarium the presence and morphology of microconidia, macroconidia, and Chlamydospores was established from their growth in carnation leaf agar (CLA), after seven days in the dark at 25 ± 0.1 °C (Leslie and Summerell, 2006). The isolations with characteristics of Rhizoctonia genus were identified by observing vegetative characteristics such as the color of the mycelium, septa, constrictions near the ramifications, during growth in PDA or Malt Extract Agar (MEA). To determine the number of nuclei, hyphae were stained with trypan blue in lactophenol. Growth rate was determined in PDA, keeping the cultures at 25 ± 0.1 °C and photoperiods of 14h/10h of light/darkness. The diameter of the culture was measured every 24 h until the mycelium covered the dish completely (Sneh et al., 1996). All isolations were initially identified up to the genus level.
DNA Extraction. A mycelia from pure cultures in PDA were taken with a sterile microbiological spatula and placed in a tube of the Kit Power Soil DNA Isolation (MoBIO Laboratories, California, U.S.A.). Cell lysis was carried out in a Precellys Evolution Homogenizer (Bertin Technologies, France), stirring the tubes at 6500 rpm for three 20 s cycles with 20 s pauses. DNA integrity was verified in a 2% agarose gel. The extracted DNA was quantified in the NanoDrop 1000 (ThermoScientific). Samples with an absorbance ratio of 260/280 between 1.8 and 2 were amplified. The DNA was stored at -20°C until its use.
DNA amplification sequencing. The non-codifying region of the internal transcribed spacer (ITS) was amplified with a segment of the region that codified for the DNA-directed RNA polymerase II second largest subunit (RPB2) of all the isolations obtained. In addition, it was amplified the region that codifies for translation elongation factor 1-α (TEF1) for the isolates with Fusarium characteristics. Information about the primers used is shown in Table 1.
Table 1 Oligonucleotides used in this study.
Locus | Primer | Oligonucleótidos (5'-3') | (pb) | Referencia |
ITS | ITS1 ITS4 | TCCGTAGGTGAACCTGCGG TCCTCCGCTTATTGATATGC | = 550 | Whiteet al.(1990) |
TEF-1a | EF1 EF2 | ATGGGTAAGGARGACAACAC GGARGTACCAGTSATCAT | = 700 | O'Donnell et al. (1998) |
RPB2x | RPB2-5F2 fRPB2-7cR | GGGGWGAYCAGAAGAAGGC CCCATRGCTTGYTTRCCCAT | = 900 | O'Donnell et al. (2013) |
RPB2z | RPB2-980F fRPB2-7cR | TGYCCIGCIGARACICCHGARGG CCCATRGCTTGYTTRCCCAT | = 674 | Gonzálezet al.(2016) |
x Specific to the genus Fusarium
z Specific to the genus Rhizoctonia
The polymerase chain reaction was carried out by mixing 12.5 ml of GoTaq® Green Master Mix (Promega), 1 μl of each forward and reverse primer (IDT Technologies) 10 mM, 1 μl of DNA (1 ng/ μl)and molecular biology degree water up to 25 μl final volume. The PCR products were separated by electrophoresis in agarose gel at 2%, dyed with GelRed (Biotium Inc) in 5X Green GoTaq reaction buffer (Promega) 15 μl:1ml. They were visualized in UV light (DigiDoc-It™, UVP) observing the size of the amplicon and its purity. Purification was carried out using ExoSAP-IT PCR Product Cleanup (Affymetrix) or with by cutting bands of the expected size with the Wizard® SV Gel kit and PCR Clean-Up System (Promega). Purified amplicons were sequenced in both directions with ABI 3730xl DNA Analyzer (Applied Biosystems) in GENEWIZ. Each sequence was reviewed manually and nucleotides in ambiguous positions were corrected with complementary sequences obtained with both primers, using the software ChromasPro v2.1.6. The sequences from regions ITS, RPB2 and TEF were compared by alignment with those contained in the databases Fusarium MLST (http://www.cbs.knaw.nl/Fusarium), Fusarium ID (http://isolate.fusariumdb.org) and in the National Center for Biotechnology Information (NCBI), in which the sequences from regions ITS and RPB2 of Rhizoctonia spp. were also compared, using the “Basic local alignment search tool” (BLAST) (Altschul et al., 1990).
Phylogenetic analysis. The Fusarium species and the anastomosic groups of Rhizoctonia and Ceratobasidium were determined separately in two data matrices. In each case, several alignments were carried out using the software Clustal Omega (Sievers et al., 2011). The sequences were linked and edited using the software UltraEdit32. The phylogenetic analysis of each data matrix was carried out separately under the criterion of Maximum Likelihood Estimation (MLE) with the software PAUP 4.0a152 (Swofford, 2002). The best nucleotide substitution model was established using ModelTest (Posada and Crandall, 1998).
The trees were viewed and modified in FigTree and exported to graphic editors. The consensus trees for Rhizoctonia spp. and Fusarium spp., were rooted using Botryobasidium simile (isolation GEL2348) and Neofusicoccum parvum (strain CCF216), respectively.
Pathogenicity tests. In compliance with Koch’s postulates, to assure that the isolates obtained were the causal agents of the root rotting in watermelon plants, a monosporic culture was chosen at random from each one of the species identified in the molecular analysis. The treatments were: 1) Non-inoculated control, 2) F. falciforme, 3) F. oxysporum, 4) F. brachygibbosum, 5) R. solani, 6) Ceratobasidium sp, 7) F. brachygibbosum + F. solani, 8) F. brachygibbosum + F. oxysporum, 9) R. solani + Ceratobasidium sp. 10) Ceratobasidium sp. + F. solani and 11) Ceratobasidium sp. + F. oxysporum. Healthy plants of the varieties SuperSeedless 7187HQ F1 and Precious Petit, aged 21 days, established in pots with a mixture of soil and perlite (1:3). Six plants were inoculated for each treatment. Each plant was treated with rotting and discoloration of vascular bundles, typical symptoms of damages by Fusarium spp. Four discs, 8 mm in diameter, were placed around the root. Healthy plants treated with sterile PDA discs were used as controls. The pots were placed in a controlled environment chamber at 25 ± 0.1 °C, with a photoperiod of 14h/10h day/night, until the appearance of symptoms. Irrigation was carried out based on water requirements, and a Hoagland nutrient solution was applied on a weekly basis. The number of disease plants was observed. The percentage of infected roots was determined using ten root segments from each plant. These tissue fragments were disinfected separately and placed in PDA. The evaluation was carried out observing the development of mycelia after seven-day incubation.
RESULTS
Damages observed during sampling. Figure 1A shows the damages observed in the crop. Plants with symptoms displayed different types of rotting in the crown, stem, roots and rootlets. One type of lesion was small, concave or not, brownish (Figure 1B) and moist-looking, typical in Rhizoctonia sp. In certain cases, this type of lesions was observed to have small pustules. In other plants, lesions were light brown, discolored and with vascular beam rot, typical in symptoms of damages by Fusarium spp. (Figure 1C).
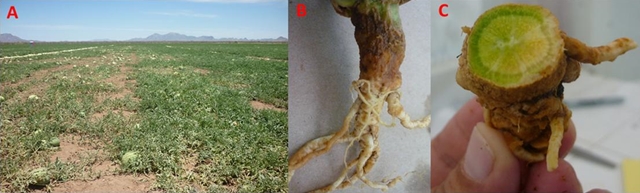
Figure 1 Damage on planted watermelon crops. A) Damage on the field, B) Roots of diseased plants, C) Vascular damage.
Isolation and morphology of the colonies and reproductive structures of fungi. A total of 45 fungal isolates were obtained from the four sampling sites, and in each one, at least one species of Rhizoctonia and Fusarium was isolated. The distribution of species by site is shown in Figure 2.
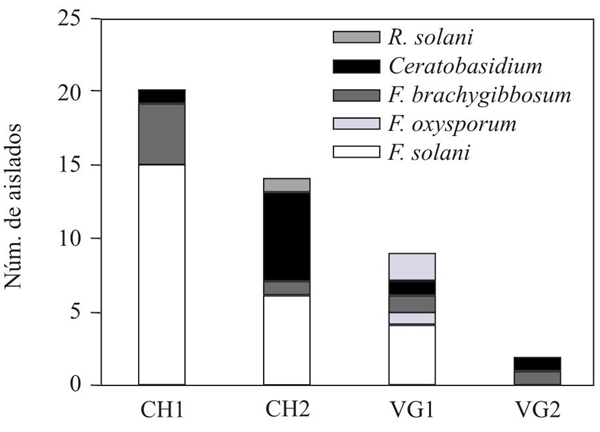
Figure 2 Distribution of pathogenic fungi species, by sampling sites. CH1= Coast of Hermosillo 1. CH2=Coast of Hermosillo 2. VG1=Valley of Guaymas 1. VG2=Valley of Guaymas 2.
The morphological analysis helped to form two groups of isolates. One group was formed of 13 isolations that presented colonies with abundant aerial hyphae, ivory-colored at first, turning light brown or brown after 7 days. In these isolations, hyphae were robust, with branches in right angles, constriction of the ramification and the formation of a septum near the point of origin, without spores, all of which are typical characteristics of Rhizoctonia sp. (Sneh et al., 1996). Average growth rate was 1.25 mm h-1. Nuclei staining revealed the presence of isolates with both binuclear and multinuclear cells (Figure 3).

Figure 3 Morphology of the colonies and colors of the medium in PDA of Rhizoctonia spp., Ceratobasidium sp., F. falciforme, F. oxysporum, F. brachygibbosum, (1A-5A and 1B-5B) respectively. (1C) Polynuclear hyphae. (1D) Septa. (2C) Binuclear hyphae. (2D) Monilioid cells. (3C) Monophialides and microconidia. (3D) Macroconidia. (4C) Microconidi in situ in CLA. (4D) Microconidia. (5C) Macroconidia. (5D) Clamidospores.
The second group of 32 isolates showed the formation of macroconidia, microconidia, chlamydospores and monophyalides in CLA, typical of Fusarium sp., according to descriptions by Leslie and Summerell (2006). Twenty-five isolations fit the description of F. solani: cream-colored colonies with red to dark gray pigments in the obverse oval-shaped microconidia without septa, long monophyalides, single or paired chlamydospores, abundant straight macroconidia with 3 to 5 septa. Other 2 presented typical characteristics for F. oxysporum: cottonlike mycelia, scarce white to pale violet and purple color in the agar; short monophyalides. The rest of the isolates produced white mycelia, which turned pink, with yellow sporodochia; oval unicellular microconidia, produced in monophyalides, curved macroconidia with 3 to 5 septa, with wide central cells, slightly pointy apex, single or chained chlamydospores (Figure 3). No isolations were found with different morphology to Rhizoctonia or Fusarium.
Molecular identification and phylogenetic analysis of fungi. A first BLAST analysis from the region between the internal transcribed spacers I and II (ITS1-ITS2) of all the isolates helped determine that 25 sequences had a similarity of 99-100% with F. solani; 5 to F. brachygibbosum, 2 to F. oxysporum, 10 to Ceratobasidium sp. and 3 sequences to Rhizoctonia solani also with a similarity of 99-100% to NCBI homologous sequences.
The phylogenetic analysis under the ML criterion for the Fusarium genus was carried out with the linked matrix of genes ITS, RPB2 and TEF1 of the 32 isolations of this study and of 25 reference strains deposited in culture collections (Al-Hatmi et al., 2016). The best nucleotide substitution model was TIM2+I+G. Total of 1816 nucleotides were considered in the data set. The multilocus analysis helped define the correct identity of the isolations initially proposed as F. solani, since they form a separate clade with a similarity of 100 % with the type isolations of F. falciforme, a member of the Fusarium solani species complex (FSSC). The identity of F. brachygibbosum and F. oxysporum was corroborated (Figure 4).
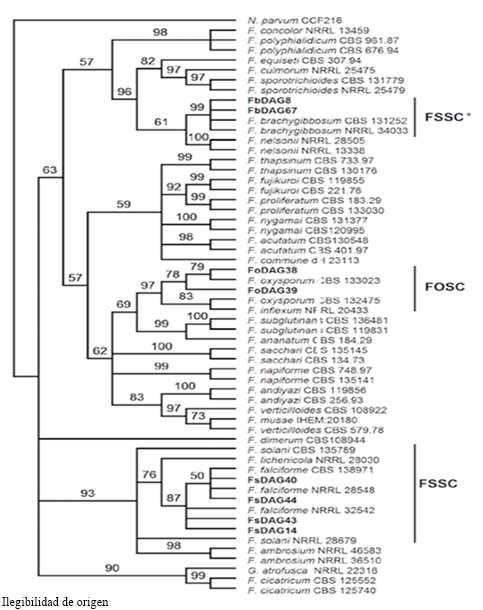
Figure 4 Phylogeny by MV of the chained sequences of the genes ITS, EF and RPB2 of 8 Fusarium spp. isolations (in bold) representative of 29 obtained from watermelon plants with wilting and root rot. Bootstrap of 1000 replications; external group: Neofusicoccum parvum.
A similar analysis was carried out with the sequences of isolations from Rhizoctonia and Ceratobasidium sp. a data matrix was integrated by the regions ITS and RPB2 of 49 isolations, including those from the present study and 36 references strains (Cantharellales), obtained from culture collections (González et al., 2016). The best nucleotide substitution model was GTR+I+G. Total of 1355 nucleotides were considered from each isolation in the data set. This analysis established the identity of two anastomosic groups for the genus Rhizoctonia, AG-4 and AG-6 and two for the genus Ceratobasidium, AG-A and AG-F (Figure 5). Table 2 shows the identity and the accession numbers of the sequences obtained.

Figure 5 Phylogeny by MV of the chained sequences of the genes ITS, EF and RPB2 of 8 Rhizoctonia spp. isolations (in bold) representative of 16 obtained from watermelon plants with wilting and root rot. Bootstrap of 1000 replications; external group: Botryobasidium simile. AG=Anastomotic group.
Table 2 Identity, location and accession numbers of sequences obtained in this study.
Identidad | Nombre del aislado | Sitio | Número de accesión en el GenBank | ||
ITS | EF | RPB2 | |||
F. falciforme(FSSC) | FsDAG10 | CH2 | KX583230 | KY514171 | MF939148 |
F. falciforme(FSSC) | FsDAG11 | CH1 | KX583231 | KY514185 | MF939149 |
F. falciforme(FSSC) | FsDAG14* | CH2 | KX583232 | KY514172 | KY637066 |
F. falciforme(FSSC) | FsDAG36 | CH2 | KX583233 | KY514173 | MF939150 |
F. falciforme(FSSC) | FsDAG43* | CH1 | KX583234 | MF939167 | KY637067 |
F. falciforme(FSSC) | FsDAG44* | CH1 | KX583235 | KY514176 | KY637068 |
F. falciforme(FSSC) | FsDAG45 | CH1 | KX583236 | KY514177 | MF939151 |
F. falciforme(FSSC) | FsDAG46 | CH1 | KX583237 | KY514187 | MF939152 |
F. falciforme(FSSC) | FsDAG48 | CH1 | KX583238 | KY514178 | MF939153 |
F. falciforme(FSSC) | FsDAG49 | CH1 | KX583239 | KY514179 | MF939154 |
F. falciforme(FSSC) | FsDAG50 | CH1 | KX583240 | KY514180 | MF939155 |
F. falciforme(FSSC) | FsDAG51 | CH1 | KX583241 | KY514181 | MF939156 |
F. falciforme(FSSC) | FsDAG52 | CH1 | KX583242 | KY514182 | MF939157 |
F. falciforme(FSSC) | FsDAG53 | CH1 | KX583243 | KY514183 | MF939158 |
F. falciforme(FSSC) | FsDAG54 | CH1 | KX583244 | KY514184 | MF939159 |
F. falciforme(FSSC) | FsDAG30 | CH1 | KX583245 | KY514186 | MF939160 |
F. falciforme(FSSC) | FsDAG37 | CH2 | KX583246 | KY514174 | MF939161 |
F. falciforme(FSSC) | FsDAG29 | CH1 | KX583247 | MF939168 | MF939162 |
F. falciforme(FSSC) | FsDAG40* | VG1 | KX583248 | KY514175 | KY637065 |
F. brachyigibbosum (FSSC)a | FbDAG41 | VG1 | KX583249 | MF939166 | MF939163 |
F. brachyigibbosum (FSSC)a | FbDAG67* | CH1 | KX583250 | KY514170 | KY637062 |
F. brachyigibbosum(FSSC)a | FbDAG8* | CH1 | KX583251 | KY514169 | KY637061 |
F. brachyigibbosum(FSSC)a | FbDAG9 | CH2 | KX583252 | MF939165 | MF939164 |
F. oxysporum f. sp. niveum (FOSC) | FoDAG38* | VG2 | KX583253 | KY514188 | KY637063 |
F. oxysporum f. sp. niveum (FOSC) | FoDAG39* | VG1 | KX583254 | KY514189 | KY637064 |
Ceratobasisium sp.AG-F | CeDAG3* | CH2 | MF804912 | KY637071 | |
Ceratobasisium sp.AG-F | CeDAG5* | VG2 | KX583256 | - | KY637074 |
Ceratobasisium sp.AG-F | CeDAG12* | CH2 | KX583257 | - | KY637072 |
Ceratobasisium sp.AG-F | CeDAG19* | VG2 | KX583258 | - | KY637073 |
Ceratobasisium sp. | CeDAG17 | CH1 | MF804910 | - | MF804913 |
Ceratobasisium sp. | CeDAG22 | CH2 | KX583259 | - | - |
Ceratobasisium sp. | CeDAG23 | CH2 | KX583260 | - | - |
T. praticola=R. solaniAG-4 | RhDAG18* | CH2 | MF804911 | - | MF804914 |
Thanatephorus sp. | RhDAG20* | VG1 | KX583262 | - | KY637069 |
Thanatephorus sp. | RhDAG21* | VG1 | KX583263 | - | KY637070 |
*= Isolations considered in the phylogenetic analysis. FSSC= Fusarium solani Species Complex. FSSCa= Fusarium sambucinum Species Complex. FOSC= Fusarium oxysporum Species Complex. CH1= Coast of Hermosillo1, CH2= Coast of Hermosillo2, VG1= Valley of Guaymas1, VG2= Valley of Guaymas. AG= Anastomosic group. - = Without data.
Pathogenicity tests: The appearance of symptoms in plants took place 14 days after inoculation, in the form of lesions in the roots and the base of the stem. All the isolation and their combinations caused the death of the plants after 21 days. Table 3 shows the percentage of infected roots in each treatment. Control plants presented no symptoms.
Table 3 Percentage of infected roots in the pathogenicity tests.
Tratamiento | SS-7187 HQ F1 | Precious Petit |
Testigo | 0 | 0 |
F. solani | 23 | 66 |
F. oxysporum | 71 | 75 |
F. brachygibbosum | 87 | 100 |
Rhizoctonia solani | 54 | 84 |
Ceratobasidium | 48 | 100 |
F. solani + F. brachygibbosum | 55 | 100 |
F. oxysporum + F. brachygibbosum | 55 | 100 |
R. solani + Ceratobasidium | 71 | 100 |
F. solani + Ceratobasidium | 40 | 80 |
F. oxysporum + Ceratobasidium | 100 | 100 |
DISCUSSION
Although Sonora is the main watermelon producing state in Mexico, there are no formal reports on the fungi related to rotting. The pathological data in this investigation show that, individually or in a group, at least five different fungal species caused root rot, which led to death of watermelon plants before they reached physiological maturity, during the formation and development of fruits. The characteristics of the colonies, morphology, nuclei staining and phylogenetic analysis of sequences helped to identify two species of the Ceratobasidiaceae family: Rhizoctonia solani and Ceratobasidium sp., and three of the Fusarium genus: F. falciforme, F. oxysporum and F. brachygibbosum, showing that there is a diverse community of fungi causing root rot in watermelon planted in the Coast of Hermosillo and the Valley of Guaymas, Sonora.
The predominant species was found to be F. falciforme, with 25 isolations from three fields of the two sites sampled. Initially, this fungus was identified as the polytypic morphospecies F. solani. Based on the linked phylogenetic analysis of the regions ITS, TEF-1α and RPB2 using ML, a separate clade is formed within the Fusarium solani Species Complex (FSSC). This complex groups at least 60 different species that, because of the similarity in the morphology of their conidia, are known as cryptic species. They have a wide range of hosts and have been subdivided into formae speciale, depending on the specificity of the host (O’Donnell et al., 2015). Recent phylogenetic analyses have revealed that each formae speciale belongs to a biologically and phylogenetically different species (Coleman, 2016; O’Donnell et al., 1998). F. falciforme has been reported as a causal agent of wilting and root rot in lima bean plants and chickpea in Brazil (Sousa et al., 2017, Cabral et al., 2016).
Five F. brachygibbosum isolations belonging to Fusarium sambucinum Species Complex (FSSC) were identified in three sampling sites. The characteristics of the colonies coincide with the first description published (Padwick, 1945), they present abundant white to pink aerial mycelia white to amber sclerotia of up to 2.0 mm in diameter, oval or fusiform conidia, hyperbolically curved macroconidia, terminal or alternated chlamydospores, single or in chain, generally unicellular. As a preliminary product of this investigation, F. brachygibbosum was reported for the first time as a pathogenic agent, causing this wilting in watermelon seeds (Rentería-Martínez et al., 2015). It has recently been recorded as a causal agent of rotting in the stem of maize plants (Shan et al., 2017), wilting and regressive death in Euphorbia larica and olive trees (Al-Mahmooli et al., 2013; Trabelsi et al., 2017) and of rotting and cankers in almond and chestnut trees (Stack et al., 2017; Marek et al., 2013).
F. oxysporum f. sp. niveum belongs to the Fusarium oxysporum Species Complex (FOSC); it was only isolated from the fields located in the Valley of Guaymas. The components of FOSC cause vascular wilting and root rot in over 100 different plant species, and based on their specificity with the host, there have been reports of over 80 formae speciale. In this sense, an accurate diagnosis, even before symptoms appear, is crucial for the management of crops (López-Mondéjar et al., 2012).
Regarding the Ceratobasidiaceae family, binuclear Rhizoctonia (teleomorph: Ceratobasidium sp.) was the species with the widest distribution, since it was isolated from all the fields sampled, and most frequently, in field No. 2 of the Coast of Hermosillo. The symptoms observed in roots and stems of infected plants were reddish-brown lesions located and slightly sunken in the base of the stem and 0.2 to 2.0 cm in length. In some cases, the discoloration presented by diseased plants affected almost 90 % of the root system (Meza-Möller et al., 2014).
The phylogenetic analysis by ML showed that the isolations obtained from Ceratobasidium sp. belong to two different anastomosic groups: AG-A and AG-F, previously found in diseased Ipomoea batatas and Arachis hypogaea plants, respectively. The phylogenetic relations of Rhizoctonia fungi show consistency between the clades formed and the anastomosic groups to which they belong (González et al., 2016).
It is important to point out that most of the Ceratobasidium sp. isolates found in this investigation belong to group AG-F, which coincides with earlier works on root rot in watermelon carried out in Arizona, U.S.A (Nischwitz et al., 2013) and Italy (Aiello et al., 2012). Ceratobasidium AG-F is also the causal agent of root rot in strawberry (Sharon et al., 2007), Tagetes erecta (Saroj et al., 2013) and pistachio (Alaei et al., 2017).
There is no previous information on the pathogenicity of the anastomosic group AG-A in watermelon, but there is on sugar beet and apple trees (Wang and Wu, 2012).
The least frequent isolations belong to the genus Rhizoctonia, which, due to the high variability in its geographic distribution, morphology, specificity of hosts, and pathogenicity, has also been proposed as a species complex (González et al., 2006). Isolation RhDAG18 obtained from site CH2 belongs to AG-4 and has been morphologically delimited as Thanatephorus praticola (Mordue et al., 1989), which was later corroborated by the multilocus phylogenetic analysis (González et al., 2016). T. praticola has been presented in association with Ceratobasidium sp. AG-F as part of a fungal complex that cause root rot and deterioration in watermelon plantations in the production stage in Italy (Aiello et al., 2012).
The pathogenicity tests showed that isolated fungi are causal agents of root rot in watermelon plants. The results of the percentage of infected roots showed that both watermelon varieties evaluated are susceptible to the five pathogens identified, inoculated separately or in combinations. In this regard, Aiello et al. (2012) detected percentages of incidence of the disease higher than 81 % when evaluating 6 different isolations of Ceratobasidium from the anastomosic group AG-F.
Due to economic losses resulting from root diseases in the areas of study, direct planting in the soil has been replaced almost entirely with watermelon grafted on patterns resistant to root rot. The commonly used rootstocks in the region are hybrids between Cucurbita maxima x C. moschata, although these hybrids have shown susceptibility to root rot (López-Elías et al., 2010). Some rootstocks have shown to be susceptible to Fusarium in Spain, the main producer of cucurbits in Europe (Armengol et al., 2000). On the other hand, the apparent resistance to wilting by Fusarium of some commercial varieties of watermelon depends on the type and initial concentration of the inoculant (Martyn and McLaughlin, 1983); in this sense, the timely identification of the pathogen will help make a better choice of rootstock. Additionally, once the pathogenic species present has been accurately established, the quantification of its concentration will manage the disease better. Using the quantitative PCR (qPCR) technique, it is possible to detect up to one 1 pg of fungal DNA in plants without symptoms (Haegi et al., 2013).
CONCLUSIONS
In the present study, Fusarium falciforme and Thanatephorus praticola are reported for the first time as causal agents of watermelon plants root rotting in Mexico. The Multilocus analysis corroborated Fusarium brachygibbosum and Ceratobasidium sp. identity, previously reported in the literature. This research is a first attempt at generating data that could lead to broader studies that may contribute to establish adequate control strategies for disease management in commercial watermelon plantations.
LITERATURA CITADA
Aiello D, Vitale A, Hyakumachi M and Polizzi G. 2012. Molecular characterization and pathogenicity of binucleate Rhizoctonia AG-F associated to the watermelon vine decline in Italy. European Journal of Plant Pathology 134:161-165. https://doi.org/10.1007/s10658-012-9973-9 [ Links ]
Alaei H, Molaei S, Mahmoodi SB and Saberi-Riseh R. 2017. New Anastomosis Group F (AG-F) of binucleate Rhizoctonia causing root and stem rot of Pistacia vera. Journal of Crop Protection 6:1-13. https://doi.org/10.18869/modares.jcp.6.1.1 [ Links ]
Al-Hatmi AM, Van Den Ende AH, Stielow JB, Van Diepeningen AD, Seifert KA, McCormick W, Assabqui R, Gräfenhan T, De Hoog GS and Levesque CA. 2016. Evaluation of two novel barcodes for species recognition of opportunistic pathogens in Fusarium. Fungal Biology 120:231-245. https://doi.org/10.1016/j.funbio.2015.08.006 [ Links ]
Al-Mahmooli IH, Al-Bahri YS, Al-Sadi AM and Deadman ML. 2013. First Report of Euphorbia larica Dieback Caused by Fusarium brachygibbosum in Oman. Plant Disease 97:687. https://doi.org/10.1094/PDIS-09-12-0828-PDN [ Links ]
Altschul SF, Gish W, Miller W, Meyers EW and Lipman DJ. 1990. Basic local alignment search tool. Journal of Molecular Biology 215:403-410. https://doi.org/10.1016/S0022-2836(05)80360-2 [ Links ]
Armengol J, José CM, Moya MJ, Vicent A and García JJ. 2000. Fusarium solani f. sp. cucurbitae race 1, a potential pathogen of grafted watermelon production in Spain. EPPO Bulletin 30:179-183. https://doi.org/10.1111/j.1365-2338.2000.tb00875.x [ Links ]
Cabral CS, Melo MP, Fonseca MEN, Boiteux LS and Reis A. 2016. A Root Rot of Chickpea caused by Isolates of the Fusarium solani Species Complex in Brazil. Plant Disease 100:2171. https://doi.org/10.1094/PDIS-05-15-0571-PDN [ Links ]
Carling DE, Kuninaga S and Brainard KA. 2002. Hyphal anastomosis reactions, rDNA internal transcribed spacer sequences, and virulence levels among subsets of Rhizoctonia solani anastomosis group 2 (AG2) and AG-BI. Phytopathology 92:43-50. https://doi.org/10.1094/PHYTO.2002.92.1.43 [ Links ]
Cedeño L. 2008. Método fácil y confiable para teñir núcleos en hongos del complejo Rhizoctonia. Bioagro 20:215-219. Disponible en línea: http://www.redalyc.org/articulo.oa?id=85714153009 (Consulta, agosto 2017). [ Links ]
Choi J, Park SY, Kim BR, Roh JH, Oh IS, Han SS and Lee YH. 2013. Comparative Analysis of Pathogenicity and Phylogenetic Relationship in Magnaporthe grisea Species Complex. PLOS ONE 8: e57196. https://doi.org/10.1371/journal.pone.0057196 [ Links ]
Coleman JJ. 2016. The Fusarium solani species complex: ubiquitous pathogens of agricultural importance. Molecular Plan Pathology 17:146-158. https://doi.org/10.1111/mpp.12289 [ Links ]
Food and Agriculture Organization. 2014. Estadísticas. Disponible en línea: http://faostat3.fao.org/browse/Q/QC/S (Consulta, junio 2017). [ Links ]
Geiser DM, Aoki T, Bacon CW, Baker SE, Bhattacharyya MK, Brandt ME, Brown DW, Burgess LW, Chulze S, Coleman JJ, Correll JC, Covert SF, Crous PW, Cuomo CA, De Hoog GS, Di Pietro A, Elmer WH, Epstein L, Frandsen RJ, Freeman S, Gagkaeva T, Glenn AE, Gordon TR, Gregory NF, Hammond-Kosack KE, Hanson LE, Jímenez-Gasco M M, Kang S, Kistler HC, Kuldau GA, Leslie JF, Logrieco A, Lu G, Lysøe E, Ma LJ, McCormick SP, Migheli Q, Moretti A, Munaut F, O’Donnell K, Pfenning L, Ploetz RC, Proctor RH, Rehner SA, Robert VA, Rooney AP, Bin Salleh B, Scandiani MM, Scauflaire J, Short DP, Steenkamp E, Suga H, Summerell BA, Sutton DA, Thrane U, Trail F, Van Diepeningen A, Vanetten HD, Viljoen A, Waalwijk C, Ward TJ, Wingfield MJ, Xu JR, Yang XB, Yli-Mattila T and Zhang N. 2013. One fungus, one name: Defining the genus Fusarium in a scientifically robust way that preserves longstanding use. Phytopathology, 103:400-408. https://doi.org/10.1094/PHYTO-07-12-0150-LE [ Links ]
González D, Rodriguez CM, Boekhout T, Stalpers J, Kuramae EE, Nakatani AK, Vilgalys R and Cubeta MA. 2016. Phylogenetic relationships of Rhizoctonia fungi within the Cantharellales. Fungal Biology 120:603-19. https://doi.org/10.1016/j.funbio.2016.01.012 [ Links ]
González D. 2013. Identification, molecular characterization, and evolution of group I introns at the expansion segment D11 of 28S rDNA in Rhizoctonia species. Fungal Biology 117: 623-637. https://doi.org/10.1016/j.funbio.2013.06.006 [ Links ]
González D, Cubeta MA and Vilgalys R. 2006. Phylogenetic utility of indels within ribosomal DNA and b-tubulin sequences from fungi in the Rhizoctonia solani species complex. Molecular Phylogenetics and Evolution 40:459-470. https://doi.org/10.1016/j.ympev.2006.03.022 [ Links ]
Haegi A, Catalano V, Luongo L, Vitale S, Scotton M, Ficcadenti N and Belisario A. 2013. A newly developed real-time PCR assay for detection and quantification of Fusarium oxysporum and its use in compatible and incompatible interactions with grafted melon genotypes. Phytopathology 103:802-810. https://doi.org/10.1094/PHYTO-11-12-0293-R [ Links ]
Leslie JF and Summerell BA. 2006. The Fusarium laboratory manual. Ed. Wiley-Blackwell. USA. 388p. [ Links ]
López EJ, Pacheco-Ayala F, Huez-López MA, Rodríguez JC, Jiménez-León J y Garza-Ortega S. 2010. Sandía (Citrullus lanatus (Thunb.) Matsum. & Nakai) injertada sobre diferentes portainjertos de calabaza (Cucurbita maxima x Cucurbita moschata). Biotecnia. XII: 3-10. Disponible en línea: https://biotecnia.unison.mx/index.php/biotecnia/article/view/87 [ Links ]
López-Mondéjar R, Beaulieu R, Ros M and Pascual JA. 2012. SCAR-based real-time TaqMan PCR for early detection of Fusarium oxysporum in melon seedlings under greenhouse nursery conditions. Crop protection 33:1-6. https://doi.org/10.1016/j.cropro.2011.10.009 [ Links ]
Marek SM, Yaghmour MA and Bostock RM. 2013. Fusarium spp., Cylindrocarpon spp., and environmental stress in the etiology of a canker disease of cold-stored fruit and nut tree seedlings in California. Plant Disease 97: 259-270. http://dx.doi.org/10.1094/PDIS-04-12-0355-RE [ Links ]
Martyn RD and McLaughlin RJ. 1983. Effects of inoculum concentration on the apparent resistance of watermelons to Fusarium oxysporum f. sp. niveum. Plant Disease 67:493-495. Disponible en línea: https://www.apsnet.org/publications/PlantDisease/BackIssues/Documents/1983Articles/PlantDisease67n05_493.PDF [ Links ]
Meza-Möller AC, Rentería-Martínez ME, Guerra-Camacho M, Romo-Tamayo F, Ochoa-Meza A, Moreno-Salazar SF. 2014. First report of root rot of watermelon caused by Ceratobasidium sp. in Sonora, Mexico. Plant Disease 98:847. https://doi.org/10.1094/PDIS-09-13-0974-PDN [ Links ]
Mordue JEM, Currah RS and Bridge PD. 1989. An integrated approach to Rhizoctonia taxonomy: cultural, biochemical and numerical techniques. Micological Research 92:78-90. https://doi.org/10.1016/S0953-7562(89)80099-1 [ Links ]
Narayanasamy P. 2011. Assessment of variability in fungal plant pathogens. Pp:245-272 In: Microbial plant pathogens detection and disease diagnosis. Vol. 1. Springer. Dordrecht, Netherlands. 291p. https://doi.org/10.1007/978-90-481-9735-4_4 [ Links ]
Nischwitz C, Chitrampalam P and Olsen M. 2013. Ceratobasidium root rot: A new disease of watermelon (Citrullus lanatus) in Arizona. Plant Health Progress. DOI:10.1094/PHP-2013-1125-01-BR [ Links ]
O’Donnell K, Kistler HC, Cigelnik E and Ploetz RC. 1998. Multiple evolutionary origins of the fungus causing Panama disease of banana: Concordant evidence from nuclear and mitochondrial gene genealogies. Proceedings of the National Academy of Sciences of the United States of America 95:2044-2049. Disponible en línea: https://www.ncbi.nlm.nih.gov/pmc/articles/PMC19243/ [ Links ]
O’Donnell K, Rooney AP, Proctor RH, Brown DW, McCormick SP, Ward TJ, Frandsen RJ N, Lysoe E, Rehner SA, Aoki T, Robert VARG, Crous PW, Groenewald JZ, Kang S and Geiser DM. 2013. Phylogenetic analysis of RPB1 and RPB2 support a middle Cretaceous origin for a clade comprising all agriculturally and medically important fusaria. Fungal Genetics and Biology 52:20-31. https://doi.org/10.1016/j.fgb.2012.12.004 [ Links ]
O’Donnell K, Ward TJ, Robert VARG, Crous PW, Geiser DM and Kang, S. 2015. DNA sequence-based identification of Fusarium: current status and future directions. Phytoparasitica 43:583-595. https://doi.org/10.1007/s12600-015-0484-z [ Links ]
Padwick GW. 1945. Notes on Indian fungi III. Mycological Papers. 12:1-15 [ Links ]
Posada D and Crandall KA. 1998. MODELTEST: testing the model of DNA substitution. Bioinformatics 14:817-818. https://doi.org/10.1093/bioinformatics/14.9.817 [ Links ]
Rentería-Martínez ME, Meza-Moller AC, Guerra-Camacho M, Romo-Tamayo F, Ochoa-Meza A and Moreno-Salazar SF. 2015. First report of watermelon wilting caused by Fusarium brachygibbosum in Sonora, Mexico. Plant Disease 99:729. https://doi.org/10.1094/PDIS-10-14-1073-PDN [ Links ]
SAGARPA, Secretaría de Agricultura, Ganadería, Desarrollo Rural, Pesca y Alimentación. 2014. Servicio de información agroalimentaria y pesquera. [2015-09-10]. http://www.siap.gob.mx/index [ Links ]
Saroj A, Kumar A, Saeed ST, Samad A and Alam M. 2013. First report of Tagetes erecta damping off caused by Ceratobasidium sp. from India. Plant Disease 97:1251. http://dx.doi.org/10.1094/PDIS-02-13-0145-PDN [ Links ]
Shan LY, Cui WY, Zhang DD, Zhang J, Ma NN, Bao YM, Dai XF and Guo W. 2017. First report of Fusarium brachygibbosum causing maize stalk rot in China. Plant Disease 101:837. https://doi.org/10.1094/PDIS-10-16-1465-PDN [ Links ]
Sharon M, Freeman S, Kuninaga S. and Sneh B. 2007. Genetic diversity, anastomosis groups and virulence of Rhizoctonia spp. from strawberry. European Journal of Plant Pathology 117:247-265. https://doi.org/10.1007/s10658-006-9091-7 [ Links ]
Sharon M, Kuninaga S, Hyakumachi M, Naito S and Sneh B. 2008. Classification of Rhizoctonia spp. using rDNA-ITS sequence analysis supports the genetic basis of the classical anastomosis grouping. Mycoscience 49:93-114. https://doi.org/10.1007/s10267-007-0394-0 [ Links ]
SIAP . Servicio de Información Agroalimentaria y Pesquera. Producción agrícola por cultivo. [2017-02-14]. http://www.siap.gob.mx/. [ Links ]
Sievers F, Wilm A, Dinee D, Gibson TJ, Karplus K, Li W, Lopez R, McWilliam H, Remmert M, Söding J, Thompson JD and Higgins DG. 2011. Fast, scalable generation of high-quality protein multiple sequence alignments using Clustal Omega. Molecular Systems Biology 7:539. https://doi.org/10.1038/msb.2011.75 [ Links ]
Sousa ES, Melo MP, Mota JM, Sousa EMJ, Beserra JEA and Matos KS. 2017. First report of Fusarium falciforme (FSSC 3 + 4) causing root rot in lima bean (Phaseolus lunatus L.) in Brazil. Plant Disease 101:1954. https://doi.org/10.1094/PDIS-05-17-0657-PDN [ Links ]
Sneh B, Jabaji-Hare S, Neate S, and Dijst G. 1996. Rhizoctonia Species: Taxonomy, Molecular Biology, Ecology, Pathology and Disease Control. Kluwer Academic Publishers Dordrecht, Netherlands. 578p. [ Links ]
Stack AJ, Yaghmour MA, Kirkpatrick SC, Gordon TR and Bostock RM. 2017. First report of Fusarium brachygibbosum causing cankers in cold-stored, bare-root propagated almond trees in California. Plant Disease 101:390. https://doi.org/10.1094/PDIS-06-16-0929-PDN [ Links ]
Stielow JB, Lévesque CA, Seifert KA, Meyer W, Iriny L, Smits D and Robert V. 2015. One fungus, which genes? Development and assessment of universal primers for potential secondary fungal DNA barcodes.Persoonia: Molecular Phylogeny and Evolution of Fungi 35:242-263. http://doi.org/10.3767/003158515X689135 [ Links ]
Swofford DL. 2002. PAUP*. Phylogenetic Analysis Using Parsimony (*and Other Methods). Version 4. Sinauer Associates, Sunderland, Massachusetts. Disponble en línea: http://paup.scs.fsu.edu/Cmd_ref_v2.pdf [ Links ]
Taylor JW, Jacobson DJ, Kroken S, Kasuga T, Geiser DM , Hibbett DS and Fisher MC. 2000. Phylogenetic species recognition and species concept in fungi. Fungal Genetics Biology 31:21-32. https://doi.org/10.1006/fgbi.2000.1228 [ Links ]
Trabelsi R, Sellami H, Gharbi Y, Krid S, Cheffi M, Kammoun S, Dammak M, Mseddi A, Gdoura R and Ali TM. 2017. Morphological and molecular characterization of Fusarium spp. associated with olive trees dieback in Tunisia. 3 Biotech 7:28. https://doi.org/10.1007/s13205-016-0587-3 [ Links ]
Wang PP and Wu XH. 2012. First report of sugar beet seedling damping-off caused by binucleate Rhizoctonia AG-A in China. Plant Disease 96:1696. https://doi.org/10.1094/PDIS-05-12-0492-PDN [ Links ]
White TJ, Bruns T, Lee S and Taylor J.1990. Amplification and direct sequencing of fungal ribosomal RNA genes for phylogenetics. In: Innis MA, Gelfand DH, Sninsky JJ and White TJ (eds). PCR Protocols: A Guide to Methods and Applications. Academic Press. San Diego, CA, USA.345p. [ Links ]
Received: October 05, 2017; Accepted: December 31, 2017