1. INTRODUCTION
White dwarfs (WDs) are the last stage of evolution for most stars. Probing their internal structures provides us with important insights on the complex evolutionary paths that led up to this stage. Furthermore, evolution is still ongoing in the WD stage, where it is primarily dominated by cooling (e.g. Salaris et al. 2013). WD interiors are characterized by stratified layers of different masses which mix and settle at certain temperatures. Therefore, as a WD cools down, its surface composition changes (e.g. Tremblay et al. 2013). Consequently, in the different phases (or temperature ranges) of WD evolution, one encounters WDs with H-dominated atmospheres (DAs) and non-DAs (He-atmosphere DOs and DBs).
On its evolutionary path, a WD crosses several instability strips, which are determined by atmospheric composition and total mass. The resulting pulsational variability opens up possibilities to probe the internal structure; for instance, Romero et al. (2014) demonstrated that nonradial gravity mode (g-mode) pulsations allow the study of the stellar interior via the technique of asteroseismology.
Asteroseismology of WDs gained momentum with the work of Winget et al. (1994), who derived precise stellar parameters for the DBV GD 358 from more than 180 significant peaks in its frequency spectrum. The corresponding group of variable stars was later designated V777 Her objects, which pulsate in g-modes with periods between 100 and 1200s (Beauchamp et al. 1999). Their cooler cousins, the ZZ Ceti stars, consist of pulsating H-rich DAV WDs and exhibit variability with periods between 70 and 2000s (Romero et al. 2019). During the last two decades, ground-based and satellite observations of WDs have significantly widened our knowledge in this field of study (Kepler et al. 2005; Hermes et al. 2014) by discovering and analysing many pulsating WDs.
However, several important questions about the pulsational behaviour of WDs remain: What exactly defines the borders of the instability strip? Do all WDs situated inside the instability strip pulsate? If not, what are the important astrophysical parameters?
In this paper, we present high-quality ground-based time-series of twenty-one WDs, including one hot Herich DO, with V magnitudes between 13.7 and 16.7mag. These objects are too faint for most current automatic ground-based surveys and at the limit of observability even for satellite-based missions such as Kepler (Doyle et al. 2017). Among the first published results from the TESS mission was an investigation of the known pulsating DBV WD 0158−160 (Bell et al. 2019). With a main period of about 640s and an amplitude of about 22mmag, this object is quite typical among pulsating WDs. Generally, TESS data are of limited use in this field of study because of the employed observing cadences (2 and 30min), the faintness of most WDs, and the expected amplitudes.
2. TARGET SELECTION, OBSERVATIONS ANDREDUCTION
We selected interesting WDs situated within or close to the heuristically established instability strip (Althaus et al. 2010). Several He-rich and He-weak objects were included to cover different atmospheric characteristics. In addition, our sample covers a wide range of ages. The astrophysical parameters of our target stars from the literature are listed in Table 1.
TABLE 1 TARGET STARS AND THEIR ASTROPHYSICAL PARAMETERS FROM THE LITERATURE
WD |
|
|
log H/He |
|
Ref |
---|---|---|---|---|---|
[K] | [dex] | ||||
0002+729 | 14 410 | 8.27 | ⎼5.95 | 8.52 | 4 |
0017+136 | 18 130 | 8.08 | ⎼4.63 | 8.07 | 4 |
0038+555 | 8 860 | 8.00 | 8.96 | 2 | |
0231+570 | 13 790 | 8.03 | 8.43 | 2 | |
0449+252 | 11 520 | 8.00 | 8.62 | 2 | |
0454+620 | 10 960 | 8.88 | 9.30 | 2 | |
0713+399 | 65 000 | 7.50 | 5 | ||
0835+340 | 22 230 | 8.25 | < ⎼ 4.63 | 7.90 | 4 |
0921+091 | 19 470 | 8.01 | ⎼4.72 | 7.90 | 4 |
0949+094 | 18 694 | 8.09 | ⎼4.52 | 3 | |
1052+273 | 23 930 | 8.41 | 7.96 | 2 | |
1107+265 | 15 130 | 8.11 | ⎼5.77 | 8.35 | 4 |
1149-133 | 20 370 | 8.30 | ⎼3.77 | 8.08 | 4 |
1326-037 | 19 950 | 8.03 | < ⎼4.81 | 7.87 | 4 |
1540+680 | 22 240 | 7.96 | < ⎼4.43 | 7.58 | 4 |
1612-111 | 23 430 | 7.96 | < ⎼4.75 | 7.46 | 4 |
1727+560 | 12 500 | 8.00 | ⎼3.70 | 1 | |
2147+280 | 12 940 | 8.86 | ⎼5.74 | 9.08 | 4 |
2234+064 | 23 770 | 8.07 | < ⎼4.72 | 7.56 | 4 |
2246+120 | 26 840 | 7.92 | < ⎼4.30 | 7.13 | 4 |
2250+746 | 16 560 | 8.15 | < ⎼6.18 | 8.26 | 4 |
1. Wegner & Koester (1985), 2. Limoges et al. (2015), 3. Koester & Kepler (2015), 4. Rolland et al. (2018), 5. Werner et al. (2018)
Photometric observations were performed at the following four sites using the described telescopes and instruments:
Hvar Observatory, University of Zagreb, 1.0m, Austrian-Croatian Telescope (ACT), Apogee Alta U47 CCD camera, integration time 15s for all targets, no filter,
Institute of Astronomy of the University of Vienna, 0.8m, “vienna little telescope” (vlt), SBIG STL6303E CCD camera, integration time 20 to 30s, no filter,
McDonald Observatory (McD), 2.1m, Argos, integration time 10 to 25s for all targets, BG40 filter,
Mt. Suhora Observatory (MSO), Poland, 0.6m, Zeiss telescope, Apogee Alta U47 CCD, integration time 30s for all targets, no filter,
South African Astronomical Observatory (SAAO), 0.75m, high-speed CCD photometer, integration time 5 to 10s, no filter.
Data reduction and differential photometry were performed using three different program packages:
Image Reduction and Analysis Facility (IRAF, Stetson 1987),
Munipack (Chrastina & Hroch 2008),
Multiple object and multiple frame CCD photometry (MOMF, Kjeldsen & Frandsen 1992).
For IRAF and Munipack, classical aperture photometry was applied; the MOMF package combines this with point spread function photometry. None of our target fields were very crowded, but the resulting noise level from each program package was tested. Time-series analysis of the differential light curves was conducted applying a standard Fourier technique and the PhaseDispersion Method. All computations were done within the programme package Peranso (Paunzen & Vanmunster 2016). To account for different seeing conditions, several apertures were used and tested. For each data set, we chose the aperture which yielded the smallest scatter in the differential light curves. For all objects, several comparison stars were available, which were checked individually to exclude variable objects.
First, the upper limits of variability of the WDs were compared, which yielded the same overall noise level of ±0.2mmag for all three methods. No trend of the noise level with frequency was detected. Furthermore, we checked the multiperiodic solution for WD 2246+120. Here, usage of the MOMF package resulted in a slightly better detection probability than provided by the other two packages.
The observation log and results from the time-series analysis are shown in Table 2. In total, we analysed 10335 frames spanning 4103 minutes of observations. All images and data are available on request from the first author.
TABLE 2 OBSERVATION LOG AND RESULTS FROM THE TIME-SERIES ANALYSIS
WD | Other | V | HJD(start) | ∆t | N | Site | UL | Freq | Amp |
---|---|---|---|---|---|---|---|---|---|
[mag] | [2450000+] | [min] | [mmag] | [mHz] | [mmag] | ||||
0002+729 | GD 408 | 14.3 | 8806.221126 | 296.6 | 517 | MSO | 1.2 | ||
0017+136 | Feige 4 | 15.4 | 5418.541842 | 71.0 | 246 | ACT | 4.3 | ||
0038+555 | EGGR 245 | 14.1 | 8807.286154 | 108.8 | 206 | MSO | 3.0 | ||
0231+570 | GD 283 | 13.7 | 8823.262619 | 305.6 | 557 | MSO | 0.8 | ||
0449+252 | PM J04523+2519 | 14.9 | 8806.437791 | 189.3 | 335 | MSO | 1.7 | ||
8824.394303 | 176.1 | 312 | MSO | 1.8 | |||||
0454+620 | PM J04586+6209 | 14.5 | 8823.500997 | 304.6 | 551 | MSO | 1.0 | ||
0713+399 | HS 0713+3958 | 16.2 | 2262.816274 | 27.0 | 99 | McD | 3.4 | ||
2263.795979 | 99.5 | 198 | McD | 1.9 | |||||
2266.794590 | 99.5 | 198 | McD | 2.4 | |||||
2267.801534 | 89.5 | 180 | McD | 2.3 | |||||
2268.811951 | 69.5 | 140 | McD | 2.1 | |||||
0835+340 | CSO 197 | 16.0 | 4560.591748 | 57.0 | 323 | McD | 3.6 | ||
0921+091 | PG 0921+092 | 16.2 | 4555.278322 | 107.7 | 228 | vlt | 6.6 | ||
4557.278634 | 89.7 | 190 | vlt | 7.2 | |||||
4563.582199 | 55.5 | 310 | McD | 1.7 | |||||
0949+094 | PG 0949+094 | 16.0 | 4205.309769 | 163.3 | 300 | vlt | 3.6 | ||
4206.336458 | 124.8 | 224 | vlt | 4.4 | |||||
4562.600301 | 56.2 | 286 | McD | 1.8 | |||||
1052+273 | GD 125 | 14.2 | 8824.524161 | 251.9 | 425 | MSO | 1.4 | ||
1107+265 | GD 128 | 15.9 | 4202.345671 | 108.2 | 194 | vlt | 3.7 | ||
4203.310475 | 161.0 | 307 | vlt | 3.4 | |||||
1149−133 | HE 1149−1320 | 16.1 | 3792.577824 | 97.2 | 356 | SAAO | 4.6 | ||
1326−037 | PG 1326−037 | 15.7 | 3794.604931 | 63.4 | 529 | SAAO | 5.7 | ||
3795.595417 | 74.7 | 428 | SAAO | 1.3 | |||||
1540+680 | PG 1540+681 | 16.2 | 5419.310115 | 94.4 | 249 | ACT | 3.5 | ||
1612−111 | GD 198 | 15.5 | 3790.591169 | 75.2 | 423 | SAAO | 2.8 | ||
1727+560 | G 227−5 | 16.1 | 5417.399217 | 84.5 | 269 | ACT | 1.9 | ||
2147+280 | EGGR 583 | 14.7 | 8824.189224 | 209.3 | 369 | MSO | 3.1 | ||
2234+064 | PG 2234+064 | 16.0 | 5418.463723 | 104.7 | 369 | ACT | 2.9 | ||
2246+120 | V393 Peg | 16.7 | 5417.477938 | 198.6 | 699 | ACT | 3.05 | 15.8 | |
2250+746 | GD 554 | 16.7 | 5421.460083 | 88.0 | 318 | ACT | 2.6 |
3. RESULTS
No new pulsating WDs were identified among our sample of twenty-one WDs; however, we have derived upper limits of variability that are also important input parameters for the test and development of pulsation models.
The results of the time-series analysis are shown in Figures 1 and 2. Defining the upper limit of variability is not straightforward and has often been discussed in the literature (Reegen et al. 2008). In general, the statistical significance of the noise in the Fourier spectrum is underestimated. We here employ a conservative approach and define the upper limit of variability as the upper envelope of the peaks in an amplitude spectrum (Figures 1 and 2). None of the detected amplitudes exceed a significance of 1.3σ of the noise level in the corresponding spectrum. Typically, we achieved an upper limit for these targets of a few mmag. In the following, information on the individual stars is provided.
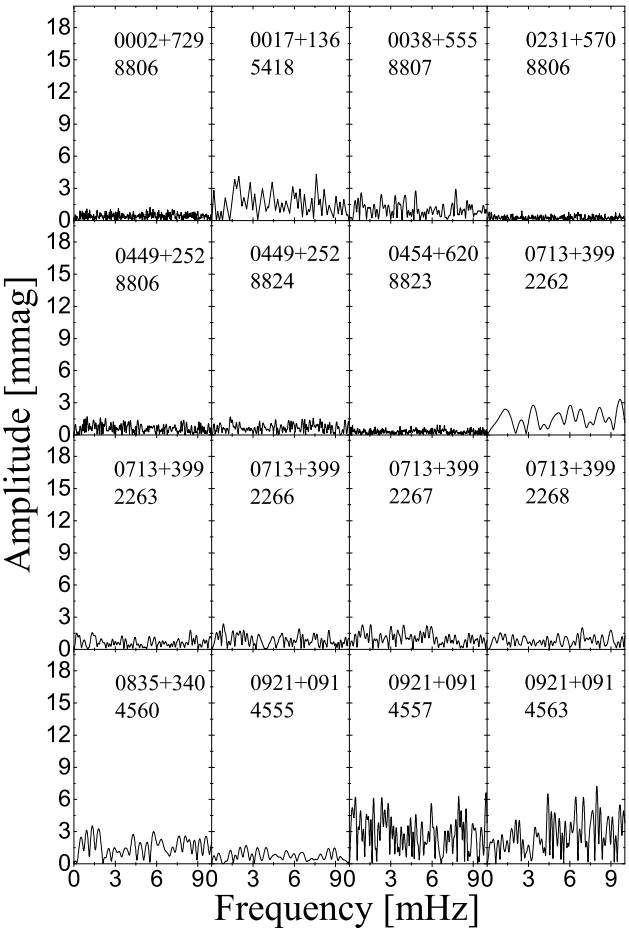
Fig. 1 Fourier spectra of our target stars. Each panel lists the WD number and the JD 2450000+ of the observing night.
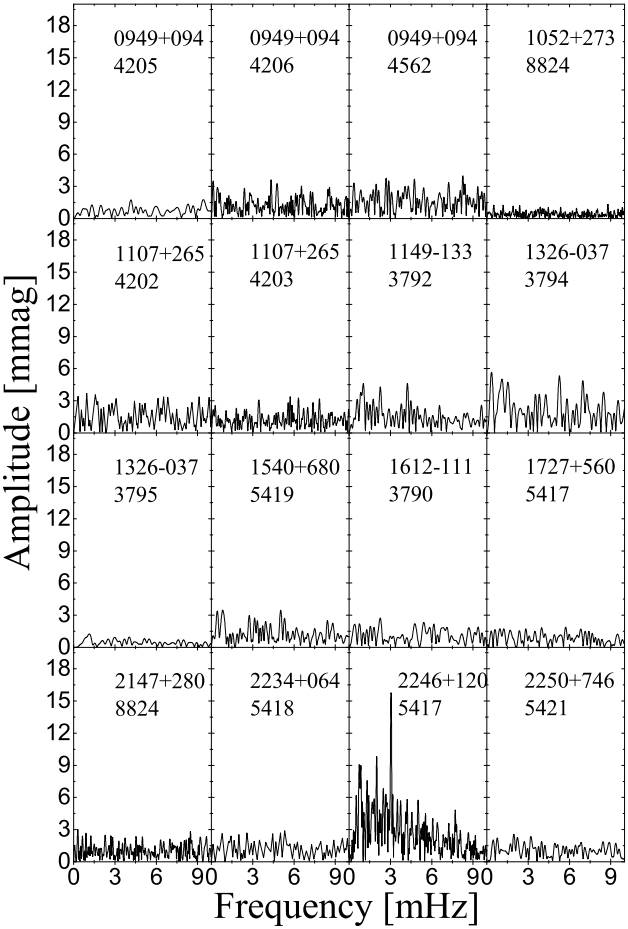
Fig. 2 Fourier spectra of our target stars. Each panel lists the WD number and the JD 2450000+ of the observing night. WD 2246+120 is a known pulsator immediately noticeable from its rich frequency spectrum.
WD 0002+729: This is a carbon-poor and metal-rich WD that does not show any signs of a surrounding dust disk (Farihi et al. 2009). The origin of its peculiar abundance pattern is still not well understood.
WD 0017+136:Limoges et al. (2015) published a parallax-based distance of 33.3pc from the Sun for this 0.65M object. They concluded that it is one of the seven DBs known to be closer than 40pc. However, the spectroscopy-based distance of 75pc (Bergeron et al. 2011) does not agree with that, which could be caused by (hitherto undetected) binarity. Bailer-Jones et al. (2018) published a distance of 78.9pc on the basis of Gaia DR2 (Lindegren et al. 2018). In the latter work, WD 0017+136 is not flagged as a binary. Gaia DR3, which is scheduled for 2021, is going to include a list of binaries, which might finally solve this issue.
WD 0038+555:Marinoni et al. (2016) included this object in their photometric short-term variability monitoring survey, which formed a part of the Gaia spectrophotometric standard star calibration. They derived an upper limit of variability of 2mmag in Johnson B and, within their criteria, accepted WD 0038+555 as a constant star. Our results are in line with this study.
WD 0231+570: This is one of the UBVRI photoelectric standard stars of Landolt (2013), which were observed within a period of 17 years at the Kitt Peak National and Lowell Observatories. Since no individual errors are given by the authors, an independent check of variability on short timescales is important. We here derive an upper limit of 0.8mmag for this star.
WD 0449+252 and WD 0454+620: Both stars are included in the paper of Bógnar et al. (2018), who searched´ for new WD pulsators for observations by the TESS space telescope. The authors reported no signs of shortterm variability.
WD 0713+399: This DO star is remarkable in several respects. Werner et al. (1995) detected unusually strong He II absorption lines that could not be reproduced by a simple DO model atmosphere. They speculated that the star may belong to a new subgroup of hot He-rich WDs. Later, Dreizler & Werner (1996) investigated an up-todate list of these WDs and concluded that they represent the non-DA cooling sequence from the hot end down to the DB gap. The observed mean mass value of about 0.6M coincides very well with those of DA and DB WDs.
WD 0835+340:Wagner et al. (1986) were the first to classify this star as a DB3, which was later confirmed by several other studies. Furthermore, Girven et al. (2011) did not find any IR-excess for this object, which implies that no low-mass stellar companion or dusty debris disc is present around it.
WD 0921+091: This star has a very low total hydrogen mass (
WD 0949+094:Castanheira et al. (2006) presented 1.25h (193 data points) of photometric observations of this star. However, they did not discover any significant signals in the observed Fourier spectrum (noise of about 6mmag). Our limits of variability range between 1.6 and 4.4mmag (Table 2).
WD 1052+273: Several studies unsuccessfully searched for a dust disk and an IR-excess around this rather bright WD (Wilson et al. 2019).
WD 1107+265: Several studies of this object are available in the literature (Bergeron et al. 2011). The log H/He value is very low (Table 1). Robinson &Winget (1983) derived upper limits of variability (semiamplitude) between 4.2 and 5.7mmag. Our results are compatible with these values.
WD 1149−133: Detailed analyses from the UV to the IR region are available in the literature for this Herich WD (Liebert et al. 1986). The results from our single observing run indicate a rather high upper limit of variability (4.6mmag). The star should be re-observed.
WD 1326−037: This WD is included as constant object in the database of synthetic photometry in the GALEX ultraviolet bands for the stellar sources observed with the International Ultraviolet Explorer (Beitia-Antero & Gómez de Castro 2016).
WD 1540+680:According to Beauchamp et al. (1999), this object is situated just on the red edge of the instability strip.
WD 1612−111: The search for a low-luminosity companion to this object yielded a null-result (Farihi et al. 2005).
WD 1727+560:The spectrum of this massive DQ WD contains strong emission features of O I and C II (Provencal et al. 2005). Unexpectedly, as compared with carbon, oxygen is overabundant by a factor of about 160 in the chromosphere. In summary, WD 1727+560 is an outstanding object for which we have not detected any signs of variability.
WD 2147+280: With an age of more than 1Gyr, this star is one of the oldest objects in our sample.
WD 2234+064: This is one of the rare WDs that show a weak Ca II K-line of interstellar origin (Zuckerman et al. 2010).
WD 2246+120: In order to check the reliability of our observational strategy and reduction techniques, we observed this known variable star, which is a pulsating He-atmosphere DB (Handler 2001). It shows variability with periods between 255 and 300s. The periods and amplitudes derived in the present study are in excellent agreement with the literature values, which lends confidence to our analysis.
WD 2250+746:Robinson & Winget (1983) derived upper limits of variability (semi-amplitude) between 3.0 and 7.1mmag, which is comparable to our results.
We have compared our derived upper limits of variability (column “UL” in Table 2) with the extensive surveys aimed at the detection of pulsating WDs by Mukadam et al. (2004), Mullally et al. (2005) and Castanheira et al. (2010). These authors also used CCD observations with comparable time bases and integration times. The observations by Mukadam et al. (2004) and Mullally et al. (2005) were done with the same equipment we employed at the McDonald Observatory, whereas Castanheira et al. (2010) used the 4.1m SOAR telescope. The limit for non-variability (NOV) was established using a classical Fourier transformation technique defining the highest white-noise peak as the detection threshold. The latter is given in the unit of millimodulation amplitude (mma), which corresponds to a 0.1% change in intensity. Typically, NOV limits between 2 and 8mma were reached. This is well within the range of our results. Castanheira et al. (2010) re-observed stars with high NOV limits and detected new variables with amplitudes below the NOV limits among them. Therefore, we caution that there might well be some undetected low-amplitude variables among the WDs of our sample.
4. CONCLUSIONS
The two primary temperature regimes in which isolated cooling WDs are observed to pulsate are T eff ≈12000K for the DAVs, and T eff ≈25000K for the DBVs. Pulsations are driven in a subsurface partial ionization zone of the dominant surface chemical element, have periods between 70 and 2000s, and are composed of nonradial gravity modes.
Among the hotter WDs, we find the GW Vir objects with T eff between 75000 K and 200000K and periods between 300 and 5000s. Here, the pulsations are driven by ionized carbon and oxygen.
Pulsating WDs provide an important glimpse into the future of the vast majority of stars and their interiors. With asteroseismic tools, it is possible to trace the stellar structure down to the core.
For modeling efforts, it is not only important to analyse the pulsational characteristics of variable WDs but also apparently non-variable objects in the same region of the Hertzsprung-Russell diagram. The search for (dis)similarities between pulsators and non-pulsators can significantly improve the framework of astrophysical parameters in which pulsation occurs.
In this work, we presented high-quality photometric time-series observations of twenty-one WDs from five different sites. To cover interesting regions of the instability strip, our sample encompassed He-rich and Heweak objects. Although we have not detected any new pulsating WD, the derived upper limits of variability are important input parameters for pulsation models.