Introduction
There is a trend towards diversifying the production of edible mushrooms in the world, although in Europe and in the Americas, the most cultivated fungus by far is currently Agaricus bisporus (J.E. Lange) Imbach. This species of mushroom represents approximately 90 % of the total production of edible mushrooms in most countries (Sánchez et al., 2018).
Agaricus bisporus is commercially cultivated on organic materials such as wheat straw, manure, and corncob, requiring an important treatment including composting and pasteurization to produce a selective substrate that promotes the mycelial growth and fruiting of mushrooms (Song et al., 2014).
The composting process occurs naturally as a consequence of the microbial activity on the wet raw material and is considered essential to develop the final quality of the compost (Souza et al., 2014). Due to the microbial metabolism of sugars, the temperature inside the compost increases, and the mesophilic microbiota is replaced by the thermophilic microbiota. The development of certain species of bacteria and fungi are of great interest not only for the conversion of organic material but also for the excretion of a wide range of metabolites with growth regulation activities (Vijay and Pathak, 2014). These organisms may have metabolic functions such as siderophore production, the ability to solubilize phosphate, hormone production, antibiotic production, nitrogen fixation or the ability to synthesize or degrade metabolites such as 1-octen-3-ol that affect the processes of mushroom development and differentiation. Addition of beneficial organisms in the substrate may be a biotechnological alternative to optimize the development of commercial production of A. bisporus (Sánchez and Royse, 2017). A well-colonized substrate may increase mushroom yield with more fruiting bodies during flushes (Kabel et al., 2017; Aguilar Pontesa et al., 2018). However vigorous mycelial growth is no guarantee of early formation and mushroom production. Often the exact opposite is true depending on the C/N ratio and microorganisms on the cover layer and on the cultivation substrate (Ferreira et al., 2009).
The compost in which mushroom is traditionally cultivated has been reported as a suitable habitat for the development of thermophilic fungi, among them Mycothermus thermophilus (Cooney & R. Emers.) X. Wei Wang, Houbraken & D.O. Natvig (synonym of Scytalidium thermophilum), Malbranchea cinnamomea (Lib.) Oorschot & de Hoog (synonym Malbranchea sulfurea) and Myriococcum thermophilum (Fergus) Aa, which stimulate the mycelial growth and production of A. bisporus (Straatsma et al., 1991, 1994; Sánchez, 2007; Sánchez et al., 2008) by reducing the concentration of ammonium, immobilizing nutrients, and producing metabolites that can inhibit competing organisms. After the thermophilic fungi colonize the substrate at 45-50 °C, the substrate is cooled down (Zhang et al., 2019) and thermophiles diminish in activity or die and leave as residues their cell membranes, which are composed of polysaccharides, fatty acids such as linoleic acid and proteins that constitute a rich reserve for A. bisporus (Fermor and Wood, 1991).
Alternative methods for substrate preparation without the generation of odor have been studied as an improvement of the traditional method of cultivation with two phases for the white button mushroom (Till, 1962; Sánchez and Royse, 2001; Bechara et al., 2005). Self-heating pasteurization method is an alternative to prepare and pasteurize the substrate in only 45 h in order to obtain biological efficiency values superior to 100 % on A. bisporus cultivation (Colmenares et al., 2017; Morales and Sánchez, 2017). This is an important advantage because it allows similar mushroom yields with a decrease of the processing time compared to the traditional method that takes between 10 and 21 days (Samp, 2007). Therefore, the objective of this work was to isolate, select and identify thermophilic microorganisms in self-heating pasteurized raw material and determine potential promoting effects of those microorganisms on A. bisporus mycelial growth.
Materials and methods
Microorganisms
Commercial spawn of A. bisporus var. portobello Heirloom cultivated on millet grain was purchased from Amycel (San Miguel de Allende, Guanajuato, Mexico). The thermophilic microbiota used in this study was isolated directly from batches of pangola grass (Digitaria eriantha Steud) in the pasteurization process and in the mycelial growth of A. bisporus and preserved in the Mycological Collection of El Colegio de la Frontera Sur (Ecosur), Mexico. The acronym ECS used to name all strains stands for Ecosur.
Self-Heating Pasteurization
For pasteurizing pangola grass, Avendaño and Sánchez (2013) and Morales and Sánchez (2017) methods were followed. These methods consisted of the use of a 1 m3 wooden box covered with a 2 cm polyurethane layer and with 50 holes evenly distributed at the bottom of the crate to provide aeration to the raw material. This crate also had a hole in the front in each of three levels of the drawer: 15 cm from the surface (L1), to the center (L2) and 15 cm above the bottom (L3), to allow temperature readings with bimetallic thermometers 30 cm long. Three pasteurization trials were performed using 90 kg of dry raw material mixed with 2 % calcium hydroxide and humidity adjusted to 65 %. It lasted 45 h with manual turning of the substrate after 30 h of processing.
Isolation and physiological features
The isolation of bacteria and fungi was carried out by serial dilutions. Samples of pangola grass were collected from three different points (Levels 1, 2 and 3) of a batch in the process of self-heating pasteurization to ultimately form a composite sample of 10 g. This sample was suspended in 90 mL of saline solution and stirred for 15 min. Dilutions to 10-6 were prepared and 100 μL of these dilutions were inoculated by crossstreaking on solid nutrient agar (NA), Czapek agar and maltextract agar (MEA) plates to isolate a wide variety of microorganisms. The plates were incubated at two different temperatures (45 and 55 °C) until the appearance of individual colonies, which were selected according to their colonial morphology and subcultured until the confirmation of the purity of the cultures. Samples were taken at 0 h (B), 30 h (R), and 45 h of the pasteurization process and the last one also corresponds when the substrate was inoculated with A. bisporus spawning or 0 day (S), 7 days (1W), and 14 days (2W) of A. bisporus mycelial growth after spawning. Samples at 0 h were also the beginning of pasteurization process, at 30 h the moment of substrate turning, at 45 h the end of pasteurization. In addition, direct isolation of fungi was performed from the raw material during the pasteurization process by placing 5 g of pangola grass on the surface of MEA plates. Subsequently, the plates were incubated for 5 days at 45 or 55 °C until fungal colonies appeared.
Isolated microorganisms were characterized by Gram stain (bacteria only), micro- and macroscopically, and they were tested for the ability to produce sidero-phores (Schwyn and Neilands, 1987; Luna et al., 2013), grow on 1-octen-3-ol (Zarenejad et al., 2012) and solubilize phosphate (Sundara and Sincha, 1963).
Extraction of DNA and analysis of ribosomal region sequences
Isolated microorganisms showing positive effect on the mycelial growth of A. bisporus were selected for molecular identification. The selected bacterial strains were cultured in liquid nutritive medium and malt extract, and then the DNA was extracted with the Aqua-Pure Genomic DNA Kit (Bio-Rad Cat. No. 732-6340), following the manufacturer’s instructions, and 50 μL of DNA was obtained.
For the extraction of fungal DNA, the protocol described by Karthikeyan et al. (2010) was adapted. The lysis TES buffer was supplemented with PVP (polyvinyl-pyrrolidone) at 1 %, and digestion with RNase was carried out before precipitation with isopropanol:isoamyl alcohol. Subsequently, the DNA extracted from both bacteria and fungi was visualized by electrophoresis in a 0.8 % agarose gel.
One microliter of different dilutions (1:20 and 1:50) was used to perform the PCR using the ITS4 (5’ TCCTCCGCTTATTGATATGC 3’) and ITS5 (5’ GGAAGTAAAAGTCGTAACAAGG 3’) (White et al., 1990) primers for fungi and fD1 (direct) (5′ AGAGTTTGATCCTGGCTCAG 3′) and rD1 (reverse) (5′ AAGGAGGTGATCCAGCC3′) primers for bacteria (Weisburg et al., 1991) in a total reaction volume of 25 μL: 1X Buffer for PCR, 0.2 mM dNTPs, 0.2 pM each primer, 1.5 mM MgCl2 and 1U Taq polymerase. PCR conditions were as follows: an initial denaturation step at 94 °C for 5 min, followed by 40 cycles of 1 min at 95 °C, an annealing step for 30 s at 53 °C for bacteria or 1 min at 55 °C for fungi, followed by an extension step for 1 min at 72 °C and a final extension for 5 min at 72 °C.
PCR products were visualized by electrophoresis in a 1 % agarose gel. Once verified, the PCR products were purified by the Quantum Prep® PCR Kit Kleen Columns (Bio-Rad, Cat. No. 732-6300) and then stored at -20 °C until the products were sent to Macrogen Inc. (Seoul, Korea) for sequencing. The sequences were assembled and edited with MEGA7® software. Subsequently, they were analyzed using the BLAST tools in the GenBank databases (https://www.ncbi.nlm.nih.gov/) and KEGG (http://www.genome.jp/kegg/).
Effect of microorganism on Agaricus bisporus mycelial growth
Of the 106 isolates, 34 microorganisms were selected to evaluate their effect on the growth rate of A. bisporus in two ways: 1) according to Rainey (1991), in the center of a Petri dish containing PDA, an A. bisporus disc was inoculated, and after 4 days of incubation at 24 °C, each of the microorganisms evaluated was inoculated in triplicate on the surface of the culture medium at 20 mm of the edge of A. bisporus colony and 2) in glass tubes with substrate (Sánchez and Royse, 2001) (65 % moisture, at 24 °C) that was colonized with the microorganism being tested. A control was inoculated with A. bisporus only, without any other microorganism (Sánchez and Royse, 2001).
To obtain the bacterial inoculum, each strain was cultured in liquid medium (nutritive medium, Czapek or malt extract) at 45 or 55 °C for 24 h with constant agitation at 170 rpm. Subsequently, the cultures were centrifuged at 10,000 G for 15 min; the sediment was suspended in a retentive solution containing in g L-1: 0.09 NaCl, 3.35 Na2HPO4 and 2.1 KH2PO4. For fungal strains, 20 g pangola grass (65 % humidity) was placed on a plate and sterilized in an autoclave at 121 °C for 40 min. Then, the fungus being tested was inoculated on the grass and incubated at 45 °C until complete colonization. This substrate was aseptically introduced into sterile glass tubes containing 5 grains of A. bisporus inoculum. The control in this case contained the substrate without fungus and with A. bisporus spawn. Moisture was adjusted with the retentive solution (Sánchez and Royse, 2001).
In both cases, (plates or tubes), measurements of the mycelial growth of A. bisporus were made every 5 days. In Petri dishes, these measurements were made at the bottom of the plate considering the radial growth. For the tubes, the measurements were made at three equidistant points around the circumference of the tube. The linear extension rate of the mycelial growth of A. bisporus (kr) was calculated by adjusting the linear growth function y=kr x + c (where y is the distance, and x is the time), which was expressed in mm/day.
Results
Evaluation of physicochemical parameters of the substrate during pasteurization
Figure 1 shows the evolution of the temperature and pH of the substrate during the 45 h of the self-heating pasteurization process. The temperature in the box with 90 kg (dry basis) of substrate reached values of 60 °C and higher after 20 h of processing in levels 1 and 2, and at the time of turning (30 h of processing), the substrate in these two levels had been maintained at this temperature for more than 9 h. At the time of turning, the batch of substrate located in level 3 (bottom) was placed in level 1 (upper part), with which it had a notable increase in temperature that led to a treatment of at least 6 h at 60 °C in the whole batch. With these results, it was considered that all of the material was pasteurized at the end of the process (45 h). In parallel, the pH value decreased from 9.1 to 6.8 during this time.
Isolation of microorganisms
The population of total cultivable microorganisms in the substrate ranged between 2.6x104 to 4.6x108 CFU g-1 at 45 °C (Figure 2) and 2.3x105 to 6.5x108 CFU g-1 at 55 °C (Figure 3), as determined from five measurements during the entire process (starting from the beginning of pasteurization until two weeks after substrate spawning).

Figure 2. Population of total microorganisms associated with the culture of A. bisporus on pangola grass isolated at 45 °C at different times of the pasteurization process and culture. B: beginning of pasteurization, R: turning of substrate, S: end of pasteurization (time of spawning A. bisporus), 1W: at 7 days of mycelial growth and 2W: at 14 days of mycelial growth. The vertical bars indicate the standard deviation of the means of three repetitions.
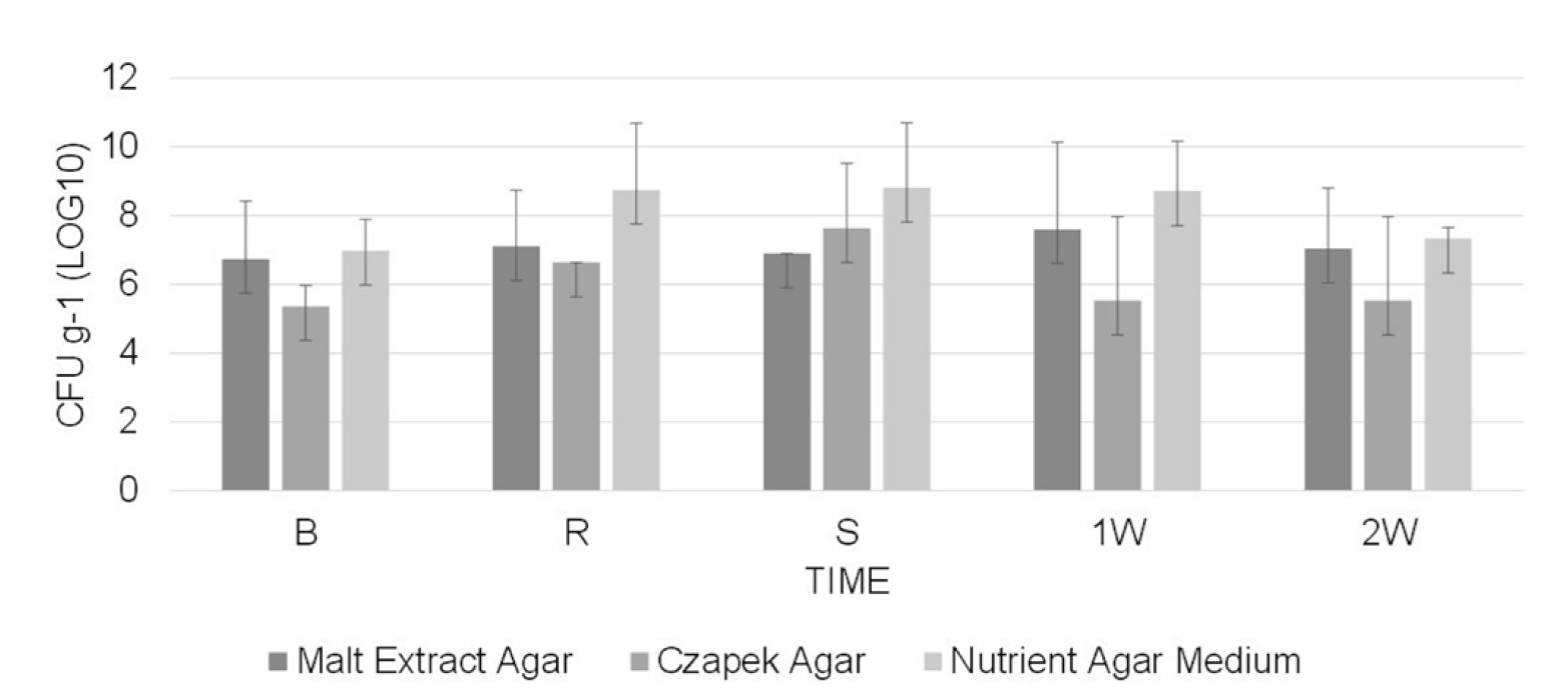
Figure 3. Population of total microorganisms associated with the culture of Agaricus bisporus on pangola grass isolated at 55 °C at different times of the pasteurization process and culture. B: beginning of pasteurization, R: turning of substrate, S: end of pasteurization (time of spawning A. bisporus), 1W: at 7 days of mycelial growth and 2W: at 14 days of mycelial growth. The vertical bars indicate the standard deviation of the means of three repetitions.
Based on their morphology and abundance, 57 and 49 microbial isolates were obtained at 45 and 55 °C respectively (Tables 1 and 2). Of these, 47 and 49 corresponded to bacteria at 45 and 55 °C respectively and 9 corresponded to fungi at 45 °C. No fungi were isolated at 55 °C. The 96 isolated bacteria were Gram positive. Regarding the shape of the bacterium colonies, punctate, granular, irregular and circular colonies were found, and circular shapes (70 % and 69 %) full edges (61 % and 55 %), creamy consistencies (58 % and 86 %) and convex colonies (60 % and 39 %) were the predominant morphologies at 45 and 55 °C, respectively.
Table 1. Culture characteristics of microorganisms isolated at 45 °C associated with different stages of cultivation of Agaricus bisporus on self-heating pasteurized substrate made on pangola grass
Time of isolation | Microorganismes | Gram | Characteristics of the colony | ||||||
Bacteria | Fungi | Colour | Form | Edge | Consistence | Elevation | |||
Removal of the substrate (R) | ECS-709 | White | Circular | Entire | Convex | Green spores |
|||
ECS-710 | Beige | Circular | Entire | Convex | |||||
ECS-B-54 | + | White | Circular | Entire | Creamy | Convex | |||
ECS-711 | White | Circular | Entire | Convex | Black spores |
||||
ECS-712 | Beige grayish | Circular | Entire | Convex | |||||
ECS-B-55 | + | Hyaline | Irregular | Undulated | Gelatinous | Pulvinate | |||
ECS-B-56 | + | Hyaline | Circular | Undulated | Gelatinous | Pulvinate | |||
Spawning of Agaricus bisporus (S) | ECS-B-57 | + | Yellow | Circular | Entire | Creamy | Convex | ||
ECS-B-58 | + | Yellow | Irregular | Undulated | Creamy | Pulvinate | |||
ECS-B-59 | + | Red | Circular | Undulated | Creamy | Pulvinate | |||
7 days after spawning Agaricus bisporus (1W) | ECS-713 | White | Circular | Entire | Convex | ||||
ECS-714 | Green | Circular | Entire | Convex | Green spores |
||||
ECS-715 | Green | Granulated | Entire | Convex | Whitespace periphery |
||||
ECS-B-60 | + | Yellow | Irregular | Undulated | Creamy | Pulvinate | |||
ECS-B-61 | + | Yellow | Irregular | Undulated | Gelatinous | Pulvinate | |||
14 days after spawning Agaricus bisporus (2W) | ECS-716 | White | Circular | Entire | Convex | Gray spores |
|||
ECS-717 | White | Circular | Entire | Convex | Brown spores |
||||
ECS-B-62 | + | Hyaline | Circular | Entire | Gelatinous | Convex | |||
ECS-B-63 | + | Hyaline | Irregular | Undulated | Gelatinous | Pulvinate |
Table 2. Culture characteristics of microorganisms isolated at 45 °C associated with different stages of cultivation of Agaricus bisporus on self-heating pasteurized substrate made on pangola grass
Time of isolation | Strains of bacterias | Gram | Characteristics of the colony | ||||
Colour | Form | Edge | Consistence | ELEVATION | |||
Removal of the substrate (R) | ECS-B-64 | + | Yellow | Circular | Entire | Creamy | Convex |
ECS-B-65 | + | White | Irregular | Undulated | Creamy | Pulvinate | |
ECS-B-66 | + | Hyaline | Circular | Undulated | Gelatinous | Pulvinate | |
Spawning of Agaricus bisporus (S) | ECS-B-67 | + | Yellow | Circular | Undulated | Creamy | Pulvinate |
ECS-B-68 | + | Hyaline | Circular | Entire | Creamy | Pulvinate | |
ECS-B-69 | + | Yellow | Circular | Entire | Creamy | Pulvinate | |
ECS-B-70 | + | Yellow | Circular | Entire | Creamy | Pulvinate | |
7 days after spawning Agaricus bisporus (1W) | ECS-B-71 | + | Yellow | Circular | Entire | Creamy | Convex |
ECS-B-72 | + | Yellow | Irregular | Undulated | Creamy | Pulvinate | |
ECS-B-73 | + | White | Irregular | Undulated | Creamy | Pulvinate | |
ECS-B-74 | + | White | Irregular | Undulated | Creamy | Pulvinate | |
ECS-B-75 | + | Yellow | Circular | Entire | Creamy | Convex | |
ECS-B-76 | + | White | Circular | Entire | Creamy | Convex | |
14 days after spawning Agaricus bisporus | ECS-B-77 | + | White | Irregular | Entire | Creamy | Pulvinate |
ECS-B-78 | + | Yellow | Irregular | Undulated | Creamy | Pulvinate |
Physiological features
All isolated microorganisms were evaluated for siderophore production, growth capacity in the presence of 1-octen-3-ol, and phosphate solubilization (Tables 3 and 4). Of the 106 isolates, only strain ECS-709 had the ability to produce siderophores, while 91 % were able to grow in the presence of 1-octen-3-ol, and 57 % had the ability to solubilize phosphate. From these results, 34 strains showing siderophore production, growth in 1-octen-3-ol or phosphate solubilization (19 and 15 microorganisms at 45 and 55 °C, respectively) were selected to evaluate the effect on the growth of A. bisporus. Regarding the effect of the selected microorganisms on the radial growth of A. bisporus on PDA plates, it was found that the fungal strains ECS-711 and ECS-712 presented a positive effect (α = 0.05), increasing the radial extension rate of A. bisporus by 106 and 208.8 %, while the rest of the fungi showed no effect. In contrast, 64 % of the bacteria showed an inhibitory effect, and 36 % of them showed no effect with respect to the control (Tables 3 and 4). In the growth tests with substrate in tubes, fungal strains ECS-709, ECS-710 and ECS-712 showed highly significant growth-promoting effects on A. bisporus (α = 0.05) with respect to the control that presented no growth. Most bacteria also presented a negative or no effect on the growth of A. bisporus on pangola grass, except for strains ECS-B-65 and ECS-B-78, which presented a fairly significant (α =0.01) promoting effect.
Table 3. Physiological features of microorganisms isolated at 45 °C associated with different stages of the cultivation of A. bisporus on self-heating pasteurized substrate made on pangola grass. Ability to grow in 1-octen-3-ol, production of siderophores, solubilization of phosphate, and effect on the growth of A. bisporus at 25 °C in PDA medium and in substrate
Microorganism | Radial extension rate of A. bisporus (MM/D) on potato dextrose agar | Linear extension rate of A. bisporus (MM/D) on pangola grass | |||||||
Time of isolation | Bacteria | Fungi | 1-octen -3-ol | Siderophores | Solubilization of phosphate | In the presence of the strain | Control | In the presence of the strain | Control |
Start of pasteurization (B) | ECS-709 | + | + | - | 0.343±0.08□ | 0.249±0.03 | 0.069±0.01*** | 0 | |
ECS-710 | + | - | - | 0.280±0.09□ | 0.249±0.03 | 0.085±0.02*** | 0 | ||
ECS-B-54 | + | - | - | 0.347±0.03□ | 0.423±0.07 | 0 | |||
Removal of the substrate (R) | ECS-711 | + | - | - | 0.513±0.04** | 0.249±0.03 | 0.054±0.04** | 0 | |
ECS-712 | + | - | - | 0.769±0.12*** | 0.249±0.03 | 0.077±0.01*** | 0 | ||
ECS-B-55 | + | - | - | 0.066±0.01*** | 0.423±0.07 | 0.073±0.03‡ | 0 | ||
ECS-B-56 | + | - | + | 0.452±0.03□ | 0.423±0.07 | 0 | |||
Spawning of Agaricus bisporus (S) | ECS-B-57 | + | - | - | 0.080±0.02*** | 0.423±0.07 | 0 | ||
ECS-B-58 | + | - | - | 0.077±0.02*** | 0.423±0.07 | 0 | |||
ECS-B-59 | + | - | + | 0.280±0.07□ | 0.423±0.07 | 0 | |||
7 days after the spawning of Agaricus bisporus (1W) | ECS-713 | + | - | - | 0 | 0.249±0.03 | 0.032±0.02□ | 0 | |
ECS-714 | + | - | - | 0.362±0.03□ | 0.249±0.03 | 0.045±0.02* | 0 | ||
ECS-715 | + | - | - | 0.433±0.07‡ | 0.249±0.03 | 0.059±0.01** | 0 | ||
ECS-B-60 | + | - | - | 0.200±0.04** | 0.423±0.07 | 0 | |||
ECS-B-61 | + | - | + | 0.200±0.02** | 0.423±0.07 | 0.097±0.00‡ | 0 | ||
14 days after the spawning of Agaricus bisporus (1W) | ECS-716 | + | - | - | 0.326±0.12□ | 0.249±0.03 | 0.051±0.005** | 0 | |
ECS-717 | - | - | - | 0.453±0.11‡ | 0.249±0.03 | 0.052±0.01** | 0 | ||
ECS-B-62 | + | - | - | 0.352±0.08□ | 0.423±0.07 | 0.046±0.00□ | 0 | ||
ECS-B-63 | + | - | + | 0.173±0.08*** | 0.423±0.07 | 0 |
Comparison of means between a treatment and its control; Meaning: ‘***’ 0.001 (highly significant differences) ‘**’ 0.01 (fairly significant) ‘*’ 0.05 (minor difference)’‡’ 0.1 (little significant difference) ‘□’ 1 (No significant difference) .
Table 4. Physiological features of microorganisms isolated at 55 °C associated with different stages of the cultivation of A. bisporus on self-heating pasteurized substrate made on pangola grass. Ability to grow in 1-octen-3-ol, production of siderophores, solubilization of phosphate, and effect on the growth of A. bisporus at 25 °C in PDA medium and in substrate
Radial extension rate of A. bisporus (MM/D) on potato dextrose agar | Linear extension rate of A. bisporus (MM/D) on pangola grass | |||||||
Time of isolation | Strain of Bacteria | 1-octen -3-ol | Siderophores | Solubilization of phosphate | In the presence of the strain | Control | In the presence of the strain | Control |
Removal of substrate (R) | ECS-B-64 | + | - | + | 0.405±0.008□ | 0.423±0.07 | 0.068±0.06□ | 0 |
ECS-B-65 | + | - | + | 0.451±0.02□ | 0.423±0.07 | 0.112±0.01** | 0 | |
ECS-B-66 | + | - | - | 0.374±0.06□ | 0.423±0.07 | 0.075±0.00□ | 0 | |
Spawning of Agaricus bisporus (S) | ECS-B-67 | + | - | + | 0.319±0.09□ | 0.423±0.07 | 0 | |
ECS-B-68 | + | - | - | 0.368±0.08□ | 0.423±0.07 | 0 | ||
ECS-B-69 | - | - | + | 0.157±0.02*** | 0.423±0.07 | 0 | ||
ECS-B-70 | - | - | + | 0.052±0.02*** | 0.423±0.07 | 0 | ||
7 days after spawning Agaricus bisporus (1W) | ECS-B-71 | - | - | - | 0.050±0.03*** | 0.423±0.07 | 0 | |
ECS-B-72 | - | - | - | 0.068±0.04*** | 0.423±0.07 | 0 | ||
ECS-B-73 | + | - | - | 0.115±0.02*** | 0.423±0.07 | 0.036±0.00□ | 0 | |
ECS-B-74 | - | - | + | 0.203±0.03** | 0.423±0.07 | 0 | ||
ECS-B-75 | + | - | + | 0.098±0.05*** | 0.423±0.07 | 0 | ||
ECS-B-76 | + | - | + | 0.151±0.08*** | 0.423±0.07 | 0 | ||
14 days after spawning Agaricus bisporus (2W) | ECS-B-77 | + | - | + | 0.283±0.12□ | 0.423±0.07 | 0 | |
ECS-B-78 | + | - | - | 0.031±0.006*** | 0.423±0.07 | 0.368±0.01** | 0 |
Comparison of means between a treatment and its control; Meaning: ‘***’ 0.001 (highly significant differences) ‘**’ 0.01 (fairly significant) ‘*’ 0.05 (minor difference)’‡’ 0.1 (little significant difference) ‘□’ 1 (No significant difference) .
Molecular identification of the isolates
The strains that showed a positive effect on the growth promotion of A. bisporus were analyzed phylogenetically by means of 16S (ECS-B-65 and ECS-B-78) and ITS (ECS-709, ECS-710, ECS-711 and ECS- 712) molecular markers. The comparison of sequences obtained from the 16S rRNA gene of the two strains isolated against the NCBI database indicated that they belon-ged to the genus Bacillus. Strain ECS-B-65 presented a high percentage of similarity (99 %) with B. hisashii and B. thermoamylovorans, while ECS-B-78 presented a high percentage of similarity with B. licheniformis (100 %). Regarding the fungal strains ECS-709, ECS-710, ECS-711 and ECS-712, the sequence of the ITS region indicated that the four isolates belonged to different genera: ECS-709 was similar to Aspergillus fumigatus, ECS-710 and ECS-712 to Rhizomucor pusillus, and ECS-711 was located molecularly as a member of the subdivision Pezizomycotina. Further studies carried out by traditional microscopy and according to its morphological features supported that this strain belonged to the genus Thermomyces according to the description by Ellis (1971). All analyzed sequences showed a similarity between 99 % and 100 % with the GenBank matched sequences (Table 5).
Table 5. Genetic affiliation of the study strains according to the comparative analysis based on the sequences of the 16S and ITS rDNA regions
Strain | Data Base | Coverage (%) | Identity (%) | Closest relative | N ° of access of the closest relative |
ECS-B-65 | NCBI Blast | 100 | 99 | Bacillus hisashii | AB909962.1 |
100 | 99 | Bacillus thermoamylovorans | FN397520.1 | ||
ECS-B-78 | NCBI Blast | 100 | 100 | Bacillus licheniformis | CP027789.1 |
ECS-709 | NCBI Blast | 100 | 100 | Aspergillus fumigatus | MH591451.1 |
ECS-710 | NCBI Blast | 99 | 99 | Rhizomucor pusillus | KJ527032.1 |
ECS-711 | NCBI Blast | 96 | 100 | Pezizomycotina | KC237297.1 |
ECS-712 | NCBI Blast | 99 | 99 | Rhizomucor pusillus | KJ527032.1 |
Discussion
Overview of microorganisms
In this work, the isolation and identification of thermophilic microorganisms (at 45 and 55 °C) during the substrate preparation by self-heating and growth during the cultivation of A. bisporus was performed. The development of the temperature during the pasteurization process allowed a thermal treatment of the whole substrate of at least 60 °C for six hours, which is considered to be enough to achieve pasteurization. It agrees with previous criteria and experiences that used pasteurized substrates by self-heating for the cultivation of Pleurotus spp., A. bisporus and other edible mushrooms (Morales and Sánchez, 2017; Colmenares et al., 2017).
The data regarding the population dynamics of thermophilic microorganisms in pangola grass pasteurized by self-heating varied between 2.6x104-4.6x108 CFU g-1 at 45 °C and 2.3x105-6.5x108 CFU g-1 at 55 °C, similar to what was reported by Souza et al. (2014), who in a study of thermophilic population diversity during phase II of composting for the cultivation of A. subrufescens found densities of 2.55x105-6x105 CFU g-1. These quantities are related to temperature profile, nutrient availability, oxygen concentration, moisture content and pH (Camacho et al., 2014), in addition to the availability of carbon produced by the degradation of lignin associated with the cellulose and hemicellulose of the substrates used (Wood, 1984). It has been reported for the genera Pleurotus and Agaricus in this sense, that the growth and production of the fruiting body depends not only on the fungus itself but also on the bacteria and other fungi on the substrate (Kertesz and Thai, 2018).
During pasteurization, bacteria were the predominant and abundant group of microorganisms, followed by filamentous fungi. Similar values were previously reported in wheat and rice straw compost, in which the prokaryotes along with a few fungi predominated during the first days of composting, while the actinomycetes prevailed during the final period of composting (Atkey and Wood, 1983; Cahyani et al., 2004). It has been described that some species of Bacillus, Pseudomonas and actinomycetes play an important role in the assimilation and transformation of ammonia, which is highly toxic to the mycelial growth of Agaricus species (Ryckeboer et al., 2003, Colauto et al., 2016). The presence of bacilli in the substrate for edible fungi after pasteurization is explained because they are fast growing and able to survive at temperatures greater than 55 °C through thermoresistance mechanisms (Torres et al., 2016).
Among the most abundant bacterial taxa in pasteurized substrates for the cultivation of species of Agaricus and Pleurotus are Pseudoxanthomonas taiwanensis, Thermus and bacilli (Bacillus, Geobacillus, and Ureibacillus) (Kertesz et al., 2016; Vajna et al., 2012), which produce α-amylases that degrade starch, as does Aspergillus spp., which also produce proteases, glucoamylases and pectinases. Bacillus produces extracellular enzymes that breakdown polysaccharides, nucleic acids and lipids, allowing the mushroom to use these products as carbon sources and electron donors (Cariello et al., 2007). Some species of Bacillus and Pseudomonas have the enzymatic capacity to degrade various cell wall components of certain fungi (Vargas et al., 2007).
The main role of the thermoresistant filamentous fungi that colonize the substrate during composting is to degrade lignocellulose, decreasing the amount of fiber and increasing the availability of the substrate for A. bisporus. These filamentous fungi are essential for the transformation of the substrate; in this study, they were represented by the strains A. fumigatus ECS-709, Thermomyces sp. ECS-711 and Rhizomucor pusillus ECS-710 and ECS-712, which were isolated during the manual turning of the substrate (R). Zhang et al. (2014) identified both Aspergillus spp. and Myceliophthora spp., as very abundant members of the compost stages of edible mushrooms. Moreover, the genus Rhizomucor has been isolated from decomposing soil, fruits or vegetables, fermentation and compost of organic matter. Rhizomucor spp., R. variabilis, are thermophilic in nature and can grow at 54 °C (Salar and Aneja, 2007).
Isolation and physiological features
In this work, only the strain ECS-709 of Aspergillus fumigatus presented the capacity to produce siderophores. Other studies (Singh et al., 2012; Zarenejad et al., 2012) have reported a higher proportion of siderophore-producing bacteria that are indirectly involved in the increase in mushroom production. However, Torres et al. (2016), when studying the mycosphere in a substrate for the culture of P. ostreatus, determined that 46 % of the isolates were able to produce siderophores. It is appropriate to observe that, in those works, mesophilic microorganisms were isolated and cultured at room temperature, while in the present work, emphasis was placed on the search for thermophilic microorganisms, which could contribute to explaining the difference in the characteristics of the isolated microbiota.
Of the 34 strains selected to evaluate the effect on mycelial growth of A. bisporus, 91 % were able to grow in the presence of 1-octen-3-ol. It has been found that in cultures in vitro, the compound 1-octen-3-ol inhibits the formation of primordia but does not inhibit the mycelial growth of fungi. In the culture of A. bisporus, the crop yield is related to the consumption of 1-octen-3-ol by Pseudomonas putida (Zarenejad et al., 2012). It has been shown that 1-octen-3-ol inhibits spore germination of Penicillium paneum (Chitarra et al., 2004) and Aspergillus nidulans (Herrero et al., 2011) and has been found to suppress the change in the reproductive phase in A. bisporus (Noble et al., 2009). The ability to consume 1-octen-3-ol could increase the yield of A. bisporus cultivation because it is directly related to the growth and development of the fungus (Eastwood et al., 2013) and the initiation of basidiocarps, and 1-octen-3-ol could also be used as an energy source for the beneficial microorganisms present in the crop (Noble et al., 2009).
In the phosphate solubilization test, 57 % of the microorganisms showed this capacity, and among them was the strain ECS-B-65 belonging to the genus Bacillus. Phosphorus is an essential macronutrient for the growth and development of plants and is involved in important metabolic pathways, such as photosynthesis, biological oxidation, nutrient uptake and cell division (Gupta et al., 2012). Filamentous fungi, such as those from the genera Aspergillus and Penicillium, have been recognized for their ability to solubilize insoluble phosphates by the release of organic acids such as citric, oxalic, malic and gluconic acids (Singh and Reddy, 2011).
Effect on the growth of Agaricus bisporus
In this study, strains belonging to the genera Bacillus (ECS-B-65 and ECS-B-78), Rhizomucor (ECS-710 and ECS-712), Aspergillus (ECS-709) and Thermomyces sp. (ECS-711) were found to have a positive effect on the growth of A. bisporus. It has been reported that Bacillus promotes the growth of P. ostreatus by secreting laccases and inhibiting the growth of Trichoderma harzianum (Velázquez-Cedeño et al., 2008). In the case of B. licheniformis, it synthesizes and excretes toxic peptides and bacteriocides that are capable of inhibiting the growth of yeasts, as well as gram-positive and gram-negative bacteria (Watabe et al., 2003). Thermomyces sp. ECS-711 and R. miehei ECS-712 promoted values of 0.51 and 0.76 mm/day of the radial extension rate (RER) of A. bisporus on PDA (Table 3). These results are lower than those reported by Coello et al. (2009), (between 1.4-0.9 mm/day on the substrate without previous colonization of Mycothermus thermophilus), and even lower than the 7 mm/day reported by Straatsma et al. (1994) when indicating that M. thermophilus is positively correlated with the production of mushrooms and strongly stimulates the mycelial growth of A. bisporus.
Op den Camp et al. (1990) indicated that the presence of M. thermophilus is important for the colonization of the substrate by A. bisporus. However, during this study, it was not possible to isolate this micromycete or other thermophilic fungi reported with similar characteristics that stimulate the growth of A. bisporus at the levels previously reported (Straatsma et al., 1991; Sánchez et al., 2008). All of the above findings indicate that the dynamics of microbial growth during pasteurization by self-heating allow the incipient presence of thermophilic microorganisms that promote the growth of A. bisporus as a consortium, with characteristics such as the consumption of 1-octen-3-ol, solubilization of phosphates, or siderophore production, in such a way that a faster growth of A. bisporus is observed with respect to the control grown on sterile substrate but still slow when compared with other more efficient substrates that have previously been reported (compost phase II, substrate colonized with M. thermophilus) (Ferreira et al., 2009).
The biological characteristics achieved at the end of 45 h of pasteurization by self-heating already allow the colonization of the substrate by A. bisporus but are insufficient for the colonization of outstanding beneficial microorganisms (such as Mycothermus thermophilus, Myriococcum thermophilum, and actinomycetes), with additional characteristics that promote the fast and competitive growth of A. bisporus and thus achieve a truly selective substrate. From this point of view, it is important to highlight that A. bisporus grew well though slowly on the self-heating pasteurized substrate, and thus, colonization of this noncomposted substrate may be improved. Therefore, it is interesting for future research to determine if it is worth trying to inoculate the substrate with other beneficial microorganisms at the beginning of the pasteurization by self-heating.
Conclusions
Microbial growth during self-heating pasteurization allows to evaluate the incipient presence of thermophilic microorganisms with characteristics such as 1-octen-3-ol consumption, phosphate solubilization and siderophores production. 106 thermophilic microorganisms present in the pasteurized Agaricus bisporus culture substrate were isolated.
The microorganisms Bacillus hisashii, B. thermoamylovorans, B. licheniformis, Aspergillus fumigatus, Rhizomucor pusillus (ECS-710 and ECS-712) and Thermomyces sp. that promote the growth of Agaricus bisporus were identified. The effect of mycelial growth was 0.51 and 0.76 mm/day.