Introduction
Biosensing systems are now a fundamental tool in healthcare, environmental monitoring, safety and protection. Nowadays, due to the pandemic outbreak generated by the SARS-CoV-2 virus, biosensors demonstrated a fundamental role offering reliable and low-cost results within a short response time to tackle such a pandemic scenario by means of timely diagnosis technologies (Huang et al., 2017).
Biosensors are analytical tools that facilitate the detection of (bio)molecules with a high degree of specificity and sensitivity. The fundamental idea behind biosensors consists of three main blocks (Figure 1), the first block consists of a biological recognition event or biorecognition event, which is extremely specific. The next block is the transduction and involves converting a biorecognition event into a measurable signal to acquire information related to the modulation of a specific physical phenomenon, such as fluorescence intensity, color change, etc. The resulting signal is then obtained and decoded (Srivastava et al., 2018; Velasco-Soto et al., 2015). It is important to note that biosensors with high sensitivity have the ability to produce a signal from smallest changes in analyte concentration. The limit of detection (LOD) of a biosensor, which is an indicator of analytical sensitivity, is determined by the smallest amount of analyte that the biosensor is able to detect (Vessman et al., 2001).

a) Sample. Substance to be analyzed in which is possible to detect the presence the target analyte. b) Biorecognition. This stage requires elements with high affinity against the target analyte. c) Transducers. A device that converts the measured biological activity into an analytical signal. d) Output. Measurable signal indicating the presence of the analyte in the sample
Source: Taken from Diana L. Mancera (2018).
Figure 1 Schematic representation of different blocks integrating a biosensor.
Herein, we provide an overview on a nanophotonic biosensing technology developed by our team. The biosensing platform is based on fluorescence or Förster resonance energy transfer (FRET) that takes place between fluorescent bioprobes acting as donors and graphene oxide (GO), acting as an acceptor. Unlike enzyme-linked immunosorbent assay (ELISA) in which blocking, separation and washing steps must be performed, this new technology allows an immunoassay to be realized through the use of a single antibody. The response of this new biosensing system can be interrogated in real-time and is observable within a few minutes (e.g., 5 minutes). Additionally, this system works with diverse photoluminescent agents and analytes.
FRET-GO based biosensor
The biosensing mechanism
The operational mechanism of our FRET-GO based biosensing platform is based on fluorescence quenching generated by the non-radiative phenomenon known as FRET (Figure 2A-B). In this non- radiative phenomenon, two materials are involved, a donor and an acceptor. FRET takes place when the emission spectrum of the donor overlaps the absorption spectrum of the acceptor. In addition, there must be certain distance between these molecules, particularly approximately 20 nm using graphene-related materials (Morales-Narváez y Merkoçi, 2018).

A. Overlapping between acceptor absorption spectrum and donor emission spectrum. B. Donor and acceptor molecular energy level schemes demonstrating correlated transitions. The vibrational transitions that occur in the donor molecule display the same energy as those that occur in the acceptor molecule.
Source: Taken from Diana L. Mancera (2018).
Figure 2 FRET mechanism. Schematic representation.
GO and its role in the biosensing platform system
Graphene is a carbon material. Due to its atomic thickness, it is considered a 2D material. It can be described as a planar sheet with several carbon atoms organized in a honeycomb arrangement which is the primary structure to build the others carbon allotropes (Figure 3) (Vasilios et al., 2015; Geim, Novoselov, 2007).

Source: Taken with permission from Vasilios et al. (2015).
Figure 3 Allotropes of carbon.
Besides, the oxidized version of graphene, graphene oxide (GO), possesses functional groups such as hydroxyl, epoxy, carbonyl, and carboxyl groups. Particularly, GO exhibits carboxyl groups onto the edges and the other oxygen-containing groups on the basal plane, as Figure 4 shows (Morales-Narváez y Merkoçi, 2012).
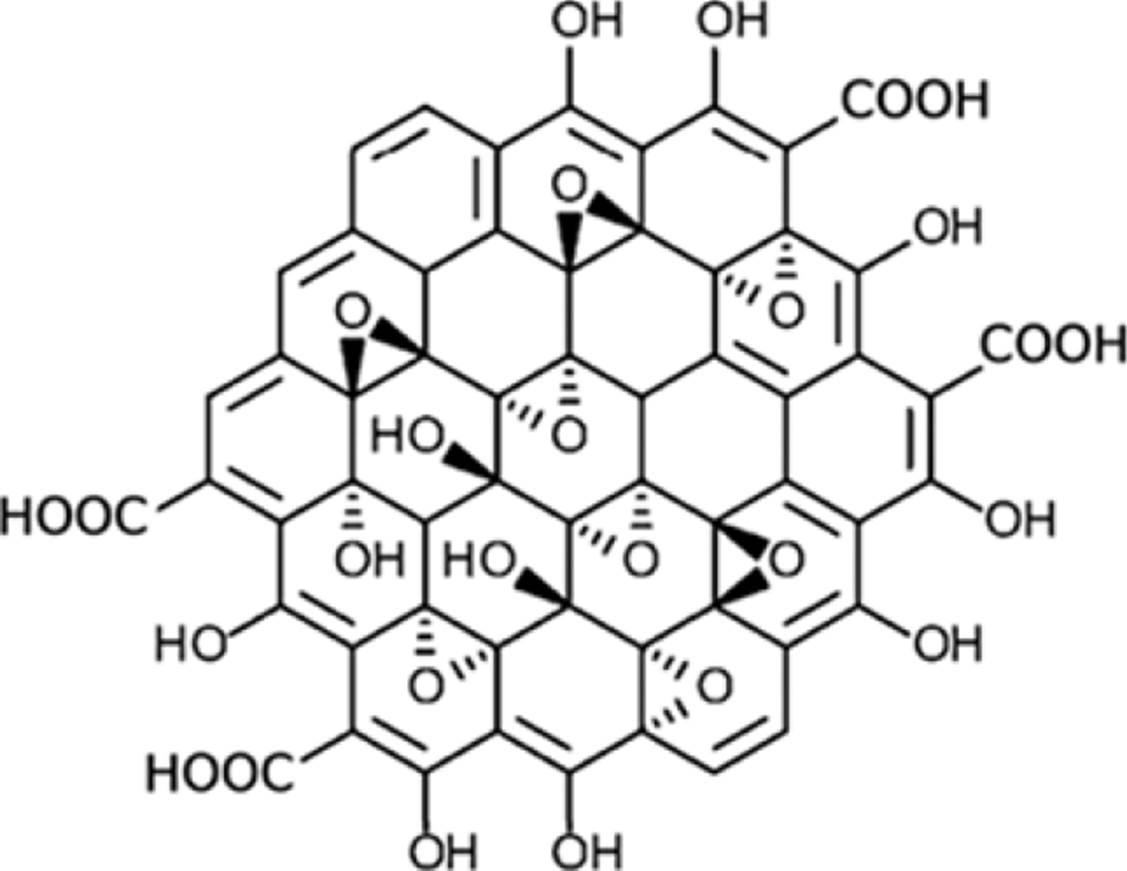
Source: Adapted with permission from Sinclair y Coveney (2019).
Figure 4 Representation of the structure of GO.
The Hummer approach is the most frequent method to produce GO. Importantly, ionic groups like O− and COO− found in GO enable this substance to engage electrostatically with proteins or DNA.
Literature reports the extraordinary optical properties of GO. For example, the wide spectral absorption range of GO is one of them. Hence, GO can be exploited as a universal acceptor in FRET (Forster, 1946). The proposed biosensing platform is featured by a surface coated with GO (FRET acceptor) and a fluorescent bioprobe (FRET donor). We discovered that the GO-coated surface has a strong affinity against those bioprobes that do not interact with the analyte, thus the respective fluorescence is quenched when no analyte is present in the sample; whereas, those fluorescent bioprobes interacting with the analyte are not quenched since such a complex (bioprobes-analyte) have no affinity against the GO-coated surface and the analyte also represents a spacer between the GO-coated surface and the bioprobes (Fluorophore-labelled antibody), as Figure 5 shows.

The GO-coated surface has a strong affinity against those bioprobes that do not interact with the analyte, thus the respective fluorescence is quenched when no analyte is present in the sample; whereas, those fluorescent bioprobes interacting with the analyte are not quenched since such a complex (bioprobes-analyte) have no affinity against the GO-coated surface and the analyte also represents a spacer between the GO-coated surface and the bioprobes.
Source: Reproduced with permission form Ortiz-Riaño et al. (2020).
Figure 5 Operational mechanism of the biosensing plataform.
FRET-GO based biosensor implementations
The proposed technology has been demonstrated to be a techically sound immunosensing platform. In general, microwell plates have been coated with GO to implement this novel technology (Ortiz-Riaño et al., 2020).
Costar 3603 are the microwell plates employed.1 The microwells surface is characterized to be hydrophilic, negatively charged, and consisting of 9-17% oxygen atoms (Li, 2008). Hence, the surface of these microwells is able to attach GO via hydrophilic contacts and hydrogen bonding given the aforementioned oxygen-containing groups of GO.
As a proof of concept, the FITC-labeled anti-human IgG antibodies (IgGFITC) with different concentrations of human IgG (H-IgG) were combined into GO-coated microwells (GOμW) and their photoluminescent response was explored in real-time. Figure 6A displays the optimal performance of this novel immunoassay targeting H-IgG. This analysis indicates that this immunodetection platform effectively reports the presence of the analyte by showing that the fluorescence levels (If/I0) are proportional to the concentration of the analyte. In addition, to evaluate the performance of this biosensing platform in a real matrix, human urine samples doped with different concentrations of prostate-specific antigen (PSA) were analyzed within 10 minutes, see Figure 6B-C.

A. Real-time analysis of different concentrations of H-IgG and a blank sample. B. Real-time analysis of different concentrations of PSA and a blank sample. C. Calibration curve resulting at 10 minutes of the proposed immunoassay (PSA detection).
Source: Adapted with permission from Ortiz-Riaño et al. (2020).
Figure 6 Analytical performance of the immunosensing platform targeting HIgG and PSA.
In addition, this immunodetection platform was reported to exhibit an analytical sensitivity that is comparable to that of ELISA, with acceptable precision and accuracy (Table 1). Besides, other relevant advantages of this innovative immunodetection platform is that it can be interrogated in real-time, works with a single antibody and avoids washing, blocking and separation steps.
Table 1 Comparison of this approach with ELISA in terms of assay time and LOD.
Analyte | Method | Assay time (min) | LOD (ng mL-1) | Reference |
---|---|---|---|---|
H-IgG | ELISA | 360 | 1.60 | (“IgG (Total) Human Uncoated ELISA Kit - Invitrogen”) * |
GOµW | 45 | 2.56 | Ortiz-Riaño et al. (2020) | |
PSA | ELISA | 360 | 0.10 | Najeeb et al. (2017) |
GOµW | 90 | 0.02 | Ortiz-Riaño (2020) | |
E. Coli | ELISA | 180 | 3 | (E. coli HCP ELISA Kit, 2G (F1020) ** |
GOµW | 30 | 2 CFU mL-1 | Avila-Huerta et al. (2020) | |
SLD | ELISA | 360 | 0.031 | Cortés-Sarabia et al. (2020) |
GOµW | 120 | 0.012 | Rodríguez-Nava et al. (2020) | |
COVID-19 Antibodies | ELISA | (25-43) | pg mL-1 to ng mL-1 | Steiner et al. (2020) |
GOµW | 42 | 0.003 | Avila-Huerta et al. (2021) |
* IgG (Total) Human Uncoated ELISA Kit - Invitrogen. https://www.thermofisher.com/mx/es/home/life-science/antibodies/immunoassays/elisa-kits.html?gclid=EAIaIQobChMI_t-zqdeO_wIVhQ99Ch0vsAKvEAAYASAAEgJG2PD_BwE&ef_id=EAIaIQobChMI_tzqdeO_wIVhQ99Ch0vsAKvEAAYASAAEgJG2PD_BwE:G:s&s_kwcid=AL!3652!3!415798135406!p!!g!!invitrogen%20elisa!9205681679!93387333557&cid=bid_pca_iel_r01_co_cp1359_pjt0000_bid00000_0se_gaw_bt_pur_con.
** E. coli HCP ELISA Kit, 2G (F1020). https://www.abcam.com/products/elisa/e-coli-hcp-elisa-kit-hostcell-protein-ab240997.html.
Source: Author’s elaboration.
Taking advantage of the versatility of this novel GOμWs-based technology, a nanophotonic immunoassay was developed for the diagnosis of bacterial vaginosis (BV), which is an infectious vaginal disease caused by the overgrowth of bacteria naturally found in the vagina, which disrupts its natural balance (Wu et al., 2019). The evaluation of the Amsel criteria (Amsel et al., 1983) and the Nugent score (Nugent et al., 1991) are typically used to make a clinical diagnosis of BV. Both approaches rely on observations made by highly qualified professionals who have even undergone extensive training. In BV infection, the enzyme known as sialidase (SLD) plays a very important role since several studies have found a close relationship between its production and the development of BV infection. Hence, SLD has been introduced as a potential biomarker of BV infection (Van de Munckhof et al., 2019).
In this context, a one-step quantitative biodetection system was designed to detect SLD concentrations. For this purpose, a monoclonal antibody (mAb) against SLD, developed by our colleagues in Universidad Autónoma de Guerrero, was exploited. Several immunological methods have shown that this mAb can recognize sialidase with high sensitivity (Cortés-Sarabia et al., 2020). Hence, in our immunoassay, mAb-decorated quantum dots (mAb-QD) were employed as a fluorescent bioprobe to interrogate the presence of SLD and GOμWs were also employed. A schematic representation of our nanophotonic immunoassay targeting SLD is shown in Figure 7A. With this nanophotonic immunoassay, 162 clinical samples were analyzed with a clinical sensitivity/ specificity accounting for c.a. 96%. Besides, it was demonstrated as a simple and cost-effective tool to detect BV, especially when compared with conventional techniques such as indirect ELISA (Rodríguez-Nava et al., 2021).

A. SLD Immunoassay diagram. B. Scheme of the biosensing platform targeting E. coli. C. Biosensing system for COVID-19 seroconversion. D. Schematic representation of the measurement of binding kinetics constants. Source: A: Adapted with permission from Rodríguez-Nava et al. (2021).. B: Adapted with permission from Avila-Huerta et al. (2020), Copyright © 2020 American Chemical Society. C: Adapted with permission from Avila-Huerta et al. (2021). Copyright © 2021, American Chemical Society. D: Adapted with permission from Edwin et al. (2022). Copyright © 2022, American Chemical Society.
Figure 7 Graphical representation of real-time biosensing platform in different approach and analytes detection.
Food safety is another field in which having an efficient, fast, economical and at the same time reliable biosensing system is of greatest importance since late detection of pathogenic bacteria can be a great threat to human health as well as to the ecosystem. Exploiting the same nanophotoic technology, we reported a rapid pathogen detection platform (Avila-Huerta et al., 2020). Particularly, our biosensing platform was used to detect Escherichia coli, even in cauliflower extracts, see Figure 7B.
The aforementioned configuration provided an optimum analytical performance at 30 minutes. As a result, the developed bacterial detection platform was shown to be quick and extremely sensitive, with a limit of detection of around 2 CFU mL-1.
Our nanophotonic immunoassay was also adapted to offer a useful tool in the recent pandemic scenario. In fact, we engineered an immunoassay targeting COVID-19 antibodies. Particularly, based on the fact that the fluorophore-tagged recombinant SARS-CoV-2 spike receptor binding domain protein (F-RBD) can act as a bioprobe that emits fluorescence upon detection of the COVID-19 antibody, a biosensing system for determination of COVID-19 seroconversion was developed (Figure 7C). The cost of this test is about 0.5 USD per unit (laboratory scale). In addition, the biosensing system provides optimal results within 42 min. With the intention of proving that this technology is technically sound in a relevant environment, 34 human serum samples were examined, as well (Avila-Huerta et al., 2021).
So far, our biosensing platform has been discussed as a versatile, low-cost, efficient and advantageous tool, especially when compared with conventional techniques such as ELISA. However, the most recent work in which this system is involved concerns the study of the macromolecular association between the biorecognition probe and the corresponding analyte. It is known that macromolecular association is crucial in drug development, diagnostics and gene editing, however macromolecular association study requires expensive instrumentation and complicated procedures (Patel et al., 2017). Since the developed nanophotonic immunoassay operates in real-time, binding constants displayed during protein-protein association can also be determined by means of our innovative technology. Figure 7D shows a schematic representation of this innovative technology to determine protein kinetics. This one-step platform was used to determine the binding data for three protein pairings, and the results were compared to data provided by suppliers or the literature, indicating that this approach is comparable with existing methods. These pairs consist of: 1) human immunoglobulin G (H-IgG)-fluorophore-tagged anti-H-IgG; 2) prostate-specific antigen (PSA)-quantum dot-tagged anti-PSA, and, 3) recombinant anti-RBD protein-fluorophore-tagged SARS-CoV-2 spike receptor-binding domain. Additionally, an open-source software that determines protein binding kinetic constants was offered (Edwin et al., 2022).
Conclusions and outlook
We discussed that graphene oxide-coated microwells, GOμWs, combined with photoluminescent bioprobes are a powerful tool for the implementation of an advantageous biosensing system, which can be considered a highly transformative immunoassay in a standard format (the 96-microwell format). Moreover, it is important to note that, due to the universal fluorescence quenching ability of GO, it is possible to engineer a photoluminescent immunodetection platform operating at different wavelengths, which may be useful for future multiplexed applications. Besides, the proposed nanophotonic immunoassay can be transferred to on-site applications using miniaturized technologies involving microfluidics and/or paper-based analytical devices. In addition, to improve the clinical sensitivity and specificity of our biosensing system, computational tools such as artificial intelligence can be employed.