Introduction
Phthalocyanines are 18 π-electron molecules that present a highly aromatic behavior and exhibit excellent catalytic, photochemical, and optical properties (Jia et al., 2018; Huang et al., 2021; Wang et al., 2015; Manbeck & Fujita, 2015; Chen et al., 2018; Ramos et al., 2015; Farajzadeh et al., 2021; Gregory, 2000). The central ring of the phthalocyanine possess (=N-) bridges and benzene rings combined in pyrrole rings, forming a square planar coordination site with a four-fold axial symmetry producing strong equatorial bonds which favor the delocalization of the π-electrons (Ishihara et al., 2014; Torre et al., 2013), see Figure 1-a. Along these π-bonds, there are significant effects on the possible axial ligands, and this fact activates a feasible catalytic reaction, depending on the ligand (Sorokin, 2013; Pizarro et al., 2018). The preferred ligands are metallic atoms at the center, often called metallophthalocyanine (MPC), see Figure 1-b. These are very popular in the scientific literature because they exhibit good thermal and chemical stability (Demirol et al., 2020; Gorduk et al., 2021; Aimi et al., 2018). Zinc phthalocyanine tetrasulfonic acid ZnTsPc is an incredible electronic donor-acceptor coordination complex that has many electrocatalysis applications (Hou et al., 2015). Additionally, the phthalocyanine can be modified by substituting a benzene ring on the periphery (Araujo et al., 2016), changing the physicochemical properties. The phthalocyanine molecules can be used as building blocks in supramolecular systems (Torre et al., 2013). The combination of phthalocyanines with other molecules such as porphyrin generates supramolecular homo and heteronuclear systems with a multicomponent donor-acceptor conjugate (Torre, 2013), which presents heavy light-harvesting applications (Torre, 2013) or electrocatalytic properties (Araki & Toma, 2006). Furthermore, the substituent can create additional interaction sites for changing the structure of the activated complex centered on the phthalocyanine and increase the electric conductivity, which can generate supramolecular electrode materials (Foster et al., 2014; Toma & Araki, 2009). These conditions make it possible to introduce suitable metal clusters, oxides, or nanoparticles to create hybrid supramolecular materials and enhance their charge-transfer properties, as catalysis or conductivity.
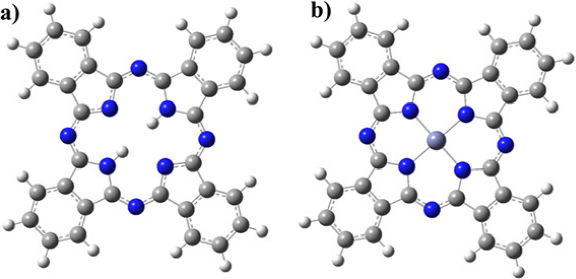
Source: Author’s elaboration.
Figure 1 a) Phthalocyanine molecule as a brick of construction of bidimensional organic materials. b) Metallic atoms at the center of the phthalocyanine are often called metallophthalocyanine.
Electrostatic self-assembly allows the spatial organization of atoms by creating many interactions to stabilize the new structure in a unique molecular framework. The self-assembly molecules can be connected via covalent bonds or noncovalent interactions. The most exciting facet of the self-as-sembly compounds is that they create active sites that minimize energy, as if they had been carefully planned, with bridging groups making the supramolecular compound thermodynamically stable. Concerning the phthalocyanine supramolecular compounds, if the connection is by noncovalent interactions, like hydrogen bonding interactions, surface effects, or π-π stacking, several advantages are presented, relative to its ease of synthesis and self-repair. For example, one of the simplest methods to obtain a large chromophoric assembly is to mix porphyrins or phthalocyanines with an ionic substituent in aqueous solutions, which has been proven to form self-assembly compounds (Molla & Ghosh, 2014; Zhong et al., 2020; Teixera et al., 2021). In contrast, if the connection is by covalent bonds, the phthalocyanine molecules form structures often called covalent organic frameworks (COFs) (Côté et al., 2005; Geng et al., 2020), which belong to a porous and crystalline material with promising applications as sensor or gas storage and gas separation (Xu et al., 2015; Lu et al., 2017; Haase et al., 2018).
In 2005, graphene was synthesized, and it has generated a boom of research regarding bidimensional materials. Graphene is a two-dimensional sheet of sp2 hybridized carbon atoms (Allen, 2010). One of the many branches of this new material science is to use some molecules as a brick of construction of more giant molecules. These construction bricks make it possible to create layered materials characterized by an extended crystalline planar structure with strong in-plane covalent bonds and weak out-of-plane non- covalent interactions (Ajavan, et al., 2016). These bricks of construction have been attempted with many elements of the periodic table, like phosphorus (Carvalho et al., 2016; Ren et al., 2017), MoS2 (Sun et al., 2017), stanene (Balendhran et al., 2014), and germanium (Acun et al., 2015). For organic molecules, the bricks of construction have been organic coordinate polymers, porphyrins, and phthalocyanines.
Considering the conditions already mentioned, it has been possible to create new bidimensional materials based on phthalocyanine supramolecules. It has been seen that MPC are very popular in forming new bidimensional materials because they present a vast number of essential applications as photoelectrocatalysis (Yu, 2020; Lin, 2015; Sick, 2018; Biswal, 2019b), optoelectronic devices or photovoltaics (Zhang, 2014; Feng & Ding, 2012; Ding & Wang), charge carrier transport (Dogru et al., 2013), and chemiresistive sensing (Meng et al., 2019). To improve the physicochemical properties, such as the electronic in π-conjugated bonds, the links between the phthalocyanines can be organic molecules that enhance the carbon-carbon bonds, or pyrazine unites or conjugated polymer structure (Meng et al., 2019; Sick et al., 2018; Jin & Hu, 2017; Zhuang et al., 2016; Guo et al., 2013). Excluding the conjugated links, planar connections can also be used to improve electronic mobilities (Spitler & Dichtel, 2010; Biswal, 2019a). The main advantages of these new bidimensional materials are that they present high mobility of the π-electrons and substantially improve charge transport properties.
To understand the behavior in detail of the bidimensional materials based on phthalocyanine, computations have proved to be very effective because they provide relevant information on the covalent and noncovalent interactions. In the present review, we will present an overview of the computational studies on these materials and their main applications.
Tessellations as the main character in bidimensional materials based on phthalocyanine (2D-PC)
Due to the nature of phthalocyanine molecules, there are several ways that they can be used as building blocks. From a mathematical point of view, the construction of a finite or infinite plane using building blocks as edges and vertices is often called a tessellation process (Jin et al., 2017). A very famous example of tessellations is the Alhambra palace in Spain. It is a very characteristic example, and it has been proved that the tessellations created contain all the spatial groups of nature (Müller, 1944). Also, M.C. Escher created a huge part of his artwork using tessellations. Again, he used all the bidimensional space groups available. When patterns are designed with different angles at each vertex, the combination results in infinite possibilities in tessellations. However, if the tessellations are created with regular polygons, the number of options is drastically reduced. A few types of tessellations can be used to develop new 2D-MPC materials; depending on the patterns or symmetries, will be the physicochemical properties that the material has. This feature present in two-dimensional materials is very appealing and can motivate the creativity of scientists to do more engineering and design new materials. As it was mentioned before, the MPC is extremely popular among the scientific community, and for this reason, the first scientific works were of bidimensional materials based on phthalocyanines, which used MPC structures as a brick to create supramolecular compounds.
A very interesting and complex tessellation created with phthalocyanines is making a four-fold complex, with a square shape of the pore. As in many other bidimensional materials, the shape of the pore, i.e., the center of the junction of the phthalocyanines, makes the material ideal for many interesting applications, mainly for gas separation or molecular trapping. Figure 2 shows a generalization of these types of tessellations. In a previous work made by Shinde et al. in 2015, it is possible to observe the applications of these micropores made by bifunctional and bidimensional covalent organic frameworks. These frameworks were made with phthalocyanines functionalized with hydrogen atoms at the center. They proved that the internal pores are excellent catalytic sites that present a remarkable ability to perform a cascade reaction of several catalytic reactions (Shinde et al., 2015).

Source: Shinde et al. (2015).
Figure 2 A 2D-PC complex with a square geometry is shown. This figure represents a generalization of the structures presented by Shinde.
Last year, other studies published by De Siddhartha have the main target to design phthalocyanines-based metal-organic frameworks (MOFs) (De Siddhartha et al., 2021). To accomplish this purpose, they used the previously mentioned tessellation, the four-fold geometry with conjugated molecules as bridges. Fateeva et al. developed phthalocyanines bearing alternative coordinating groups that would allow to move the hypothetical MOFs designed beyond metal-carboxylates and which have achieved new topological and physicochemical properties. In this study, they explored the hot topic of MOFs and confirmed that phthalocyanine MOFs present very little structural data available so far. Currently, the only structure data available has the four-fold square shape, for example as shown in Figure 2. This experimental data was experimentally reported by Matheu et al., based on the catechol MPC with Co and Fe (Matheu, 2019). The central metallic atom is coordinated with four catechol groups, and then these catechol groups are connected to four MPC molecules. This experimental complex has a pore size of 1.07 nm and a second pore of 1.46 nm. For this three-dimensional compound, there is a series of new 2D layered MOFs based on the catechol MPC based on several metallic atoms. From these structures, the crystallinity is very low. In general, the simulation of these structures used an AA stacking model with a square planar metal coordination geometry. Each metal atom is linked to two catecholate and then upon two phthalocyanine ligands. Experimental conductivity measurements were performed by Nagatomi et al., in which they reported a conductivity value around 1.6 x 10-6 S cm-1 (Nagatomi et al., 2018), which reflects a high conductivity and is very promising for constructions of Li-ion batteries.
A work that is very worth mentioning is one made by Wang et al., in this work, they designed a 2D-MPC material using two novel pyrazine links and MBC as bricks of construction with Zn and Cu as the metallic center (Wang et al., 2019), as seen in Figure 3. They synthesized the materials and performed computational studies to examine thermal and chemical stability, as well as optical and electrochemical properties. They made their computational calculations using density functional theory (DFT) with the computational package Quantum Espresso. They used the generalized gradient approximation (GGA) for the functional to estimate the electronic correlation and overlapping, and they localized the d-electrons with the DFT+U method. The dimensions of the unit cells used were a = b = 2.22 nm, and there is an interplanar distance of c = 0.33 nm. The pyrazine was linked with the MPC of Zn or Cu via van der Waals interactions. The supramolecular complexes studied were exciting materials, with a p-type semiconductor behavior and an electronic bandgap of 1.2 eV. They demonstrated that the p-type semiconductor by changing the metal center from Cu to Zn in the phthalocyanine molecule does not affect the conductivity, around 5 x 10-7 S/cm, and charge density approximated to 1012 cm-3. The charge carrier transport is highly anisotropic, which is a good quality for bidimensional materials due to holes that do not present mobilities in-plane and limiting mobilities out-of-plane. They corroborate the electric conductivity with the electronic band spectra, which provide an estimate of the effective mass of the complex, see Figure 3. The electronic bandgap is around 0.8 eV to 0.55 eV, and it is attributed to interlayer π-π interactions.

Source: The band structures are reprinted with permission from Wang et al. J. Am. Chem. Soc. (2019), 141, 16810-16816. Copyright 2021, American Chemical Society.
Figure 3 It is shown a 2D-MPC complex, and the band structure of the complex proposed by Wang et al. (2019). This compound is a construction of the 2D-MPC crystal-synthesized in Ref. Wang et al. (2019).
Another interesting study was made by Sun et al., in which they designed a 2D-MPC material with Co (Sun et al., 2015). Here, the MPC was used as a brick of construction differently, see Figure 4. They made a computational study within the framework of DFT, using the PBE functional with the computational package Vienna Ab Initio Simulation Package (VASP). Likewise, the compounds have been synthesized; the substrate on which the 2D-MPC was built was Ag (110). The resultant geometry has completed a consequence of a self-assembled process. As it can be seen, the central pore was significantly bigger, suggesting applications as traps of other molecules or drugs, and separation of gases. A closer view of the geometry indicates that the majority of the MPC molecules are connected between them in a uniform, staggered fashion. The aryl-aryl coupling occurs explicitly at the C2 site of the MPC molecule, just as previously reported by Sun et al., aryl-aryl coupling of a quaterphenyl molecule (Sun, 2014). Several facts determine the physical and chemical properties: first, how the phthalocyanine is used as a construction brick. Second, the metal-ligand that is used at the center of the phthalocyanine. Third, the angles of the pattern also modify the physical and chemical properties. Sun et al. show different structural motifs, which vary the electrical energy by almost 0.5 eV. The differences between the motifs are, mainly, the internal angles of the principal pore. The ground-state configuration corresponds to a square formed by the MPC with angles smaller than 90 degrees.

Source: The figure is a representation made by us of the structure reported in reference Sun et al. (2015).
Figure 4 A 2D-MPC material based on Co atom synthesized by Sun et al.
There are still some other exciting tessellations left to test. Generally, these tessellations have been obtained experimentally, but they have not been computationally analyzed. To exemplify the tessellations left to try it is essential to apply the regular polygon restriction mentioned before. If identical polygons are at each vertex, counting them either in a clockwise way or anticlockwise, there are few possibilities. In general, a tessellation could be made with any regular polygon and identical vertices with adjacent polygons sharing one entire edge, which are known as the Archimedean tiling. In general, the bidimensional covalent organic frameworks (COFs) use the Archimedean tiling to create their tessellations, in which any polymer or organic molecule is used as a polygon (Geng et al., 2020). For a phthalocyanine molecule, not all the Archimedean tilings are feasible because the phthalocyanine molecule has four vertices, making only the Archimedean tilings with the polygon as a square. It is also possible to mix the polygons with different ligands to increment the number of possible tessellations available. It has been proved that tile assemblies in an Archimedean tiling affect the force-deflection response as stiffness, load carrying capacity, and toughness. Furthermore, it has been proved that for small tile areas, the mechanical behavior is improved substantially (Williams & Siegmund, 2021). Figure 5.A) shows the possible Archimedean tiling possible with one regular polygon. The three figures are denoted as 4·4·4·4, which reflects that a four-fold polygon is connected to another four-fold polygon. Figure 5.B) shows a modification of these Archimedean tiles with a hexagonal ligand, and an asymmetry of 4·6·4. These tessellations are the equivalent to the Archimedean tiling with two regular polygons. Also, the ligands can be repeated if required, forming a “ligand chain” that increments the pore size as desired. The ligand chain symmetry is 4·2·4. Figure 5.C) shows a hypothetical Archimedean tiling with three regular polygons. In this figure, the hexagonal ligands form a three-fold polygon creating asymmetry of type 4·3·3·4. In current scientific literature, as shown here, the tessellation is studied computationally and synthesizes the ones with one regular polygon and two regular polygons. We propose the three-fold polygon as the next generation of bidimensional materials. In addition to regularly connected tessellations, there are other possible irregularly connected tessellations where the angle or the sequence of the ligands can be changed. This means that there are more possibilities in this new engineering of two-dimensional materials based on phthalocyanine. These 2D-MPC materials show a large surface area, high crystallinity, and porosity that make them very worthy of attracting the scientific community’s attention.

Source: Author’s elaboration.
Figure 5 Some possible regular tessellations are available with the phthalocyanine molecule. A) These three first tessellations are Archimedean tilings with one regular polygon. B) The tessellations represented here are a modification of the Archimedean tiling with the combination of a hexagonal polygon to create Archimedean tiling with two regular polygons. C) A hypothetical tessellation with three regular polygons.
Figure 6 shows A type structures (4·4·4·4) experimentally synthesized. Figure 6.A) is a two-dimensional structure synthesized by Kubicki et. al, they grew up Co phthalocyanine films on the one-dimensional Si(111)(4 x 1)-In surface with a (4 x 4) periodicity (Kubicki, 2021), in which the structures is connected by four-fold polygons. They obtained these structures using scanning tunneling microscopy (STM) and overlapping the molecular structure model. Another good experimental visualization was made by Cirera et. al., where they show various flat structures with phthalocyanines (Cirera et al., 2016). They held another interesting point of view, they propose the design by using the building blocks not as tessellations but as monomers, such that the creation will be done as polymers. Figure 6.B) shows a structure created with phthalocyanines as monomers, where the symmetry is still (4·4), joining the monomers via their benzene rings. Another experimental result with one of the B tilings is shown in Figure 5 and was obtained by Bischoff et al., where they used structures of Porphyrins, bonded with Cu-based organometallic structures (Bischoff et. al., 2016).

Source: 6A: Kubicki (2021), this figure was taken from Appl. Phys. Lett. 119, 133105 (2021) with copyright of the AIP Publishing. 6B: Cirera (2016), presenting in Nature Communications, 7: 11002 (2016) with copyright. 6C: Bischoff et al. (2016), in Eur. J., 22, 1-10 (2016) with copyright of the AIP Publishing.
Figure 6 Three scanning tunneling microscopy images (STM) of two-dimensional surfaces are shown: A) A two-dimensional cobalt phthalocyanine films obtained by Kubicki et al. B) An experimental structure created with phthalocyanines as monomers is shown here, where the symmetry is (4·4), uniting the monomers via their benzene rings synthesized by Cirera et al. C) Experimental structure type (4·2·4) where the links are made with organometallic structures obtained by Bischoff et al. (2016).
Some applications of bidimensional materials based on phthalocyanine (2D-PC)
As we mentioned before, there are several essential applications related to 2D-PC. The most essential and popular are for catalytic purposes. The most outstanding applications are discussed below.
The first application mentioned here is ammonia synthesis. For industrial and agricultural purposes, ammonia is an indispensable substance because it is a precursor for nitrogen fertilizers and other nitrogen-containing compounds related to the industry (Erisman et al., 2008; Galloway et al., 2008). To produce ammonia in the industry, the Haber-Bosch process is used. However, this method is very harsh due to the high temperature and pressure needed, besides the negative consequence of massive carbon dioxide production. Due to these facts, there is an urgent need to find a more efficient wave to produce ammonia. An alternative reaction is the electrocatalytic nitrogen reduction reaction, which uses a pair of nitrogen atoms and protons as reactants to generate ammonia. The electrocatalytic reaction is N2 + 6H+ + 6e- = 2NH3. There are several advantages to this reaction. For example, there is no need for high pressures and temperatures, and the proton source in this method is water in-stead of H2. The key to performing this electrocatalytic reaction is obtaining a strong catalytic activity, and two-dimensional materials have been presented as an important platform to design a successful reduction of N2 to NH3 (Yang et al., 2018; Zhao et al., 2019; Gao et al., 2020). There is a new branch of study in which these materials are the principal target of the design of single-atom catalysts (SACs). In this new branch of SACs, there are several effective catalysts reactions proposed, such that FeN3 adsorbed on graphene at room temperature can help to convert H2 to NH3 (Li et al., 2016). The main disadvantage of graphene in this catalytic reaction is, in large-scale applications, it is challenging to induce suitable pores and subsequently embed metallic adatom. That is one of the main reasons why phthalocyanine is the perfect option. As we mentioned before, there are several ways to perform a suitable porosity in these bidimensional materials and to firmly embed isolated single metallic atoms. In the work made by Huang et al., they optimized around sixty 2D-MPC with the computational simulation package Vienna Ab Initio Simulation Package (VASP), with thirty metals, like Ti, Cr, Zr, Nb, Mo, Tc, Hf, Ta, W, Re, Sc, V among others (Huang, 2021). They used systems based on Archimedean tiling of 4·4·4·4 types (the middle tessellation shown in Figure 5.A). To evaluate the catalytic activity of the systems proposed, the first step is a study of adsorption of the N2 molecule, which is a prerequisite for the conversion of N2 to NH3. Then, the second step is to calculate the Gibbs free energy changes for N2H (ΔGN2H) adsorption. It is known that the convention of the sign in the Gibbs free energy depends on the definition taken by the authors, for example, negative Gibbs free energy for exothermic reaction and positive for endothermic process. Huang et al. used this convention, which is equivalent to the N2 and N2H molecules spontaneous adsorption over 2D-MPC. The candidates taken by them with a good catalytic activity were the systems in which the metal catalysts with ΔGN2H are less than 0.8 eV. This energy threshold was selected because a small ΔGN2H reflects slightly uphill in the free energy, and the step does not need much energy at the beginning. The most novel systems found by Huang et al. were with Mo, Re, and Tc as atomic atoms. These systems present significant orbital hybridization and charge transfer between the N2 and the 2D-MPC system. In comparison with this research, a study made by Liu et al. reaches similar conditions. They conclude the high feasibility of nitrogen reduction to ammonia on 2D-MPC systems with Mo (Liu, 2020). Also, Liu et al. Show that 2D-MPC is an excellent substrate for the design of single-atom catalysts.
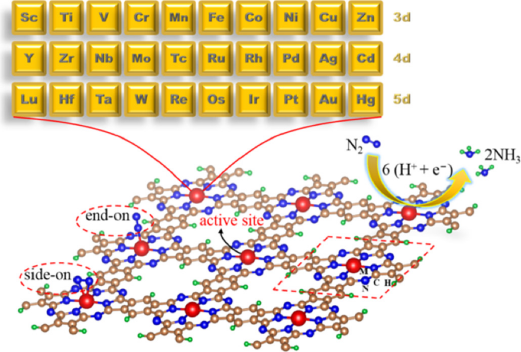
Source: This figure is reprinted with permission from Huang et al. ACS Appl. Mater. Interfaces (2021), 13: 608-621. Copyright 2021, American Chemical Society.
Figure 7 The systems studied by Huang et al. electrocatalysis are up-and-coming in the 2D-MPC proposed by them.
Another exciting application of 2D-MPC worth mentioning is the electrochemical reduction of CO2 to carbon compounds which promises a route to carbon-neutral energy (De Luna et al., 2019; Montoya et al., 2016). In general, metal complexes have been used to catalyze the reduction of CO2 at high current densities because they promote fast electron transport properties and increment the catalytically active sites (Yaghi et al., 2019). 2D-MPC, MOFs, are ideal candidates to substitute the metal complex as catalytic elements due to their extended porous structures (Dibetsoe et al., 2015). A work published by Matheu et al., reported the electrochemical reduction of CO2 using a 2D-MPC linked with 3D metal-catecholate frameworks, which are a class of MOFs (Matheu, 2019). The metal-catecholate presents a high electronic transport due to the overlap of the valence orbitals of the metallic atoms (Fe, V). The phthalocyanines of this material are based on Co, in which the structure has a framework of [Fe6(OH2)4(CoPc)3]6- and Fe3(-C2O2-)6(OH2)2 trimmers. Each phthalocyanine with Co is coordinated with four Fe3(-C2O2-)6(OH2)2 trimmers. Mathieu et al., mentioned that electronic transport is promoted due to the in-teractions forming several one-dimensional channels instead of π-π stacking interactions, which improves the accessibility of the catalytic CoPc sites. Another study made, related to the CO2 reduction, was driven by Manbeck et al., on iron and cobalt phthalocyanines (Manbeck, 2015). They mentioned that metal phthalocyanines are visible light absorbers. Nonetheless, the photochemically generated catalyst activity for CO2 reduction is lower than expected because there is no photoinduced second electron. But, for electrochemical CO2 reduction, this situation does not apply. Also, reduced Fe and Co phthalocyanines react with protons to produce covalent hybrids, in specific, metal hybrids, which are intermediates steps for H2, HCOO-, and CO production.
Another interesting application, much different from catalysis, is the corrosion inhibitor for aluminum. A study made by Dibetsoe et al. proved that some phthalocyanines and phthalocyanine derivatives are excellent candidates for corrosion inhibitors of aluminum in the acidic medium (Dibetsoe et al., 2015). They made an experimental and computational study to understand the phenomenon involved in the corrosion and prevent it. They proved with seven systems and concluded that there is an appreciable inhibition efficiency on corrosion, which diminishes as the temperature is increased from 30 ºC to 70 ºC. The adsorption was studied with the phthalocyanine molecules as adsorbate and aluminum surface as substrate, observing that the adsorption is spontaneous and involves forces within physisorption and chemisorption mechanisms. Also, there are strong interactions between the studied inhibitors, 2D-PC, and metal surfaces.
Conclusions
The 2D-MPC materials are novel and promising materials for many applications like catalysis and developed electronic devices due to the high electronic transfer and malleability that presents. The phthalocyanine molecules can be used as a brick of construction of new bidimensional materials. The advantages that are present over other bidimensional materials are the ease to induce suitable porosity and embed isolated metal atoms on the structure for large-scale applications. There are so many ways in which phthalocyanine can form 2d-PC. The most popular way is using metallic atoms at the center of the phthalocyanine and, with this, create a tessellation with regular squares formed by the phthalocyanine molecules at each vertex or sharing entire edges. From the computational point of view, the most popular tessellation made is with square sharing edges, 4·4·4·4 types, forming the well-known Archimedean tiling, and variations of this symmetry. From the experimental point of view, there are so many options and variety with so many ligands working as another polygon, and these new tessellations are not well studied and understood so there is so much work to be done. Besides all the possibilities on symmetry, there are other options on tessellations, in which the symmetry could be broken. As it is already observed, motifs with fewer symmetries could be more stable and promote a better electronic transfer.