Servicios Personalizados
Revista
Articulo
Indicadores
-
Citado por SciELO
-
Accesos
Links relacionados
-
Similares en SciELO
Compartir
Revista ALCONPAT
versión On-line ISSN 2007-6835
Rev. ALCONPAT vol.7 no.3 Mérida sep./dic. 2017
https://doi.org/10.21041/ra.v7i3.239
Applied research
Surface treatment with silicon-based nanoparticles induced during curing: Effect on durability of portland cement based materials
1 Universidad Autónoma de Nuevo León, Facultad de Ingeniería Civil, México
The effect of the introduction of silicon-based nanoparticles (NBS) prepared by the sol-gel method was studied. The introduction of NBS was induced for 72 hours during curing by using mortar specimens with a w/c ratio of 0.65 and a suspension prepared at [NBS] = 0.1% with respect to the volume of the curing water. Subsequently, the samples followed a period of immersion in potable water to promote the reaction of NBS inside mortar. Frequent measurements of electrical resistivity were made. Subsequently, a series of specimens were exposed in environments rich in Cl- or CO2. The results indicated a decrease in the penetration of aggressive agents into the mortar specimens. This coincides with increasing resistivity specimens treated with respect to the reference.
Keywords: Durability; surface treatment; nanoparticles; curing; permeability
El efecto de la introducción de nanopartículas base silicio (NBS) preparadas por sol-gel en la permeabilidad de materiales base cemento portland fue estudiado. La introducción de NBS en morteros fabricados con una relación a/c de 0.65 fue inducida durante el curado por 72h, utilizando suspensión de [NBS]=0.1% vs. el volumen del agua. Posteriormente, las muestras siguieron un periodo de inmersión en agua potable con el fin de promover la reacción de la NBS con la matriz cementante y la resistividad fue medida frecuentemente. Después, fueron expuestos en ambientes con Cl- o CO2. Los resultados indicaron una disminución en la penetración de estos agresivos y un incremento de la resistividad en los especímenes tratados; por ende un incremento de la vida útil.
Palabras clave: durabilidad; tratamiento superficial; nanopartículas; curado; permeabilidad
O efeito da introdução de nanopartículas à base de silício (NBS) preparado por sol-gel sobre a permeabilidade dos materiais de base de cimento Portland foi estudado. A introdução de NBS em argamassas feitas com uma relação a / c de 0,65 foi induzida durante a cura por 72 h, usando [NBS] = 0,1% vs. o volume de água. Posteriormente, as amostras seguiram um período de imersão na água potável para promover a reação do NBS com a matriz de cimento e a resistividade foi medida com freqüência. Posteriormente, foram expostos em ambientes com Cl ou CO2. Os resultados indicaram uma diminuição na penetração destes agressivos e um aumento da resistividade nos espécimes tratados; aumentando assim a vida útil.
Palavras-chave: durabilidade; tratamento de superfície; nanopartículas; curado; permeabilidade
1. INTRODUCTION
Problems of durability in reinforced concrete structures (RCS) begin with the interaction of the medium with the surface of the portland cement base materials (Cai et al., 2016; Hou et al., 2015; Hou et al., 2016). In the short to medium term, this interaction causes the deterioration of the RCS, generating further annual expenses between 18 to 21 billion USD in renovations or repairs due to the corrosion of reinforcing steel (Barnat-Hunek et al., 2016; Khaloo et al., 2016). More than 50% of these RCS present deterioration problems because of the high permeability or low quality concrete (Fajardo, et al., 2015; Rtimi et al., 2016), which favors the transport mechanism of aggressive agents as to the carbon dioxide (CO2), chloride ion (Cl-) and sulphate (SO4 -2), these being, the main cause of deterioration in the RCS (Achal et to the., 2015; Trapote-Barreira et al., 2014). However, we must remember that the important properties, such as strength, permeability and durability, are linked directly with the concrete porosity (Fajardo et al., 2015; Hou et al., 2015; Hou et al., 2016; Kupwade-Patil et al., 2016). However, the porosity is determined by the type and the amount of cement used, the level of compaction, transportation, time and type of curing, with the latter being one of the main stages of the construction system of the RCS, since it is of great help and contributes to the achievement of many of their properties, (Fajardo et al., 2015; Zahedi et al., 2015). Curing is the mechanism used to promote hydration of cement; taking control of the temperature and the movement of moisture from the surface to the inside of the concrete. Curing occurs in a period where the RCS usually lost moisture by evaporation, bleeding or hydration. During this period, there is a demand for water to continue with hydration. The gradient of moisture generated promotes the movement of water into the interior, coupled with the absorption originating the porosity of the matrix of concrete. This technique is not new, but allows the hydration, in a way that maximizes the potential properties that can be developed on a cement portland based matrix (Kong et al., 2016; Kupwade-Patil et al., 2016; Wyrzykowski et al., 2016). However, an improper curing process (or absence) of concrete can result in a high porosity, especially on the outer surface of the concrete. Today there are a wide variety of products that claim to offer a series of benefits to the surface of the concrete; from increase in the mechanical properties to permeability reduction. However, these products added during or after the curing process, are not achieving the benefits offered and the needs of the construction industry. Indeed, problems were found associated with improper application, degradation caused by constant exposure to UV rays, chemical incompatibility and therefore loss of adhesion between it and the substrate (Lakshmi et al., 2012; Zhu et al., 2016; Zhu et al., 2013). So, a wide variety of research has been generated over the last decades, mainly due to the economic impact caused by the problem of durability, where a variety of ways to improve the RCS were proposed and that they are more long-lasting. However, the most common strategy adopted, is to slow down the process of degradation of concrete reinforced by the decrease of porosity by reducing the water/cement ratio or the addition of nanoparticles (Efome et al., 2015; Franzoni et al., 2014; Jia et al., 2016; Pacheco-Torgal et al., 2009; Pigino et al., 2012; Pour-Ali et al., 2015). Nanoparticles through migration in hardened mortars have been entered in previous studies and found to be blocking the pores, causing a decrease in permeability (Fajardo et al., 2015; Sanchez et al., 2014). Other methods have been developed since the introduction of nanoparticles into the interior of cement base materials applied at early ages (Hou et al. 2015; Jalal et to the. 2012). These techniques have demonstrated the beneficial effects of the interaction of nanoparticles with certain phases of the matrix of the cementitious materials. However, present difficulties associated mainly to the complexity of the application in situ or real elements. Therefore, this paper explores the influence of the movement of moisture from the curing with water in cement portland base materials making it an enabling way for the induction of nanoparticles with the aim of improving the properties that increase durability.
2. EXPERIMENTAL PROCEDURE
2.1 Materials
Ordinary portland cement (CPO 40), with a chemical composition similar to a type I cement was used for this study and comply with ASTM C150 and NMX-C-414-ONNCCE respectively. It is used as aggregate, standard sand (silica's Ottawa) that conforms to ASTM C 778. In the case of the mixed water, deionized water for the preparation of mortar specimens was used, complies with the norm NMX-C-122-ONNCCE and as well to prevent the intrusion of ions Cl- to the mix.
2.2 Manufacture of specimens
Designed cylindrical specimens of mortar with a diameter of 50 mm and 150 mm in length. Specimens were manufactured with a 0.65 water/cement ratio (w/c) as shown in Table 1, this in order to maintain a characteristic of a conventional concrete porosity. Mixing of mortars was carried out following the procedure described in ASTM C 305 and ASTM C 109 standard. After being cast, specimens were kept at 20 ° C for 24 h as set out in the standard ASTM C171.
2.3 Production of silicon-based nanoparticles (NBS)
To obtain amorphous NBS and sizes ranging from 8 to 50 nm, the sol-gel was utilized at 70 ° C using the procedure described in a previous work (Fajardo et al., 2015).
2.4 Preparation of the specimen and application of curing.
The specimens were demold once met 24 hours since its manufacture. Subsequently, cross sections at the ends were performed (to 25 mm) on each specimen, this to avoid the effects of border produced during the preparation and casting. Then cross-sections were performed to obtain specimens of 50 mm in length, as shown in Figure 1.

Figure 1 Obtaining of the cross sections from the specimen of mortar for the implementation of treatment with NBS.
The application of the solution with NBS took place during the curing process. A solution was prepared using 0.1% of NBS of the volume of water used in curing. Application of the solution was conducted from surface form generating a film of water with a height of 20 mm (see Figure 2), which remained for 3 days. Reference samples (CNT) where drinking water for curing was employed were also used for comparison.
2.5 Characterization of CNT and NBS treated specimens
After the application of treatment with NBS, the specimens were cut for the determination of the electrical resistivity in 3 zones of 16 mm each (see Figure 3). Zone 1 (Z1), being the closest to the area where the curing with NBS was applied, zone 2 (Z2) intermediate treatment, while zone 3 (Z3), is the furthest area to the treated surface. The obtained sections were placed in immersion in water to saturate the specimens. Subsequently, the specimens were monitored constantly during 112 days to determine the effect generated by the NBS.
Physisorption of N2 and electrical resistivity tests were determined in CNT and NBS treated specimens as described in previous research (Fajardo et al., 2015).
2.6 Exposure to aggressive media
Once the electrical resistivity measurements showed a change in the microstructure of mortar specimens (an increase in the electrical resistivity), these were expose to an environment rich in CO2 o Cl- to determine the NBS effect in two different aggressive media.
In the case of CO2 treatment, 3 specimens were taken for the CNT and NBS treated mortars, which were coated in the periphery with a epoxy paint (Epoxaclyl E-6000) and sealer with Alkafin (acrylic sealer, Comex®) to promote a crossfeed of carbonation. Subsequently, the samples were placed in an environmental chamber at 30° C with a relative humidity of 60 ± 5% and a 10% concentration of CO2 for 14 days. At the end, the depth of carbonation was determined using the procedure described in a previous work using phenolphthalein as indicator (Fajardo et al., 2015).
Another series of specimens were placed in water for a period of 56 days in a 165 ± 1 g/l NaCl solution as indicated in the standard ASTM C 1556. In order to determine the concentration of chlorides, 10 g of powder from each zone of the manufacture tablets were obtained. Then, the content of chlorides (total vs % by weight of cement) was obtained in a X-ray fluorescence - Epsilon 3 X. It is noteworthy that for this test 50 mm sections were divided into 3 zones called Z1, Z2 and Z3 approximately 16 mm thickness (see Figure 4b).
3. RESULTS AND DISCUSSION
Figure 5 presents the results obtained from the electrical resistivity in mortar specimens corresponding to different areas of the CNT and NBS specimens. The 10 kOhm.cm electrical resistivity threshold (URE) was set as a dotted horizontal line in the figure. This value is commonly used as an effective parameter to evaluate the risk of corrosion of steel embedded in concrete, particularly when corrosion was induced by aggressive agents (Polder, 2001; Koleva et al., 2008). A concrete matrix that will exceed this threshold goes from a high to low/moderate corrosion risk of reinforcing steel. The time expressed in the figure, represents the immersion time from 1 to 112 days after having been subjected to curing with NBS for 72 hours.
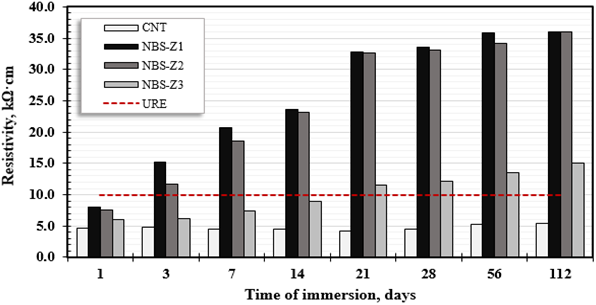
Figure 5 Evolution of the electrical resistivity of the CNT and NBS treated specimens during the immersion time.
In general, there is an ascending behavior during the first 56 days, achieving an increase in resistivity ranging from 34-36 kΩ.cm in the NBS treated specimens, while CNT specimens remained around 5.0 kΩ.cm. It can be observed that after 28 days the NBS treated specimen resistivity increased up to 7 times above the CNT specimens for the case of zones 1 and 2. This increase was superior to that obtained in a previous work where up to 3.5 times was achieved using a more complex system of introduction (Fajardo et al., 2015). Similarly, an increase in the electrical resistivity in the NBS treated specimens from day 3 by placing them above the URE, being most notable for Z1 and Z2 samples with a resistivity ranging between 12-15 kΩ.cm, which infers an increment in the durability from the decrease of the permeability. It is important to mention that during this stage the cementing material still presents hydration reactions, so it can be inferred that the induction of NBS during curing could be more effective than other relatively more complex techniques based on applications of electric fields or void systems in hardened concrete (Fajardo et al., 2015; Kawashima et al., 2013; Kupwade-Patil et al., 2016; Sanchez et al., 2014; Zhu et al., 2016).
Although the mechanisms are not fully defined, some authors infer mechanisms generated by the fast interaction of the NBS with the cementing matrix generating precipitation and subsequent flocculation of the NBS to the contact with the pore solution. So, the NBS crowd and obstruct the interconnections of pores to interact with the Ca(OH)2 generating secondary reactions of hydration and decreasing the permeability of the RCS (Cárdenas et al., 2008; Fajardo et al., 2015).
Figure 6 presents the results of the diameter and pore area obtained by the technique of Physisorption of N2 in zone 1 of the CNT and NBS specimens after 14 days of immersion.
Porosity in the range of 25 - 450 A (2.5 to 45 nm), is observed with a bimodal behavior; emphasizing the area of mesopores (< 10 nm). The specimens treated with NBS decrease the permeability, by blocking a large number of small capillary pores, exposing the porosity > 90 A (9 nm). Closing the entry mainly to interconnected pores and pores of the gel. This, due to the interaction of the NBS during the reactions of hydration reducing 90% of the adsorption of N2 in the specimens treated with NBS regarding CNT specimens (Cai et al 2016; Zhang et al., 2011).
Figure 7 shows the isotherms of adsorption of nitrogen in CNT and NBS treated specimens, showing the presence of isotherms of type IV (according to IUPAC) concerning the characteristics of mesoporous and macroporous materials of bottle or irregular neck type.
In general, there is a reduction in the volume of adsorption nitrogen around 90% in samples treated with NBS during the first 14 days of immersion with respect to sample CNT, indicating a reduction in pore diameters and therefore reduction permeability. Therefore, it is confirmed that, under the experimental conditions, using NBS in aqueous solution during curing promotes the entry of NBS reduce small capillary pores in accordance with the results obtained by Hou, these being responsible of the permeability in portland cement base materials (Cai et al 2016;) Hou et to the. 2013, Hou et al., 2015).
In Figure 8 are shown the results of mortar specimens that were exposed in an environment rich in CO2 with the objective of evaluating their effect. There is a clear decrease in the depth of carbonation on mortar specimens treated with NBS regarding the CNT sample. It is therefore possible to conclude that the effect generated in the mortars by the NBS treatment is due to a decrease in permeability due to obstruction of bottleneck pore type. The obstruction causes a decrease of the interconductivity and therefore an increase in the electrical resistivity of the cementing matrix.
Table 2 shown the results of mortar specimens exposed in an environment rich in Cl-. The results confirm a decrease in the concentration of chlorides, which represent a decrease in the diffusion of Cl- ions through the binder matrix in the specimens treated with NBS and consequent decrease of transport of aggressive agents through the mortar. Therefore, the decrease in the concentration of chlorides evidence a decrease in permeability, which is consistent with the results presented above.
Table 2 Concentration of chlorides in the CNT and NBS treated specimens.
Zones | Total Cl - (% by weight of cement) | |
CNT | NBS | |
1 | 17.23 | 0.09 |
2 | 12.85 | 0.06 |
3 | 10.72 | 0.07 |
The increase of the service life of newly placed RCS could be obtained when applying a treatment as described here. The curing process using NBS could be considered a new option to increase the durability during the construction process, which is the main problems of the loss of durability.
4. CONCLUSIONS
The NBS application induced during outer curing through a solution, according to the experimental conditions used here, leads to the conclusion that:
• The transport of moisture during the curing process favors the entry of the NBS towards the interior of the matrix binder.
• The resistivity of mortar treated with NBS increased up to 7 times above the CNT specimens from their first 21 days of immersion in water.
• The decrease in the adsorption of N2 was attributed to the reduction of interconnection of the porosity and therefore reducing the permeability, thus avoiding the introduction of ions Cl- & CO2.
The NBS application during the curing process, may be promising in the increase of the durability in the RCS.
5. ACKNOWLEDGEMENT
The authors would like to acknowledge PAICYT-UANL IT435-15. Dulce Cruz-Moreno would like to acknowledge CONACYT for the grant No. 414932 for her doctoral training.
REFERENCES
Achal, V., Mukherjee, A. (2015), A review of microbial precipitation for sustainable construction. Construction and Building Materials, 93, 1224-1235. [ Links ]
Barnat-Hunek, D., Smarzewski, P., Suchorab, Z. (2016), Effect of hydrophobisation on durability related properties of ceramic brick. Construction and Building Materials, 111, 275-285. [ Links ]
Cai, Y., Hou, P., Duan, C., Zhang, R., Zhou, Z., Cheng, X., Shah, S. (2016), The use of tetraethyl orthosilicate silane (TEOS) for surface-treatment of hardened cement-based materials: A comparison study with normal treatment agents. Construction and Building Materials, 117, 144-151. [ Links ]
Cárdenas H., Struble, L. (2008), “Modeling electrokinetic nanoparticle penetration for permeability reduction of hardened cement paste”. ASCE J Mater Civ Eng ; 20(11):683-691. [ Links ]
Du, H., Du, S., Liu, X. (2015), Effect of nano-silica on the mechanical and transport properties of lightweight concrete. Construction and Building Materials, 82, 114-122. [ Links ]
Efome, J. E., Baghbanzadeh, M., Rana, D., Matsuura, T., Lan, C. Q. (2015), Effects of superhydrophobic SiO2 nanoparticles on the performance of PVDF flat sheet membranes for vacuum membrane distillation. Desalination, 373, 47-57. [ Links ]
Fajardo, G., Cruz-López, A., Cruz-Moreno, D., Valdez, P., Torres, G., Zanella, R. (2015), Innovative application of silicon nanoparticles (SN): Improvement of the barrier effect in hardened Portland cement-based materials. Construction and Building Materials, 76, 158-167. [ Links ]
Franzoni, E., Varum, H., Natali, M. E., Bignozzi, M. C., Melo, J., Rocha, L., Pereira, E. (2014), Improvement of historic reinforced concrete/mortars by impregnation and electrochemical methods. Cement and Concrete Composites, 49, 50-58. [ Links ]
Hou, P., Cheng, X., Qian, J., Zhang, R., Cao, W., Shah, S. P. (2015), Characteristics of surface-treatment of nano-SiO 2 on the transport properties of hardened cement pastes with different water-to-cement ratios. Cement and Concrete Composites, 55, 26-33. [ Links ]
Hou, P., Kawashima, S., Kong, D., Corr, D. J., Qian, J., Shah, S. P. (2013), Modification effects of colloidal nanoSiO 2 on cement hydration and its gel property. Composites Part B: Engineering, 45(1), 440-448. [ Links ]
Hou, P., Zhang, R., Cai, Y., Cheng, X., Shah, S. P. (2016), In situ Ca(OH)2 consumption of TEOS on the surface of hardened cement-based materials and its improving effects on the Ca-leaching and sulfate-attack resistivity. Construction and Building Materials, 113, 890-896. [ Links ]
Jalal, M., Mansouri, E., Sharifipour, M., Pouladkhan, A. R. (2012), Mechanical, rheological, durability and microstructural properties of high performance self-compacting concrete containing SiO2 micro and nanoparticles. Materials and Design, 34, 389-400. [ Links ]
Ji, T. (2005), Preliminary study on the water permeability and microstructure of concrete incorporating nano-SiO2. Cement and Concrete Research, 35(10), 1943-1947. [ Links ]
Jia, L., Shi, C., Pan, X., Zhang, J., Wu, L. (2016), Effects of inorganic surface treatment on water permeability of cement-based materials. Cement and Concrete Composites, 67, 85-92. [ Links ]
Kawashima, S., Hou, P., Corr, D. J., Shah, S. P. (2013), Modification of cement-based materials with nanoparticles. Cement and Concrete Composites, 36(1), 8-15. [ Links ]
Khaloo, A., Mobini, M. H., Hosseini, P. (2016), Influence of different types of nano-SiO 2 particles on properties of high-performance concrete. Construction and Building Materials, 113, 188-201. [ Links ]
Koleva, D. A., Copuroglu O., Breugel K., van Ye G., de Wit J. H. W. (2008), Electrical resistivity and microstructural properties of concrete materials in conditions of current flow. Cement Concr Compos 30:731-44. [ Links ]
Kong, Y., Wang, P., Liu, S., Gao, Z. (2016), Hydration and microstructure of cement-based materials under microwave curing. Construction and Building Materials, 114, 831-838. [ Links ]
Kupwade-patil, K., Al-aibani, A. F., Abdulsalam, M. F., Mao, C., Bumajdad, A., Palkovic, S. D., & Büyüköztürk, O. (2016), Microstructure of cement paste with natural pozzolanic volcanic ash and Portland cement at different stages of curing. Construction & Building Materials, 113, 423-441. [ Links ]
Lakshmi, R. V., Bharathidasan, T., Bera, P., Basu, B. J. (2012), Fabrication of superhydrophobic and oleophobic sol-gel nanocomposite coating. Surface and Coatings Technology, 206(19-20), 3888-3894. [ Links ]
Pacheco-Torgal, F., Jalali, S. (2009), Sulphuric acid resistance of plain, polymer modified, and fly ash cement concretes. Construction and Building Materials, 23(12), 3485-3491. [ Links ]
Pigino, B., Leemann, A., Franzoni, E., Lura, P. (2012), Ethyl silicate for surface treatment of concrete - Part II: Characteristics and performance. Cement and Concrete Composites, 34(3), 313-321. [ Links ]
Polder, R. B. (2001). Test methods for on site measurement of resistivity of concrete - a RILEM TC-154 technical recommendation. Construction and Building Materials, 15(2-3), 125-131. [ Links ]
Pour-Ali, S., Dehghanian, C., Kosari, A. (2015), Corrosion protection of the reinforcing steels in chloride-laden concrete environment through epoxy/polyaniline-camphorsulfonate nanocomposite coating. Corrosion Science, 90, 239-247. [ Links ]
Rtimi, S., Pulgarin, C., Sanjines, R., Kiwi, J. (2016), Accelerated self-cleaning by Cu promoted semiconductor binary-oxides under low intensity sunlight irradiation. Applied Catalysis B: Environmental, 180, 648-655. [ Links ]
Sánchez, M., Alonso, M. C., González, R. (2014), Preliminary attempt of hardened mortar sealing by colloidal nanosilica migration. Construction and Building Materials, 66, 306-312. [ Links ]
Trapote-Barreira, A., Cama, J., Soler, J. M. (2014), Dissolution kinetics of C-S-H gel: Flow-through experiments. Physics and Chemistry of the Earth, 70-71, 17-31. [ Links ]
Wyrzykowski, M., Ghourchian, S., Sinthupinyo, S., Chitvoranund, N., Chintana, T., Lura, P. (2016), Internal curing of high performance mortars with bottom ash. Cement and Concrete Composites, 71, 1-9. [ Links ]
Zahedi, M., Ramezanianpour, A. A., Ramezanianpour, A. M. (2015), Evaluation of the mechanical properties and durability of cement mortars containing nanosilica and rice husk ash under chloride ion penetration. Construction and Building Materials, 78, 354-361. [ Links ]
Zhang, M. H., Li, H. (2011), Pore structure and chloride permeability of concrete containing nano-particles for pavement. Construction and Building Materials. [ Links ]
Zhu, L. J., Zhu, L. P., Zhang, P.-B., Zhu, B. K., Xu, Y.-Y. (2016), Surface zwitterionicalization of poly(vinylidene fluoride) membranes from the entrapped reactive core-shell silica nanoparticles. Journal of Colloid and Interface Science, 468, 110-119. [ Links ]
Zhu, Y. G., Kou, S. C., Poon, C. S., Dai, J. G., Li, Q. Y. (2013), Influence of silane-based water repellent on the durability properties of recycled aggregate concrete. Cement and Concrete Composites, 35(1), 32-38. [ Links ]
Received: August 02, 2017; Accepted: September 18, 2017